Abstract
Objective
The present study tested the central hypothesis that selective demineralisation of dentine extrafibrillar minerals by lowering the phosphoric acid concentration improves the quality of the resin-dentine interface.
Methods
Dentine surfaces were etched with different concentrations of phosphoric acid (1, 5, 10, 20, 30 or 40 wt%). Scanning electron microscopy was used to observe the micromorphology of the etched dentine surfaces. Energy dispersive X-ray analysis was performed to determine the residual Ca-content of the demineralised dentine matrix. Atomic force microscopy-based nanoindentation was used to analyse the nanomechanical properties of the treated dentine surfaces. The influence of H 3 PO 4 concentration on resin-dentine bond strength was evaluated by microtensile bond strength testing. One-way ANOVA was used to compare the residual Ca-content ratio, reduced elastic modulus (Er) of the treated dentine surfaces and microtensile bond strength among groups.
Results
Collagen fibrils appeared to be wider in diameter after etching with 5% and 10% H 3 PO 4 . The partially-demineralized collagen scaffold retained part of its rigidity to maintain an uncollapsed three-dimensional structure. Etching with 1% H 3 PO 4 resulted in the highest residual Ca-content ratio and Er of demineralised dentine matrix, followed by 5% H 3 PO 4 . Those values were all significantly higher than values derived from the other groups. Etching with 30% H 3 PO 4 resulted in the lowest Ca-content ratio and Er. Using 5% H 3 PO 4 as etchant resulted in the highest resin-dentine bond strength.
Conclusions
Selective demineralisation of the dentine matrix may be achieved by lowering the H 3 PO 4 concentration to 5 wt%, to achieve better bonding performance.
Clinical relevance
By retaining intrafibrillar minerals, more through air-drying of the partially demineralised collagen matrix may be accomplished without the need to worry about collapsing a mineral-free collagen matrix during air-drying. This may result in the elimination of water-wet bonding during the application of etch-and-rinse adhesives.
1
Introduction
Dentine adhesives are functionally classified as etch-and-rinse adhesives or self-etch adhesives. Although both classes of adhesives can achieve high immediate bond strength, many studies showed that the high immediate bond strength achieved with the etch-and-rinse technique are not durable . Improvement of resin-dentine bond durability remains a timely issue even after more than 60 years of intensive research on dentine bonding.
Lack of resin-dentine bond durability in etch-and-rinse adhesives has been attributed to the involvement of water during the formation of hybrid layer . With the advent of the water-wet bonding concept in 1992, resin-dentine bond strengths achieved with the use of etch-and-rinse adhesives dramatically increased from 10 MPa to more than 40 MPa . Nevertheless, this bonding concept also introduced undesirable consequences. After acid etching, the apatite crystallites in the mineralised dentine matrix are almost completely dissolved by the 30–40% phosphoric acid to a depth of 5–8 μm. Prior to the application of resin monomers, it is critical to keep the microporosities patent within the demineralised collagen matrix. When water is evaporated from the demineralised collagen matrix, the relatively flexible collagen fibrils rapidly form interpeptide hydrogen bonds with their nearest neighbours, causing the matrix to collapse and form an impermeable membrane-like structure that prevents optimal resin monomer infiltration . Water has the highest Hansen’s solubility parameter for hydrogen bonding (δ h ; 40.4 (J/cm 2 ) ½ ), which can easily break the hydrogen bonds between collagen fibrils to provide room for the infiltration of resin monomers . Because dimethacrylates such as triethylene glycol dimethacrylate are almost insoluble in water, hydrophilic resin monomers such as 2-hydroyethyl methacrylate (HEMA) are introduced into dentine adhesives as a co-solvent, and to enhance the dispersion of hydrophobic resin monomers within the wet demineralised dentine substrate. This noble objective, however, is accomplished at the expense of creating potentially permeable, unstable resin matrices that are prone to water sorption, leaching of resinous components and hydrolysis over time .
