Key points
- •
Late and secondary orbital reconstructions are more demanding than primary reconstructions due to altered anatomic landmarks and late soft tissue changes—so the ultimate objective should be an adequate primary reconstruction.
- •
Computer-assisted planning/surgery in combination with intraoperative navigation and/or imaging improve postoperative results.
- •
Most common indications for secondary orbital reconstructions are globe malposition, binocular diplopia, and impaired eye motility.
Introduction: nature of the problem
Secondary orbital reconstructions mostly affect defects in the area of the orbital floor or the medial wall, because these structures are most susceptible to fractures due to fragility. These defects may cause an increase of the orbital volume and a dislocation of the orbital content; furthermore, an altered globe position may arise ( Fig. 1 ). Clinically, enophthalmos and/or hypoglobus develops ( Figs. 2 and 3 ). As part of primary trauma diagnostics, swelling of the soft tissue in the affected eye socket may compensate this increase in volume for a short time. Even supposedly objective methods such as Hertel or Naugle ophthalmometry are not helpful because the bony structures are not sufficiently taken into account in those cases. In cases where a volume-relevant defect is not treated surgically and swelling has subsided, the bulb can be dislocated permanently and associated complaints may arise. In addition to an aesthetically noticeable asymmetry, functional restrictions are particularly stressful for the patients. These restrictions range from binocular diplopia to motility restrictions and may have a significant impact on everyday quality of life. Such symptoms may be more apparent in insufficiently reconstructed orbits than in fractured orbits with no treatment at all ( Fig. 4 ). This finding is due to more pronounced scarring in previously operated orbits on one hand, on the other hand to foreign material, that has to be removed during secondary orbital reconstruction, and this may complicate the further outcome. The ultimate goal of any reconstruction, primary or secondary, must therefore be to restore the original aesthetic and functional situation as good as possible by means of accurate preoperative diagnostics and planning.




Surgical technique
Preoperative planning
A thorough patient history including the initial trauma and all previous surgeries, as well as physical examination has to be conducted with special focus on visual acuity, pupil function, eye motility, and binocular diplopia. Even though major eye injuries are seldom, an interdisciplinary examination by an ophthalmologist is recommended also for medicolegal reasons. The position of the bulb is assessed using a Naugle or Hertel ophthalmometry and photo documentation. Current indications for secondary orbital corrections are enophthalmos larger than 2 mm and persistent diplopia due to possible tissue entrapment. Optimal timing for the procedure depends on the specific case, but 3 to 6 months after primary reconstruction or trauma is favored by most surgeons. For preoperative planning a three-dimensional (3D) imaging for evaluation of the bony boundary and the orbital soft tissue situation is mandatory. Computed tomography with contrast medium and a layer thickness of less than 1 mm is recommended. Because of the lack of soft tissue resolution, cone-beam computed tomography (CBCT) alone often does not provide sufficient information. The same applies to MRI for the bony boundary. The 3D imaging and the clinical findings facilitate a precise planning of the further procedure.
A recent 3D data set can therefore be used as the basis for further planning. The planning is basically identical to that of a primary orbital reconstruction. Assuming the symmetry of the midface, the orbital shape of the unaffected side is used. Using a mirroring function of the planning software, this object serves as a template for the virtual reconstruction of the fractured orbit. In primary reconstruction cases, a simple mirroring is usually sufficient. The requirements for patient-specific implants are also similar to those of primary cases: round edges, radiologic visibility, inert material, and reconstruction of significant anatomic areas (ie, posterior ledge, transition zone). The bony frame may be reconstructed in the same shape as before the trauma with the help of determining the orbital volumes based on the bony boundaries, which works well in primary orbital trauma repair. In the case of secondary reconstructions, additional overcorrections of the orbital volume may be necessary due to posttraumatic or postsurgical fat tissue atrophy or increase of the bony orbit (eg, additional roof fracture or loss of lateral wall). The amount of overcorrection has to be estimated individually for each patient and fracture pattern, hence up to now no conclusive literature is available on this matter ( Fig. 5 ). The same applies to imported foreign material. The scope of such an additional virtual volume correction may unfortunately only be determined objectively to a limited extent by volume measurement and still requires a solid experience of the operating surgeon. Ultimately, the goal of preoperative planning is the construction of a virtual model of the orbit, which matches the clinical situation. This virtual model is then the basis for 3D-printing patient-specific biomodels or, ideally, manufacturing patient-specific implants. Many surgeons prefer titanium as standard material due to its biological and technical characteristics (ie, inert, dimensionally stable, radiopacity). The preoperative virtual planning may also be used intraoperatively for real-time navigation or intraoperative 3D imaging with subsequent superimposition as a quality control.

