Abstract
Objective
To evaluate the degree of conversion (DC%), salt yield and mechanical properties of self-adhesive luting agents (SAA) set under dual-cure (E) and self-cure (NE) modes.
Methods
Three SAA (GC LinkAce/GCLA, MaxCem Elite/MXEL, Rely-X Unicem 2/RXUN) and an adhesive resin luting agent (Rely-X Ultimate/RXUL-control) were used. The properties tested under E and NE modes were a) DC% and phosphate salt yield after 10 min, 1 h (h) and 3 weeks (w) storage, by infrared spectroscopy; and b) the mechanical properties of 3 w-stored specimens by instrumented indentation testing (Martens hardness/HM, Elastic modulus/EIT, Elastic index/ηIT) and microscopic Vickers hardness/VH. Statistical analysis was performed by 3-way ANOVA (DC%), 2-way ANOVA (salt yield) and 1-way ANOVA (mechanical properties) at an a = 0.05.
Results
Significantly higher DC% was found in E, except from the 3 w groups of GCLA and MXEL. Within E, no significant differences were found, but within NE, there were differences in the 3 w groups of GCLA (vs 10 min) and MXEL (vs 1 h). All materials demonstrated increased salt yield in NE, with the highest values found in RXUL and RXUN. GCLA, RXUL showed the lowest HM in E and MXEL the highest in NE. The rankings of the significant differences in EIT were MXEL > GCLA,RXUN,RXUL (E) and RXUL,MXEL > GCLA,RXUN (NE), whereas for ηIT RXUL,RXUN > GCLA,MXEL (E) and GCLA > RXUL > MXEL,RXUN (NE). The results of VH measurements showed an overestimation ranging from 13% up to 38% in comparison with HM.
Significance
There are significant differences in the properties tested, which may anticipate variations in the chemical, mechanical and biological performance of the products.
1
Introduction
The developments in all-ceramic and resin composite indirect restorations have made luting procedures more demanding, aiming to integrate the restorations with hard dental tissues for enhanced strength, retention, marginal sealing and aesthetics. Today, resin luting agents, especially the particle reinforced resin composite materials, are considered as the materials of choice, since they demonstrate superior mechanical properties , stability and reduced solubility , high adhesive strength to restorative materials and tooth structure, and improved aesthetics .
Resin composite luting agents have been classified into three groups depending on the adhesive system used for tooth pre-treatment . Originally, 3-step adhesive systems (etchant, primer, bonding resin) had been introduced, which had been then substituted for two-step, etch & rinse (etchant, adhesive resin) and later on for two-step, self-etch (acidic adhesive primer, adhesive or bonding resin) systems, ranking the composite luting agents, accordingly. Moreover, based on the monomer chemistry and curing mechanism of the main luting agent, they were further classified as non-adhesive or adhesive and light-, self- or dual-cured. The complexity arising from these multistep application procedures, established the need for simplification of the luting protocols, to reduce chairside time, complexity and technique sensitivity . The result was the design of self-adhesive composite luting agents (SAA), not requiring any means of tooth pre-treatment. The first product of this category launched in 2002 (Rely-X Unicem, 3M ESPE) as a dual-cured SAA.
The simple and user friendly luting protocol of this new agent was soon adopted by most dental materials manufacturers; a great number of SAA have been introduced in the market with many variations in composition. The main components of SAA can be classified as follows: (a) Aromatic and aliphatic dimethacrylate monomers, to form a crosslinked network, (b) acidic methacrylate monomers to adhere with enamel and dentin and copolymerize with the crosslinking monomers, (c) glass filler particles or basic compounds, to neutralize residual acidic monomers, (d) conventional silanated filler particles to provide strength by an inert reinforcing effect, (e) appropriate catalysts and stabilizers to comply with the dual-cure nature and shelf-life requirements of the materials and (f) pigments and opacifiers, to match the aesthetic requirements. Most of the products contain sources of fluoride release, as well (fluoroaluminosilicate glass fillers, YbF 3 , etc.) .
