13
Rotary and Reciprocating Motions During Canal Preparation
Ove A. Peters and Ana Arias
Summary
The last decade has seen major changes in the way engine-driven root canal preparation is performed. The introduction of the reciprocation movement has increased clinical efficacy by reducing the number of instrument fractures. Moreover, changes in production technology, for example post-milling heat treatment, have drastically increased fatigue resistance. Some concerns have emerged, such as the potential of dentinal microcrack generation or coronal overpreparation when greater taper instruments are used in reciprocation or rotation. These issues along with several key mechanical properties are assessed by a variety of established testing paradigms in laboratory studies. Based on these test results, usage parameters have been defined. On the other hand, clinically, there is a scarcity of reports that describe outcomes with the current generation of instruments; most initial reports suggest that superior laboratory data may translate to improved clinical performance.
13.1 Goals and Limitations of Engine-driven Root Canal Preparation
Best clinical practice suggests that root canal preparation should result in a tapered and smooth flowing shape that promotes efficient disinfection and an appropriate canal filling; care should be taken to preserve the original anatomy of the root canal system and the strength of the root [1]. In 1974, Herbert Schilder described the desired shape of a properly prepared root canal when he stated, ‘A well-shaped root canal should maintain the original anatomy, the position of the apical foramen, keep the foramen as small as practical and present a continuous tapered preparation from the apical to the coronal third in all the planes to provide a linear resistance form for a proper obturation’ [2]. The technology developed since that time has made the task of root canal shaping much more predictable (Figure 13.1), and with time, the concept has evolved.

Figure 13.1 Examples of root canal preparations for different canal configurations; treatments performed with the principles detailed in this chapter and with rotary root canal instruments.
Both rotary and reciprocating shaping instruments with multiple designs and improved alloys have been developed for a more predictable and safer root canal preparation. The ideal final shape should be designed by clinicians to determine the best instruments and strategies to conform to the current treatment standards. To date, two major concerns remain: the adequate final apical size and the best overall shape of a prepared root canal.
There is no convincing data to suggest a universally appropriate size of apical preparation for the optimum disinfection of a root canal system. Some studies have suggested that larger apical canal diameters allowed deeper irrigant penetration and hence, possibly a decrease in remaining microbial contamination [3–5]; however, others have reported that smaller sizes can accomplish the task just as adequately as larger diameters [6, 7]. Importantly, no apical preparation technique has fulfilled the requirement to render an infected root canal system and its apical extent entirely free of microbiota after instrumentation procedures [8, 9].
The best overall shape of a prepared root canal in the era of so-called minimally invasive dentistry suggests a conscientious removal of dentine in order to maintain the original anatomy of the root canal and the strength of the tooth. Schilder’s preparation goal of a continuously tapered preparation has frequently led to comparatively large coronal canal diameters that may reduce radicular wall dimensions specifically in the coronal third of curved roots [10]; this in turn may reduce outcomes as the amount of dentine removed may relate to difficulties restoring the tooth and also to increased susceptibility to fracture [11].
The aim of root canal treatment has been described as the treatment or prevention of apical periodontitis [12]. However, research continues to emerge that demonstrates issues such as nonrestorable caries, periodontal problems, and importantly, vertical root fracture as major reasons for the loss of root filled teeth [13]. The consideration of patient-centred outcomes has resulted in a focus on retention in function as a relevant endpoint of root canal treatment [14], in addition to and as an extension of the traditional focus on apical periodontitis. Still, a certain amount of space for irrigation and for an adequate canal filling is required. Therefore, the best overall shape should combine the optimal preparation of the apical third for a successful treatment outcome and the maintenance of coronal dentine for long-term functional tooth retention.
13.2 Current Instrument Designs, Movements, and Manufacturing Methods
Since Walia [15] introduced the use of nickel-titanium (NiTi) alloys for endodontic instruments in 1988, there has been a significant evolution of NiTi instruments in order to provide better mechanical characteristic such as flexibility [16], efficiency, and cutting ability [17]. New designs, alloys, manufacturing techniques, heat treatments, and motions have been introduced by manufacturers over time (see examples in Figure 13.2).