Preparation and patient positioning
When using intraoperative navigation, only hard tissue–based registration is thought to be sufficiently exact for intraorbital navigation. A dental splint with navigational markers (eg, titanium mini screws) may be fabricated preoperatively through a dental technician. With the help of a preoperative 3D data set and the prepared splint, an exact hard tissue registration is possible without further invasive measures. Using this workflow, the most accurate radiation-free position control may be performed. Real-time intraoperative navigation is still an extremely helpful tool, especially for complex reconstructions or less-experienced surgeons.
The patient is placed supine during the procedure and intubated orally in most cases. In cases of intraoperative navigation both the oral cavity and scalp part of the head need to be disinfected in order to place the registration splint and transfixed navigation parts.
The use of a headlamp or an alternative light source tailored to the surgeon is strongly recommended.
Surgical approach
The transconjunctival retroseptal approach seems most suitable, as seldom complications occur. Incision may be done using either a scalpel or a monopolar device. If there are no contraindications, the monopolar offers the advantage of bloodless preparation in layers. In general, the best position for the surgeon is cranially to the patient giving appropriate view for most orbital areas.
Surgical procedure
The lower eyelid is held caudally using lid retractors; the bulb together with the intraorbital soft tissue is gently pulled cranially. Now the conjunctival tissue is incised. It is important to keep a sufficiently large distance from the lid edge, and an arcuate cut should be performed ( Fig. 6 ). Now the subconjunctival layer is also dissected using the monopolar needle ( Fig. 7 ). The intraorbital tissue is carefully pulled up. The eyelid retractors may now be replaced by smaller ones. The periosteum can now be identified easily and incised as well in the area of the infraorbital margin ( Fig. 8 ). Now the orbital floor or the lower parts of the medial wall may be dissected easily ( Fig. 9 ). In cases with large defects in the area of the medial wall, a transcaruncular extension of the transconjunctival approach may be necessary. This way, the entire floor and the medial orbital wall may be reached in a safe and reliable manner. After preparation of the affected region of the orbit and removal of any previously inserted foreign materials, the reconstruction is carried out. A patient-specific titanium implant allows the orbital volume to be reconstructed in a dimensionally stable manner in accordance with the preoperative planning. Intraoperative navigation offers a radiation-free and real-time technique for quality control. For this purpose, a transfixed navigation star is attached to the scalp and the 3D position of the patient in the room is registered by means of the aforementioned dental splint. This way, a continuous comparison with the virtual planning may already take place during the reconstruction process ( Fig. 10 ). The correct position is first checked clinically. If this seems reasonable, fixation is carried out with at least one osteosynthesis screw in the area of the orbital margin ( Fig. 11 ). The ultimate objective control, however, is the intraoperative 3D imaging (ie, CBCT) ( Fig. 12 ). In addition to direct evaluation of the images, superimposition with the preoperative planning allows for accurate intraoperative quality control ( Figs. 13 and 14 ). With intraoperative imaging corrections may be carried out directly during the same surgery. This way, any secondary intervention is avoided. After satisfactory reconstruction, passive eye motility is checked with the forced duction test. A visual control of the implant borders ensures that no tissue entrapment exists. After rinsing with ringer lactate, the wound is closed using Vicryl 7-0 ( Figs. 15–17 ).
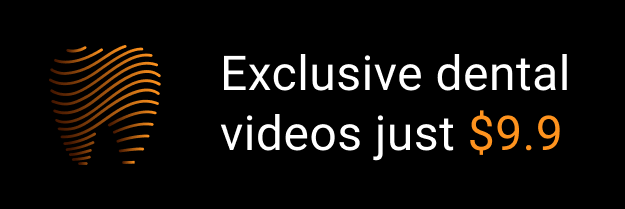