The curing capacity of these materials has been investigated in several studies. Although it has been confirmed that self-curing leads to lower degree of C C conversion in the absence of light-curing , there is a great variation in the values obtained by various experimental designs and analytical techniques (FTIR, Raman). Moreover, despite the fact that acidic monomers are included in these materials, their neutralization capacity has been evaluated in few studies by surface pH measurements only , without any further information on the acid–base reaction involved. The mechanical properties of the SAA have been assessed, so far, by conventional methods (3-point flexural strength and modulus on bar-shaped specimens , biaxial flexural strength , hardness and wear ) and were found to be similar or inferior to composite resin luting agents, provided that the SAA specimens had been light-cured, for most of the products tested.
Recently, new materials have been introduced, with improved properties and handling characteristics. The aim of the study was to comparatively evaluate the curing efficiency, examine the salt formation capacity and evaluate the mechanical properties of SAA. The null hypothesis was that there are no statistically significant differences among the products in the properties tested.
2
Materials and methods
The products used in the study are listed in Table 1 . GCLA, MXEL and RXUN are SAA, whereas RXUL is a new adhesive luting agent to be used on tooth surfaces previously treated with a self-etching universal adhesive. This product, with monomer and filler composition close to RXUN, was used as reference.
Product shade/lot/code | Composition a | Manufacturer |
---|---|---|
GC LinkAce A2 shade, Lot:1211069-76 (Code: GCLA) | Resin: DUDMA, GDMA, MDP | GC Corporation, Tokyo, Japan |
Catalysts: CHP, 2- tert -butyl-4,6-dimethylphenol | ||
Filler: silanated glass (50–70 wt%) | ||
MaxCem Elite Yellow shade, Lot: 49818224 (Code: MXEL) | Resin: HDDMA, GDMA, DUDMA, GPDMA | Kerr Italia Srl, Scafati, Italy |
Catalysts: TMBHP, CQ, stabilizer | ||
Filler: FAlSiO 4 glass, SiO 2 , Ba-glass,YF 3 (67 wt.%) | ||
RelyX Ultimate A1 shade, Lot:494545 (Code: RXUL) | Monomers: DCDMA, TEGDMA, substituted-DMA, GPDMA and GPDMA isomer | 3M Deutschland GmbH, Neuss, Germany |
Catalysts: sodium persulphate, sodium toluene p -sulphinate, TBPIN, 1-benzyl,5-phenyl barbituric acid-Ca salt | ||
Filler: silanated glass-powder, silanated SiO 2 , oxide glass, Ca(OH) 2 , TiO 2 (61–71 wt%) | ||
RelyX Unicem 2 A2 shade, Lot: 495505 (Code: RXUN) | Monomers: TEGDMA, substituted-DMA, DCDMA, GDMA-P and GDMA isomer-P adducts | 3M Deutschland GmbH, Neuss, Germany |
Catalysts: sodium persulphate, sodium toluene p -sulphinate, TBPIN, cupric acetate monohydrate, 1-benzyl,5-phenyl barbituric acid-Ca salt, [(3-methoxypropyl)imino]di-2,1-ethanediyl bismethacrylate | ||
Filler: silanated glass-powder, silanated SiO 2 , oxide glass chemicals, Ca(OH) 2 , TiO 2 (55–72 wt%) |
a According to the safety data sheet (sds) files of the manufacturers.
Two groups of specimens (E: exposed, for light-activated/dual-cured and NE: non-exposed for self-cured) were prepared from each product (n = 18/group), as follows: a transparent blue matrix strip (Blue Striproll, KerrHawe SA, Bioggio, Switzerland) was placed on a microscopic glass-slide and two 100-μm thick glass cover-slips were placed each side leaving a central area of 10 mm length free. A material aliquot was placed directly from the mixing tip of the luting agent at the center of the strip, a second strip was placed onto the material and finally covered by another glass cover-slip. The material diameter (∼7 mm) was adjusted to be smaller than the tip of the light-curing device (10 mm), to mediate uniform light exposure. The specimens of group NE were not irradiated, whereas the specimens of group E were irradiated by a LED curing unit (G2 Bluephase, Ivoclar-Vivadent, Schaan, Liechtenstein) for 10 s under the standard irradiation mode (1200 mW/cm 2 light intensity), as tested with a curing radiometer (Bluephase meter, Ivoclar-Vivadent), with the tip of the fiber-optic in contact with the top cover-slip. All the 100 μm-thick specimens produced were stored in dark and dry conditions (37 °C/40% RH), covered with the strips and slips to avoid oxygen inhibition. The specimens of each product were randomly classified in 3 subgroups (n = 6/subgroup) to be tested after 10 min, 1 hour (h) and 3 weeks (w) storage at the conditions described before.