Figure 13.2 Example of different NiTi instruments shown, from top to bottom, as photographs, scanning electron micrographs (SEMs) of their cross-section and with differential scanning calorimetry (DSCs) diagrams showing their crystal configuration. (a) ProTaper Universal, (b) ProTaper Next, (c) WaveOne Gold, and (d) TruShape, all by Dentsply Sirona, Ballaigues, Switzerland.
The evolution to date can be described in different phases. Early instrument improvements were mostly the result of design changes in micro-milled instruments made from standard NiTi wire. In this first phase, manufacturers modified characteristics such as cross-sections, tip designs, and uniform/progressive tapers over the length of the cutting blades to improve the properties of rotary instruments.
In a second period, new kinematics were adopted. Reciprocating motion had been used for several decades for root canal preparation with stainless steel files [18], while NiTi instruments were typically rotated clockwise at speeds of 250 to 350 rpm. A case series published by Yared [19] demonstrated that reciprocation with unequal angles of rotation might be used successfully with a ProTaper F2 instrument (Dentsply Sirona, Ballaigues, Switzerland). This new technique for root canal preparation using a single instrument was reported to reduce working time, cost, instrument fracture, and cross-contamination. Thereafter, manufacturers developed two specific instruments to shape root canals using this concept: Reciproc (VDW, Munich, Germany) and WaveOne (Dentsply Sirona). Three major changes were combined at the same time with the development of these files: improved alloy, different motion, and new concepts of use. The instruments were manufactured from M-Wire, a NiTi alloy that is heat-treated before milling and was proven to increase cyclic fatigue (CF) resistance to almost four-fold [20]. Both instruments were actioned with a motion loosely based on the balanced force technique [21], which is considered the most effective hand instrumentation technique [8, 22]. It consists of a series of clockwise and counterclockwise movements with graded angles of rotation.
For engine-driven instruments, the angles of rotation were determined based on stress-strain curves to ensure that file tips would under most clinical conditions experience only safe torque levels. Commercialized motors have rotation patterns of alternating rotations of approximately 150–170º and 30–50º [23]; the larger angle of rotation is in the direction of the flutes. This is actually the opposite to the balanced force technique where the initial engagement is done with a smaller angle of rotation. Importantly, commercialized reciprocation files have left-leaning flutes, so the initial engaging rotation is counterclockwise. Clinically. the new concept of use responded to a ‘single-use, single-file system to shape the root canal completely from start to finish’ [24].
The third phase of development was marked by the appearance of proprietary thermal treatments after the milling process that intended to further enhance the mechanical properties of endodontic instruments. Observations had indicated that subjecting the already milled file to a series of heating and annealing steps drastically changed the physical properties [25]. Specifically, higher flexibility and hence better fatigue resistance of files manufactured with heat-treated alloys have been thoroughly reported.
The earlier introduction of M-Wire (Sportswire LLC, Langley, OK, USA) and R-phase processes (SybronEndo, Orange, CA, USA) had already suggested that heating/annealing of NiTi wires was associated with better mechanical properties. In the mid-2010s, the market saw the development of so-called Blue and Gold alloys (Dentsply Sirona), named after the blue/gold-coloured surface layer that results from the oxidation that the proprietary heating and cooling processes induced on the surface of the instruments [26–28].
The basis for the observed changes is the ability of NiTi alloy to have several different crystal conformations, notably martensitic and austenitic crystal lattices [16], with the transition of the two conformations determined by ambient temperature and applied strain. Heat treatment serves to modify the specific temperatures that describe the conformational changes. Martensite exists at comparatively low temperature and is inherently more flexible compared to austenite. First generation NiTi instruments were in the austenitic conformation both at room and body temperature (note that clinical shaping occurs at about 35ºC).