Bonding of contemporary self-etch adhesives is also based on the water-wet bonding concept since these adhesives contain 20–35% water . Self-etch adhesive formulations usually include hydrophilic monomers with acidic functional groups, water, HEMA, and bifunctional dimethacrylates. Water is indispensable for ionisation of the acidic resin monomers in self-etch adhesives. The process releases hydronium ions that etch through the smear layer to partially demineralise the underlying dentine. After comonomer infiltration, the volatile solvents and water are partially removed by air-drying. As a water-miscible co-solvent, HEMA decreases the vapour pressure of water and interferes with water evaporation . Prior to polymerisation, HEMA may form a homogenous hydrogel containing unbranched polymer and create water channels (i.e. water trees) within the polymerised resin matrix . After polymerisation, the residual water, incompletely polymerised HEMA, and acidic monomers that are retained within the polymer network can absorb water, resulting in the plasticisation of the polymers and decreases in the physical properties of the polymerised adhesive .
Contemporary etch-and-rinse adhesives, self-etch adhesives as well as universal adhesives cannot eliminate the adverse effect of water and do not produce perfect hybrid layers. As long as the dentine is completely demineralised, it is difficult for resin monomers to completely infiltrate the interfibrillar and intrafibrillar spaces within the demineralised collagen matrix. Whereas the interfibrillar spaces (i.e. spaces around the collagen fibrils) are comparatively easier to be infiltrated by resin monomers, infiltration of the intrafibrillar spaces (i.e. spaces around the microfibrils and between collagen triple helices with a collagen fibril) is a formidable task even for the most hydrophilic monomers with the smallest molecular dimensions. It is surmised that if mineralised collagen fibrils can be selectively demineralised to remove only the interfibrillar minerals while keeping the intrafibrillar minerals intact, there will be no need for resin monomers to infiltrate the nanoscopical intermolecular spaces within the collagen fibrils. An additional advantage is that the interfibrillarly-demineralised collagen fibrils retain their strength because of the presence of intrafibrillar minerals and will not collapse during the process of air-drying.
The simplest way to selectively demineralise dentine with the etch-and-rinse technique is to lower the acidity of the etchant. Hence, the objective of the present study was to examine the central hypothesis that selective demineralisation of dentine extrafibrillar minerals by lowering the phosphoric acid concentration improves the quality of the resin-dentine interface. This objective was accomplished by testing three null hypotheses: 1) there is no difference in dentine surface morphology after the application of different concentrations of phosphoric acid; 2) there are no differences in the Ca 2+ content and modulus of elasticity of dentine surfaces after they are etched with different concentrations of phosphoric acid; and 3) there are no differences in the tensile bond strengths of resin-dentine bonds prepared from etching dentine with low (below 10%) or high concentrations (10–40%) of phosphoric acid.
2
Materials and methods
2.1
Teeth collection
Unerupted, non-carious third molars were collected from adult patients with their informed consent, under a protocol approved by the Peking University School of Stomatology Institutional Review Board (PKUSSIRB-201522043). The teeth were stored at 4 °C in saline containing 0.02% sodium azide to prevent bacterial growth and used within 1 month after collection.
2.2
Preparation of etchants
The etchants employed for etching dentine surfaces consisted of phosphoric acid solutions with different concentrations (1, 5, 10, 20, 30 or 40 wt%). They were prepared from 85 wt% phosphoric acid (Millipore Sigma, St. Louis, MO, USA) by dilution with deionised water. The pH values of these etchants are shown in Table 1 .
Groups | pH value | Ca-content ratio (%) * N = 5 teeth |
Reduced elastic modulus (GPa) * N = 10 points |
---|---|---|---|
Control | – | – | 19.65 ± 2.43 a |
1% | 1.48 | 54.71 ± 2.58 A | 2.04 ± 0.29 b |
5% | 1.01 | 42.49 ± 2.49 B | 1.00 ± 0.22 c |
10% | 0.82 | 32.93 ± 0.71 C | 0.40 ± 0.10 d |
20% | 0.55 | 28.82 ± 3.35 C | 0.32 ± 0.08 d |
30% | 0.35 | 17.38 ± 3.68 D | 0.19 ± 0.05 e |
40% | 0.46 | 31.99 ± 1.47 C | 0.36 ± 0.10 d |
* Values are means ± standard deviations. For each parameter, values identified by different superscripts letters are significantly different (P < 0.05).