- a)
Degree of conversion and phosphate salt formation
The degree of the C C bonds conversion (DC%) and the capacity of phosphate salt formation (salt yield) at the specific time intervals were evaluated by micro-attenuated total reflection FTIR spectrometry (m-ATR FTIR) on the directly irradiated (E) and the top (NE) specimen surfaces using an ATR accessory (Golden Gate, Specac, Kent, UK) equipped with a diamond internal reflection element (2 mm × 2 mm sampling area, single-reflection, ∼2.5 μm depth of analysis at 1000 cm −1 ) and ZnSe lenses, attached to an FTIR spectrometer (Spectrum GX, PerkinElmer, Bacon, UK). Spectra were acquired from the center of each specimen, pressed against the diamond crystal by the sapphire anvil of the accessory, under the following conditions: 4000–650 cm −1 wavenumber range, 4 cm −1 resolution, 30 scans co-addition, ATR and baseline corrections.
For DC measurements, the C C vibrations at 1635 cm −1 were chosen as analytical bands in all groups, whereas the aromatic C⋯C vibrations at 1605 cm −1 (RXUL, RXUN), the N H vibrations at 1545 cm −1 (GCLA) and the C O vibrations at 1715 cm −1 (MXEL) were used as reference bands, respectively. To calculate the DC%, the net peak absorbance heights were measured at each band and the analytical to reference band ratios of each group were normalized relative to the corresponding control values (unset paste measured immediately after mixing) according to the equation: DC% = 100 × [1 − (Ap C = C × Am RF /Am C = C × Ap RF )], where A is the net peak absorbance heights of set (p) and unset (m) materials at the analytical (C C) and reference bands (RF: C⋯C, N H or C O), respectively.
For assessment of the capacity of phosphate salt formation, the region 1250–850 cm −1 was selected, where appear the peaks of P O ( v2 at 1250 cm −1 ), P O C, (1150, 940 cm −1 ) and PO 3 2− ( v3, v1 at 1030–960 cm −1 ) vibrations. Since at the same region there are absorption peaks of the CH OH (1185–1160 cm −1 ) and C O C (1145–1050 cm −1 ) groups of the resin backbone, and the Si O groups (1106, 1078 cm −1 ) of the filler particles, subtraction techniques were employed.
Expanded spectra (2000–750 cm −1 region) of the E and NE groups were used. Intense bands were identified for major components; the C O at 1715 cm −1 for resin and the complex peak of 1250–850 cm −1 , which incorporates the 1030–960 cm −1 region assigned to phosphate salts. Spectra were multiplied by a scaling factor and the resin component of set materials was subtracted (D = NE − E). Then, a scale expansion (factor 0.9–1) was used to enhance the remaining bands and identify the difference bands. Phosphate salt formation was determined by measuring the differential peak area at the region 1030–960 cm −1 , which was normalized against the corresponding differences of the individual reference bands used for DC% measurements. This normalization was performed to compensate for any changes in the intensity of the C O during the post-curing period. The spectra of 10 min were subtracted for the corresponding spectra of 1 h and 3 w to evaluate the role of storage period within E and NE groups, and then the spectra of the same period were subtracted (NE − E) to evaluate the role of the setting mechanism (self-curing vs dual-curing).