Heat-treated root canal shaping instruments with a sizeable martensitic component have demonstrated an improvement in mechanical properties [26, 27, 29]. Both rotary and reciprocating instruments are benefiting from these new martensitic alloys. In fact, differential calorimetry analysis (DSC) is becoming a popular tool to understand the phase transformation behaviour of an alloy during temperature change. This technology is used to assess transformation temperatures for phase changes (and therefore property changes) in room temperature compared to body temperature.
Another important aspect in addition to details of any heat treatment is the production process. Most currently available instruments are manufactured using micro-grinding methods; however, this technology introduces grinding marks that may develop into micro-fractures during clinical use. For this reason, in addition to the raw material, manufacturing methods have also evolved. The first instrument not manufactured by grinding was the so-called Twisted File (Kerr, Brea, CA, USA) launched in 2008. The twisting manufacturing process at controlled temperatures appears to avoid surface defects [30, 31]. Further manufacturing methods are the so-called shape setting and electro discharge machining (EDM). Shape-setting allows an instrument to be created based on a micro-milled wired blank to a three-dimensional shape. For example, TruShape (Dentsply Sirona, York, PA, USA) conforming instruments [32, 33] are submitted to a shape setting heat treatment to generate their characteristic S shape. HyFlex EDM (Coltene, Cuyahoga Falls, OH, USA) is manufactured from controlled memory (CM) NiTi material via a thermal process that generates local vaporization of metal. EDM may be described as a noncontact thermal erosion process of machining electrically conductive materials by using precisely controlled sparks between an electrode and a workpiece in the presence of a dielectric fluid. Each spark removes material from both the electrode and workpiece by being heated until material vaporizes. The main difference with other machining methods is the absence of physical contact for material removal; therefore, although the electrode is considered the cutting tool, EDM has no tool force [34].
Advancement in manufacturing techniques promoted a new strategy for the shaping of root canals that are not round in cross-section, and are in fact the majority according to anatomy data [35–38]. The first instrument to permit the so-called conforming shaping was the Self-Adjusting File (SAF, Redent Nova, Ra’anana, Israel). The subsequent generation of files, the so-called 3D-conforming files (XP-endo Shaper, FKG; La Chaux-de-Fonds, Switzerland; TruShape, Dentsply Sirona, see Figure 13.2), is not only supposed to be used for nonround cross-sections, but also with the goal of preserving more dentine with less detriment to the root structure. With this same concept in mind, TruNatomy has been developed with a strong offset cross-section and a smaller wire blank to combine conforming, shaping, and dentine preservation. So far, beyond the use of heat-treated Blue and Gold alloy, no reciprocating instrument has been developed with these new manufacturing methods and conservative three-dimensional concepts.
13.3 Clinical Recommendations for Rotary and Reciprocating Canal Preparation
The technique and sequence described in this chapter (Figure 13.3) addresses the goals of preserving as much dentine as possible promoting functional tooth retention, while providing enough space for irrigation and canal filling. At the same time, steps should be included to avoid root canal preparation mishaps. The reader is referred to endodontic textbooks or monographs [1] for a more detailed description, but a brief summary of these steps is included here.

Figure 13.3 Suggested instrumentation sequence for engine-driven root canal instrumentation depicting the steps of scouting, orifice modification, working length determination/patency, glide path preparation, full shaping, and apical gauging. Note the dashed line illustrating patency length and the solid lines indicative of root canal thirds.
13.3.1 Preparation for Treatment
A thorough individual analysis of the tooth to be treated (based on well-angled preoperative radiographs and small field-of-view cone beam computed tomography scans if such information is needed) must be reviewed prior to treatment to alert to difficulties that if mishandled would create procedural mishaps.
After dental dam placement, access cavity preparation is the first invasive step of a root canal treatment; this step plays a crucial role because all subsequent treatment steps may be compromised if the access cavity is not adequate. A tooth-specific approach to access cavity design is recommended to avoid sacrificing more tissue than necessary, in search of a convenient form with a restricted dimension that allows the precise location of the canal orifices on the pulpal floor. Such conservative approach may be facilitated by the combination of the operating microscope (magnification and illumination together) and specifically designed ultrasonic tips [39, 40] in order to avoid further unnecessary structural weakening. At the same time, a balance has to be struck between tissue preservation and the need to understand the location of all canal orifices as well as to perform efficient cleaning and shaping procedures.