2.3
Scanning electron microscopy
The occlusal enamel of 18 teeth was removed with a water-cooled low-speed Isomet saw (Buehler Ltd., Lake Bluff, IL, USA) under copious water cooling. Sectioning was performed perpendicular to the tooth axis. A second parallel cut was conducted at 1 mm below the dentinoenamel junction to expose the mid-coronal dentine. Eighteen dentine discs (700 ± 100 μm thick) were obtained and randomly divided into 6 groups. A stereomicroscope (SMZ 1500, Nikon, Japan) was used to ensure that the dentine discs were free of enamel. The occlusal side of the dentine discs were polished with 320-, 800-, 1200-, 2400- and 4000-grit wet silicon carbide papers under running water for 20 s each. Each occlusal dentine surface was then etched with a different concentration of phosphoric acid for 15 s. The treated dentine surfaces were rinsed with running water for 30 s. Immediately after rinsing, the dentine discs were dehydrated in an ascending series of ethanol (25%, 50%, 75%, 95% and 100%). Changing of ethanol was performed without allowing the demineralised dentine surface to be exposed to air, that would have resulted in the collapse of the demineralised collagen matrix by surface tension. After drying with a critical point dryer (EM CPD300, Leica, Germany) for 4 h, the dentine discs were mounted on aluminum stubs and sputter-coated with gold. The specimens were observed using a field emission scanning electron microscope (Helios Nanolab 600i, FEI, Hillsboro, OR, USA) at 5 kV.
2.4
Energy dispersive X-ray analysis
The residual calcium content on the surface of the phosphoric acid-treated dentine discs was analysed using an energy dispersive X-ray micro-analyser (EDX). The dentine discs were polished using the same method as described previously. Prior to acid etching, the dentine discs were sectioned into two halves. One-half was etched with a respective concentration of phosphoric acid. The other half served as the control and was not etched. The dentine surfaces were analysed with scanning electron microscopy (SU8020, HITACHI, Japan) EDX (X-Max M, Oxford Instrument, Wiesbaden, Germany). Ten points were randomly selected on each dentine disc and analysed using an accelerating voltage of 10 kV and a counting time of at least 3 min for each point. The residual Ca-content on each etched dentine surface was expressed as a percentage of the content derived from the control half of the same tooth. Measurements were conducted on five teeth for each group to obtain the mean measurement for that group .
2.5
Nanoidentation with atomic force microscopy
Seven 5 mm-long, 1.5 mm-wide and 1.0 mm-thick dentine beams were obtained from one mid-coronal dentine disc that had been polished using the previously described method. Six dentine beams were randomly selected and etched with different concentrations of phosphoric acid. The remaining dentine beam served as the control group and was not etched. After etching, the nanomechanical properties of the treated dentine surfaces were evaluated with a pyramidal Berkovich diamond indenter (Hysitron Inc., Minneapolis, MN, USA; tip radius of curvature 150 nm) attached to an atomic force microscope (Nanoscope III Digital Instruments, Santa Barbara, CA, USA). Ten points from the intertubular dentine of each treated dentine surface were randomly selected and tested.
Because dentine possesses viscoelastic and viscoplastic time-dependent behaviour, the demineralised collagen matrix may be subjected to adhesive interaction with the Berkovich tip. Accordingly, a multi-cycling method was used in the present study to eliminate thermal drift/creep in order to obtain stable and reliable readings. Each specimen was partially unloaded to 10% of the maximum load at every cycle and then reloaded to the next peak using the same constant loading/unloading rate (400 μN/s). The load ranged from 100 μN to 1000 μN with an incremental load of 100 μN per cycle. Loads and displacements were recorded during the loading and unloading cycles. The reduced elastic modulus (Er) of the exposed dentine matrix, being an elastic-plastic material, was calculated using the following equation:
Er = Π 2 β s A
where β is the correction factor for the indenter shape (for a Berkovich indenter, β ≈ 1.05), A is the contact area at the point of unloading, and S is the slope of the unloading curve .