- b)
Mechanical properties
To evaluate the mechanical performance of the materials under the storage conditions and irradiation modes described above, instrumental indentation testing (IIT) was employed. Disk-shape specimens were prepared from each material using teflon molds (10 mm diameter and 2 mm thickness). Each mold was placed on a microscopic glass-slide covered with a transparent blue matrix strip, the luting agent was then dispensed into the mold, a second glass-slide covered with a strip was pressed onto the mold to remove material excess and clamped with holders to apply a constant pressure. Three groups of specimens (n = 12/group) were prepared for each material. Half the specimens (n = 6/group) were light-cured as before (E), whereas the rest were non-exposed (NE). The first group was stored in dark and dry conditions at 37 °C for 10 min, the second for 1 h and the third for 3 w. IIT measurements were carried out employing a universal hardness-testing machine (ZHU 0.2/Z2.5, Zwick Roell, Ulm, Germany). For each specimen force-indentation depth curves were monitored applying a Vickers indenter under 9.8 N load with 15 s dwell time. Three readings were taken from the directly irradiated surfaces of E and the top surfaces of NE subgroups, and the mean value was used as representative of the specimen. From the force-indentation depth curves recorded, the following IIT parameters were determined according to the ISO 14577-1 specification : The Martens hardness (HM), the indentation modulus (E IT ), and the percentage of the elastic part of indentation work (η ΙΤ ), also known as elastic index. Finally, the Vickers hardness (HV1) was measured based on the diagonal of the indentation, as imaged under a 20× objective lens with a 17× field of view magnifier. All indents were made at the center of the specimen surfaces and they were located at a distance of more than 2.5× the indentation diameter from the specimen edges according to the ASTM E384 guidelines .
- c)
Statistical analysis
The results of DC% were analyzed by descriptive statistics, 3-way ANOVA (Balanced Design) and All Pairwise Multiple Comparison Procedures (Holm–Sidak method). Salt formation derived from difference (NE − E) spectra were evaluated within each material group as a function of the storage time by 1-way ANOVA and Tukey post-hoc tests, whereas 2-way ANOVA and Tukey tests were used for assessment of the spectral differences per time interval (3 w–10 min and 1 h–10 min) between NE and E modes per material. For HM, HV1, E IT and η ΙΤ , 1-way ANOVA and Tukey tests were used. The correlation between the differences in DC% and salt yield obtained from difference spectra (NE − E) for the storage period per material was investigated by Pearsons correlation coefficient. All tests were performed at a 95% confidence level (α = 0.05), using the SigmaStat v 3.1 software (Jandel Scientific Software, St. Raphael, CA, USA).
2
Materials and methods
The products used in the study are listed in Table 1 . GCLA, MXEL and RXUN are SAA, whereas RXUL is a new adhesive luting agent to be used on tooth surfaces previously treated with a self-etching universal adhesive. This product, with monomer and filler composition close to RXUN, was used as reference.
Product shade/lot/code | Composition a | Manufacturer |
---|---|---|
GC LinkAce A2 shade, Lot:1211069-76 (Code: GCLA) | Resin: DUDMA, GDMA, MDP | GC Corporation, Tokyo, Japan |
Catalysts: CHP, 2- tert -butyl-4,6-dimethylphenol | ||
Filler: silanated glass (50–70 wt%) | ||
MaxCem Elite Yellow shade, Lot: 49818224 (Code: MXEL) | Resin: HDDMA, GDMA, DUDMA, GPDMA | Kerr Italia Srl, Scafati, Italy |
Catalysts: TMBHP, CQ, stabilizer | ||
Filler: FAlSiO 4 glass, SiO 2 , Ba-glass,YF 3 (67 wt.%) | ||
RelyX Ultimate A1 shade, Lot:494545 (Code: RXUL) | Monomers: DCDMA, TEGDMA, substituted-DMA, GPDMA and GPDMA isomer | 3M Deutschland GmbH, Neuss, Germany |
Catalysts: sodium persulphate, sodium toluene p -sulphinate, TBPIN, 1-benzyl,5-phenyl barbituric acid-Ca salt | ||
Filler: silanated glass-powder, silanated SiO 2 , oxide glass, Ca(OH) 2 , TiO 2 (61–71 wt%) | ||
RelyX Unicem 2 A2 shade, Lot: 495505 (Code: RXUN) | Monomers: TEGDMA, substituted-DMA, DCDMA, GDMA-P and GDMA isomer-P adducts | 3M Deutschland GmbH, Neuss, Germany |
Catalysts: sodium persulphate, sodium toluene p -sulphinate, TBPIN, cupric acetate monohydrate, 1-benzyl,5-phenyl barbituric acid-Ca salt, [(3-methoxypropyl)imino]di-2,1-ethanediyl bismethacrylate | ||
Filler: silanated glass-powder, silanated SiO 2 , oxide glass chemicals, Ca(OH) 2 , TiO 2 (55–72 wt%) |
a According to the safety data sheet (sds) files of the manufacturers.