Once the tooth is accessed, the clinician should scout the root canal. The coronal portion of the root canal should be explored with small hand files to assess the depth that the instrument may advance without force. In cases of moderate difficulty, it may be possible to advance a small hand file (size 10 or smaller) with gentle watch-winding motions directly to the root canal terminus and estimate the working length with an electronic apex locator. In more complicated cases, it is advisable to not negotiate the full canal length at this stage. Removal of restrictive coronal dentine, after scouting the root canal with a small file, will make negotiation procedures easier. The purpose of scouting is not the negotiation of the canal to its full length, but to assess the initial direction and canal dimension before the placement of any engine-driven instrument to prevent ledges that will make the step of negotiation more difficult to accomplish.
13.3.2 Early Coronal Modification
The more difficult the canal to access, the more beneficial it is to modify the coronal portion of the root canal prior to any deep entry into the apical third. Early coronal flaring (also known as orifice modification) improves access to the canals by removing coronal interferences. To retain as much structural strength as possible, clinicians should consider the use of instruments with a limited maximum flute diameter to accomplish this step, thereby restricting the preparation size to a conservative dimension and avoiding an over-flaring that would reduce dentinal walls’ thickness and structural strength. There is evidence to indicate that preserving pericervical dentine (4 mm above and below the crestal bone) is crucial for the distribution of functional stresses and hence tooth strength and long-term viability of the entire complex [41]. Finite element analysis has demonstrated that masticatory stresses are reduced when even small amounts of this pericervical dentine are preserved [42]. Moreover, appropriate early coronal flaring is also beneficial for the earlier access of disinfecting irrigation solutions, better tactile control of hand instruments during negotiation and the easier placement of files in the delicate apical third [1].
At this point and with a good understanding of root canal anatomy, negotiation with small, precurved stainless steel hand instruments to the root canal terminus is typically possible. The negotiation step allows the exploration of the individual anatomy of a root canal to its terminus.
Best practice during negotiation includes the use of small files in a ‘watch-winding’ hand motion in connection with an apex locator and in presence of a viscous chelator to prevent pulp tissue blockage [1]. Working length is determined with an electronic apex locator at this point and later on properly confirmed with a periapical radiograph. Any canal should be explored to patency, that is, until a small negotiation file (e.g., 08 or 10) reaches the periodontal ligament, as indicated by a well-defined colour reading of an apex locator.
Proper negotiation is another of the key factors during cleaning and shaping. Experience enhances the ability to negotiate root canals with difficult anatomy to the terminus. Inability to negotiate the root canal to the terminus increases the chance of a failing root canal treatment. Specifically, in canals with substantial curves, appropriate curves should be applied to negotiation instruments. Files should move along the root canal with watch-winding movements that preserve apical patency and do not push dentine shavings apically [43].
The goal during negotiation is not only to achieve working length but also apical patency. Patency of the apical foramen needs to be maintained during the rest of the shaping procedure [44]. A patency file can be defined as a small flexible K-file that passively moves through the apical constriction without widening it [45].
Apical patency is a ‘technique where the apical portion of the canal is maintained free of debris by recapitulation with a small file through the apical foramen’ [46]. The patency concept has historically been controversial. One of the cited reasons for not using apical patency is the possible extrusion of debris through the apical foramen [47], a condition often considered to result in postoperative pain and possibly delayed healing [48]. However, it has been demonstrated in laboratory studies that even if a patency file is contaminated, it is just disinfected by the 5.25% sodium hypochlorite (NaOCl) present in the root canal during the shaping procedures [49]. Some laboratory [49, 50] and in vivo [44] evidence suggests that keeping canals patent is associated with better outcomes. At the same time, maintaining apical patency benefits root canal preparation by:
13.3.3 Working Length and Patency
In fact, the most predictable method to prevent blockage is to regularly use the so-called patency file during cleaning and shaping procedures. Laboratory studies have also reported benefits in irrigation due to the enhancement of irrigant delivery to the apical third [53, 54] while reducing the gas bubbles that may prevent the advancement of fluids to working length [55]. The mere presence of NaOCl in the apical root canal third does not guarantee the proper cleaning of the entire root canal. As discussed elsewhere in this book, sufficient time and surface contact is needed for the dissolution of organic matter by NaOCl [56]. However, while patency in laboratory-based studies may be connected to small amounts of irrigant to reach the periodontium, maintaining patency does not appear to increase the chance of irrigation accidents clinically [57], and it does not increase the chance of postoperative pain [58]. For these reasons, the benefits of maintaining apical patency seems to outweigh the possible risks and it has been reported as a significant factor for a better endodontic prognosis [44].