2.6
Microtensile bond strength testing
Twenty-four molars were allocated randomly to six groups according to the phosphoric acid concentration. After exposing the mid-coronal dentine surface, a standardised smear layer was created by grinding with 600-grit silicon carbide paper under running water for 60 s. Each dentine surfaces was etched with a different concentration of phosphoric acid for 15 s, and then rinsed with water spray for 30 s. The dentine surface was blot-dried with moist tissue paper (Kimberly-Clark, Roswell, GA, USA) to keep the demineralised collagen matrix visibly moist, in accordance with the water-wet bonding technique. Two separate layers of an ethanol-based two-step etch-and-rinse adhesive (Single Bond Plus, 3 M ESPE St. Paul, MN, USA) was applied on the moist dentine surfaces. This was followed by evaporation of the adhesive solvent for 15 s and light-curing of the adhesive for 20 s at 800 mW/cm 2 using a visible light-curing unit (Elipar FreeLight 2, 3 M ESPE). Resin composite build-up was subsequently performed using three 1.5 mm increments of a hybrid resin composite (Clearfil AP-X, Kuraray Noritake Dental Inc., Osaka, Japan). Each increment was individually light-cured for 20 s.
The bonded teeth were stored in water at 37 °C for 24 h. Each bonded tooth was sectioned in the x and y directions across the adhesive interface to obtain 16 resin-dentine beams, each with cross-sectional dimensions of approximately 0.7 × 0.7 mm. The exact dimensions were measured with a pair of digital callipers. Each beam was individually fixed to a custom-made testing jig with cyanoacrylate adhesive (Zapit, Dental Ventures of America Inc., Corona, CA, USA) and subjected to tensile loading using a universal testing machine (EZ-L-1kN, Shimadzu, Japan) at a crosshead speed of 1.0 mm/min until failure.
2.7
Statistical analysis
The residual Ca-content ratios, reduced elastic modulus of the treated dentine surfaces and microtensile bond strengths were separately analyzed using one-way analysis of variance, after ascertaining the normality and homogeneity of variance assumptions of the respective data sets. Microtensile bond strengths obtained from beams derived from each of the 4 teeth of each group (6 groups) were pooled together to obtain the mean bond strength value, with each tooth treated as a statistical unit. For each parameter, post-hoc pairwise comparisons were performed using the Student-Newman-Keuls statistic. When either of the aforementioned statistical assumptions was violated, the data set was non-linearly transformed to satisfy those assumptions prior to the use of parametric statistical methods. For each analysis, statistical significance was pre-set at α = 0.05.
2
Materials and methods
2.1
Teeth collection
Unerupted, non-carious third molars were collected from adult patients with their informed consent, under a protocol approved by the Peking University School of Stomatology Institutional Review Board (PKUSSIRB-201522043). The teeth were stored at 4 °C in saline containing 0.02% sodium azide to prevent bacterial growth and used within 1 month after collection.
2.2
Preparation of etchants
The etchants employed for etching dentine surfaces consisted of phosphoric acid solutions with different concentrations (1, 5, 10, 20, 30 or 40 wt%). They were prepared from 85 wt% phosphoric acid (Millipore Sigma, St. Louis, MO, USA) by dilution with deionised water. The pH values of these etchants are shown in Table 1 .
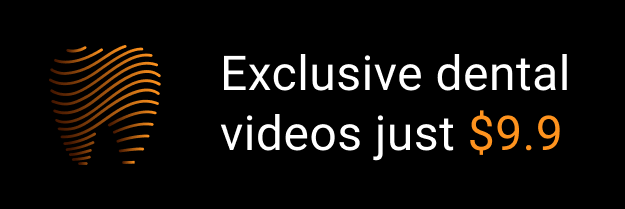