Two groups of specimens (E: exposed, for light-activated/dual-cured and NE: non-exposed for self-cured) were prepared from each product (n = 18/group), as follows: a transparent blue matrix strip (Blue Striproll, KerrHawe SA, Bioggio, Switzerland) was placed on a microscopic glass-slide and two 100-μm thick glass cover-slips were placed each side leaving a central area of 10 mm length free. A material aliquot was placed directly from the mixing tip of the luting agent at the center of the strip, a second strip was placed onto the material and finally covered by another glass cover-slip. The material diameter (∼7 mm) was adjusted to be smaller than the tip of the light-curing device (10 mm), to mediate uniform light exposure. The specimens of group NE were not irradiated, whereas the specimens of group E were irradiated by a LED curing unit (G2 Bluephase, Ivoclar-Vivadent, Schaan, Liechtenstein) for 10 s under the standard irradiation mode (1200 mW/cm 2 light intensity), as tested with a curing radiometer (Bluephase meter, Ivoclar-Vivadent), with the tip of the fiber-optic in contact with the top cover-slip. All the 100 μm-thick specimens produced were stored in dark and dry conditions (37 °C/40% RH), covered with the strips and slips to avoid oxygen inhibition. The specimens of each product were randomly classified in 3 subgroups (n = 6/subgroup) to be tested after 10 min, 1 hour (h) and 3 weeks (w) storage at the conditions described before.
- a)
Degree of conversion and phosphate salt formation
The degree of the C C bonds conversion (DC%) and the capacity of phosphate salt formation (salt yield) at the specific time intervals were evaluated by micro-attenuated total reflection FTIR spectrometry (m-ATR FTIR) on the directly irradiated (E) and the top (NE) specimen surfaces using an ATR accessory (Golden Gate, Specac, Kent, UK) equipped with a diamond internal reflection element (2 mm × 2 mm sampling area, single-reflection, ∼2.5 μm depth of analysis at 1000 cm −1 ) and ZnSe lenses, attached to an FTIR spectrometer (Spectrum GX, PerkinElmer, Bacon, UK). Spectra were acquired from the center of each specimen, pressed against the diamond crystal by the sapphire anvil of the accessory, under the following conditions: 4000–650 cm −1 wavenumber range, 4 cm −1 resolution, 30 scans co-addition, ATR and baseline corrections.
For DC measurements, the C C vibrations at 1635 cm −1 were chosen as analytical bands in all groups, whereas the aromatic C⋯C vibrations at 1605 cm −1 (RXUL, RXUN), the N H vibrations at 1545 cm −1 (GCLA) and the C O vibrations at 1715 cm −1 (MXEL) were used as reference bands, respectively. To calculate the DC%, the net peak absorbance heights were measured at each band and the analytical to reference band ratios of each group were normalized relative to the corresponding control values (unset paste measured immediately after mixing) according to the equation: DC% = 100 × [1 − (Ap C = C × Am RF /Am C = C × Ap RF )], where A is the net peak absorbance heights of set (p) and unset (m) materials at the analytical (C C) and reference bands (RF: C⋯C, N H or C O), respectively.
For assessment of the capacity of phosphate salt formation, the region 1250–850 cm −1 was selected, where appear the peaks of P O ( v2 at 1250 cm −1 ), P O C, (1150, 940 cm −1 ) and PO 3 2− ( v3, v1 at 1030–960 cm −1 ) vibrations. Since at the same region there are absorption peaks of the CH OH (1185–1160 cm −1 ) and C O C (1145–1050 cm −1 ) groups of the resin backbone, and the Si O groups (1106, 1078 cm −1 ) of the filler particles, subtraction techniques were employed.