Working length (WL) is defined as the distance from a coronal reference point to a point at which canal preparation and canal filling should terminate. Although both endpoints included in the definition are important and require attention; the apical endpoint of the prepared shape in relation to the apical anatomy has been one of the most discussed topics in the endodontic literature.
Several definitions of anatomical structures at the root canal end are important for working length definition; the reader is referred to chapter 1 on apical root canal morphology for details.
Classically, the ideal apical endpoint of root canal treatment was thought to be at the narrowest diameter of the canal, at the conceptual apical constriction. It was believed that this point coincided with the cemento-dentinal junction (CDJ), based on histologic sections and ground specimens. However, as stated in the definition, the position and anatomy of the CDJ varies considerably from tooth to tooth, from root to root, and from wall to wall in each canal. At the same time, a clear apical constriction cannot be found in many root canals in which the apical portion of the canal has been shown to be tapered or parallel [59]. For this reason, some authors have advocated terminating the preparation in necrotic cases at 0.5 to 1 mm short of the radiographic apex and 1 to 2 mm short [60, 61] in cases involving irreversible pulpitis, although there is no definitive validation for this strategy at present [61].
Working short presents higher risks of accumulation and retention of debris, which in turn may result in apical blockage and may contribute to procedural errors in the first place; furthermore, the infected debris, bacteria, and their by-products can remain in the most apical portion of the canal in cases with pulpal necrosis jeopardizing apical healing and contributing to a persistent or recurrent apical periodontitis [62, 63] or post-treatment disease [9, 64].
Moreover, most publications on outcomes that extrapolate the impact of apical termination are retrospective. On the other hand, a prospective study demonstrated that not only the maintenance of apical patency but also the apical extent of canal cleaning is a significant prognostic factor for root canal treatment, recommending to extend canal cleaning as close as possible to its apical terminus. In that study, the odds of success were reduced by 12% for every 1 mm of the canal short of the terminus remaining ‘un-instrumented’ [44].
The selection of an adequate coronal reference point during WL determination is also important. Some clinicians advocate using the same coronal reference for all root canals in the same tooth to ease the procedure; however, the proper determination of a straight and stable reference localised in the original path of the instrument when shaping each canal will avoid procedural mishaps during canal preparation. This concept has become very important with the generalisation of the use of rotary instruments. To avoid stresses exerted on the rotary instrument, it neither should be stopped in the interior of the root canal, nor should its direction be manipulated to check if the length is accurate. The more visible the reference point, the better the clinician can check the proper shaping length.
Best practices for WL determination include the prior estimation of WL from properly angulated preoperative periapical radiographs, the additional determination of WL with an apex locator in a canal that contains an appropriate amount of electrically conducting medium (e.g. a chelator or ion-containing irrigation solution) and proper verification with a periapical radiograph), and the determination of WL only after coronal modification has been accomplished.
While specific manufacturer guidelines may vary, electronic apex locators work best with some, but not too much, electrically conducting fluid in the root canal space. A small file is advanced until the apex locator indicates apical patency and then pulled back. Apex locators can produce irregular signals in large, fluid-filled canals and so it is desirable to check the working length with larger files that come closer to binding at the foramen. The better the adaptation of the tip of the instrument to the foramen, the more accurate the length determination.