Expanded spectra (2000–750 cm −1 region) of the E and NE groups were used. Intense bands were identified for major components; the C O at 1715 cm −1 for resin and the complex peak of 1250–850 cm −1 , which incorporates the 1030–960 cm −1 region assigned to phosphate salts. Spectra were multiplied by a scaling factor and the resin component of set materials was subtracted (D = NE − E). Then, a scale expansion (factor 0.9–1) was used to enhance the remaining bands and identify the difference bands. Phosphate salt formation was determined by measuring the differential peak area at the region 1030–960 cm −1 , which was normalized against the corresponding differences of the individual reference bands used for DC% measurements. This normalization was performed to compensate for any changes in the intensity of the C O during the post-curing period. The spectra of 10 min were subtracted for the corresponding spectra of 1 h and 3 w to evaluate the role of storage period within E and NE groups, and then the spectra of the same period were subtracted (NE − E) to evaluate the role of the setting mechanism (self-curing vs dual-curing).
- b)
Mechanical properties
To evaluate the mechanical performance of the materials under the storage conditions and irradiation modes described above, instrumental indentation testing (IIT) was employed. Disk-shape specimens were prepared from each material using teflon molds (10 mm diameter and 2 mm thickness). Each mold was placed on a microscopic glass-slide covered with a transparent blue matrix strip, the luting agent was then dispensed into the mold, a second glass-slide covered with a strip was pressed onto the mold to remove material excess and clamped with holders to apply a constant pressure. Three groups of specimens (n = 12/group) were prepared for each material. Half the specimens (n = 6/group) were light-cured as before (E), whereas the rest were non-exposed (NE). The first group was stored in dark and dry conditions at 37 °C for 10 min, the second for 1 h and the third for 3 w. IIT measurements were carried out employing a universal hardness-testing machine (ZHU 0.2/Z2.5, Zwick Roell, Ulm, Germany). For each specimen force-indentation depth curves were monitored applying a Vickers indenter under 9.8 N load with 15 s dwell time. Three readings were taken from the directly irradiated surfaces of E and the top surfaces of NE subgroups, and the mean value was used as representative of the specimen. From the force-indentation depth curves recorded, the following IIT parameters were determined according to the ISO 14577-1 specification : The Martens hardness (HM), the indentation modulus (E IT ), and the percentage of the elastic part of indentation work (η ΙΤ ), also known as elastic index. Finally, the Vickers hardness (HV1) was measured based on the diagonal of the indentation, as imaged under a 20× objective lens with a 17× field of view magnifier. All indents were made at the center of the specimen surfaces and they were located at a distance of more than 2.5× the indentation diameter from the specimen edges according to the ASTM E384 guidelines .
- c)
Statistical analysis
The results of DC% were analyzed by descriptive statistics, 3-way ANOVA (Balanced Design) and All Pairwise Multiple Comparison Procedures (Holm–Sidak method). Salt formation derived from difference (NE − E) spectra were evaluated within each material group as a function of the storage time by 1-way ANOVA and Tukey post-hoc tests, whereas 2-way ANOVA and Tukey tests were used for assessment of the spectral differences per time interval (3 w–10 min and 1 h–10 min) between NE and E modes per material. For HM, HV1, E IT and η ΙΤ , 1-way ANOVA and Tukey tests were used. The correlation between the differences in DC% and salt yield obtained from difference spectra (NE − E) for the storage period per material was investigated by Pearsons correlation coefficient. All tests were performed at a 95% confidence level (α = 0.05), using the SigmaStat v 3.1 software (Jandel Scientific Software, St. Raphael, CA, USA).
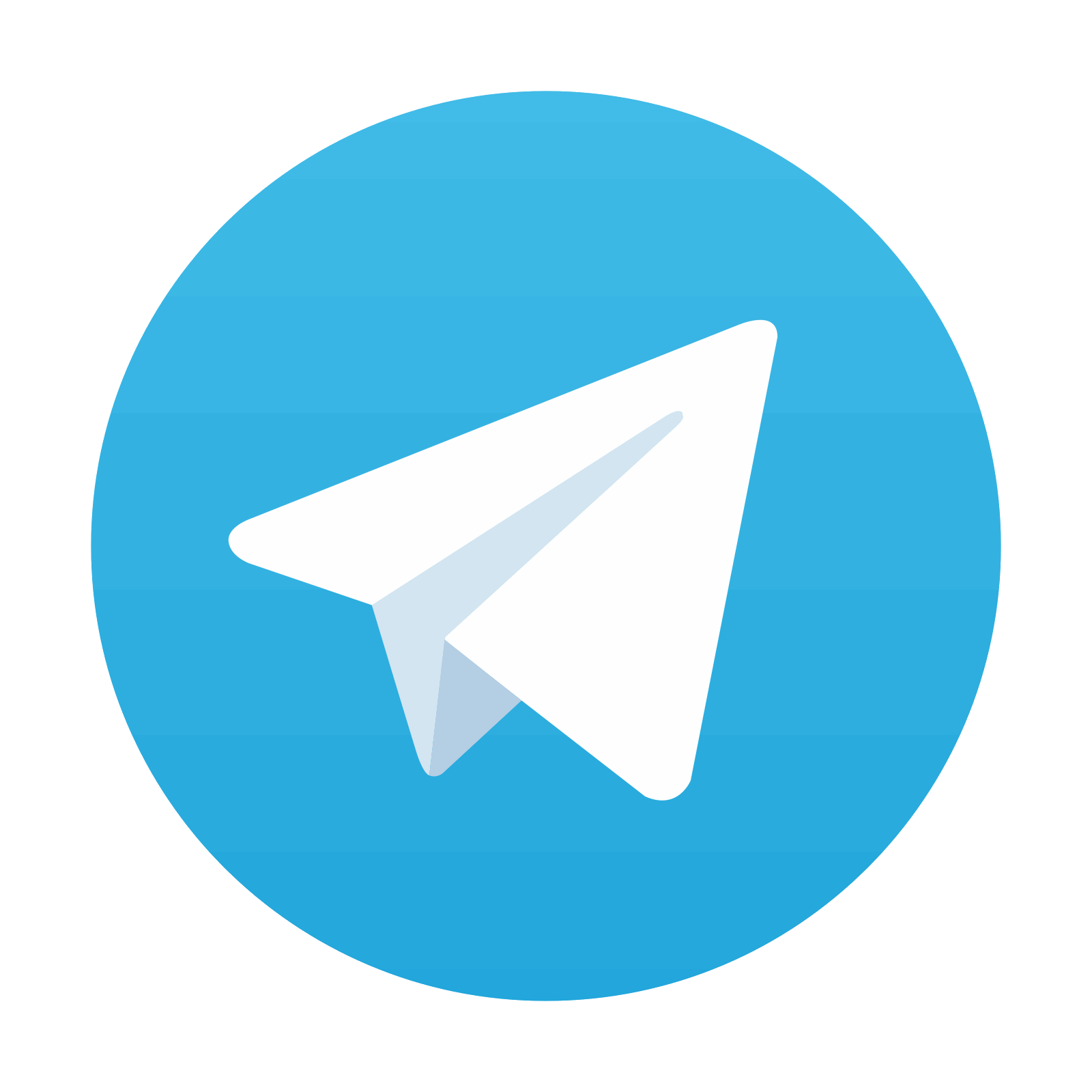
Stay updated, free dental videos. Join our Telegram channel

VIDEdental - Online dental courses
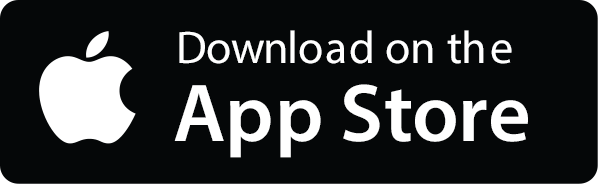
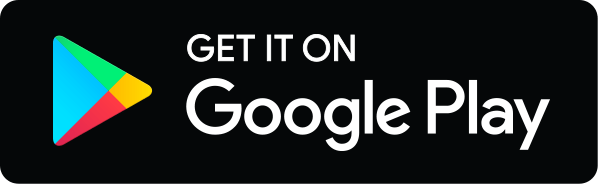