13.3.4 Glide Path Preparation
The next step prior to canal shaping is the creation of a glide path to WL. The presence of a glide path is essential for the predictable use of rotary instruments because it minimises the so-called threading-in effect [65] and risk of torsional fracture of instruments. One possible explanation is that a glide path reduces the risk of taper lock that can occur if the canal cross-section is smaller than the tip of the instrument [66]. Therefore, a glide path reduces the contact area between subsequent shaping instruments and the canal wall and therefore the torsional loads induced by shaping rotary instruments [67].
It is advocated to explore and shape a root canal at least to a size 15 instrument before the use of a rotary NiTi instrument to full working length [68]. This instrument size is typically large enough for the safe advancement of the rotary or reciprocating shaping instrument tip [69] and small enough so as not to create canal transportation. The achievement of an appropriate glide path is indicated by the fact that a straight size 15 K-file can passively and smoothly travel to working length with long gentle in-and-out movements.
Conventionally, glide path preparation was performed and secured with K-files sizes 10 and 15 used in watch-winding or balanced-force motion. However, NiTi rotary instruments with small tip diameter and small taper have been designed specifically to simplify the process after a size 10 K-file has reached WL.
Preparation and confirmation of a rotary or reciprocating glide path have demonstrated better preservation of the canal anatomy and fewer aberrations [70–72], and have shown fewer incidents of postoperative pain [73, 74]. However, some studies have also reported the good performance of manual instruments for glide path preparation in terms of apical transportation [75] or changes in the angle of canal curvature [76] when compared with rotary glide path preparation. The advancement in rotary and reciprocating shaping files have also been applied to instruments designed for glide path preparation.
Despite the similarity in the operation with shaping files systems, the different variable taper and maximum flute diameter of mechanised glide path files could affect the stress distribution pattern and the torsional behaviour since those are the first instruments touching the entire canal wall [77, 78]. Increased diameter and taper also increased the contact surface with the dentine so that the instrument could suffer from cyclic fatigue. In fact, it has been reported that instruments designed for glide path preparation with greater taper and diameters exerted maximum forces in the root canal close to those of shaping instruments [79]. Considering that these instruments are not required to shape the entire root canal, but only to prepare enough space in the last millimetres for an easy advancement of rotary or reciprocating shaping instruments, it seems intuitive that low peak torque and force should be used during glide path preparation [79]. Reciprocating instruments have also been designed for glide path preparation.
After establishing a reproducible glide path, shaping the root canal can be initiated. There are many systems available and so far, no agreement exists either in the literature or among clinicians and educators regarding the best instruments to prepare a root canal. Hence, the purpose of this section is not to recommend a specific shaping system but to focus on generic rules to accomplish an ideal final shape that should accomplish an ideal disinfection and canal filling while avoiding pitfalls and maintaining the greatest amount of dentine for further resistance of the tooth.
13.3.5 Canal Preparation
With the use of NiTi and mechanical shaping, one of the main recommendations in this section will focus on the avoidance of instrument fracture. The following advice will help to prevent this from occurring by optimal case selection, avoiding cyclic fatigue (careful shaping of curved canals, reducing coronal interference, controlled number of use of instruments), avoiding torsional stress (glide path preparation, good selection of torque settings and motions to prevent threading-in of the file, and recapitulation to avoid taper lock are recommended practices), periodical cleaning of flutes to avoid accumulation of debris or gel in the flutes for a correct functioning of the instrument, and considering new developments and research to more resistant and efficient instruments [1].
In relation to the technique, manufacturers’ directions for use are generally recommended. Depending on the shaping system selected, there are three general approaches:
- Crown down: The main approach for many years and still being used with some rotary systems. Canal preparation can occur in two different forms: a) beginning with a specific taper and maintaining the taper but descending tip diameters while advancing apically in the root canal (example: size 40, .06 taper, followed by size 35, .06 taper, then 30, .06 and 25, .06); and b) diminishing progressively the taper of the instrument when moving apically but maintaining a constant tip diameter (example: size 25, .10 taper, followed by size 25, .08 taper and 25, .06 and finally 25, .04 if a file size 25, .06 does not reach working length).
- Single length: All the instruments are passively inserted to the full WL until achieving the desired apical diameter.
- Hybrid techniques: A combination of different hand/rotary/reciprocating systems to gain efficacy and conservative preparations in anatomical challenges. A detailed knowledge of properties, benefits, and limitations of each system is required for the appropriate selection of the instrument that will shape each canal third.
For ergonomic purposes and to help clinicians identify the correct length of each canal while the instrument keeps rotating, manufacturers place engraved lines at different lengths (18, 19, 20 and 22 mm) in the shanks of rotary instruments. Using any technique and system, preparation continues until the desired final shape is achieved. Morphology of root canals varies, therefore there should not be a standardised apical canal size. Each independent canal with different preoperative root dimensions requires a different apical preparation. Having in mind a fixed preparation size for a type of root canal results in gross over-enlargement of most canals and under-treatment of apically resorbed ones [80]. Appropriate irrigation sequence and activation of the irrigant solutions is also mandatory and described in chapter 11.
13.4 Physical Properties of Engine-driven Root Canal Instruments
In terms of selecting a proper shaping system, it is important to understand typical properties related to design, alloy, and motion of the instruments. Especially important is to be aware of cutting efficiency, torsional performance, and CF resistance. In fact, the performance of all contemporary instruments manufactured with a different concept than pre-existing ones should be tested in all the cited parameters before clinical use.
13.4.1 Cutting Efficiency
Endodontic instruments should be cutting efficiently to allow the removal of dentine and facilitate root canal preparation for both lateral (‘brushing’ motion) or axial cutting while penetrating to WL. Although the cutting ability of different instruments has been compared in rotation and acceptable cutting efficiency reported for both martensitic and austenitic alloys [81, 82], studies comparing the cutting efficiency of rotary versus reciprocating shaping instruments are sparse. Gambarini et al. [83] reported similar lateral cutting efficiency after prolonged use when the same instrument was actioned with different motions.
In terms of glide path preparation, the kinematics used have an influence in the cutting capacity of instruments. In fact, reciprocating instruments have demonstrated greater cutting efficiency than those used in continuous rotation. Moreover, further inclination (45º or 70º) in the contact between the instrument and the canal wall increased the cutting ability of instruments for glide path preparation [84].
13.4.2 Cyclic Fatigue Resistance
Instrument fracture remains a major concern of clinicians [85]. The fracture of engine-driven NiTi instruments can occur either due to CF or torsional failure. While CF fractures are the result of alternating compressive and tensile forces experienced by a rotating instrument in a curved canal, torsional fractures occur when some part of the instrument binds in the root canal while the shank continues to rotate. It has been shown that CF is a major cause of instrument fracture [86].
The absence of standardised specifications to test CF resistance of rotary NiTi makes it difficult to compare the results of different studies. Moreover, most studies have been performed at room temperature. One clinical study showed that room temperature NaOCl placed in root canals rapidly approaches body temperature [87]. Newer alloys present higher transformation temperatures than those of conventional austenitic materials [88] that may in fact transform close to body temperature [29]. Several studies have confirmed the different fatigue behaviour of rotary instruments when tested at room versus either at body [89, 90] or intracanal [91] temperatures.
Despite the wide range of testing conditions and methodologies that have been used to compare CF resistance of rotary endodontic instruments; this aspect is probably the one with the highest level of consensus among studies for rotary and reciprocating instruments. The reciprocating motion seems to relieve stress on the instrument by special counterclockwise (cutting action) and clockwise (release of the instrument) movements and, therefore, reduces the risk of CF caused by alternating compressive and tensile forces experienced by a rotating instrument in a curved canal. A systematic review revealed that reciprocating motion improves the fatigue resistance of endodontic instruments, compared to continuous rotation, independent of other variables such as the speed of rotation, the angle or radius of curvature of simulated canals, geometry and taper, or the surface characteristics of the NiTi instruments [92].
Reciprocating movement resulted in extended fatigue lifespan for both rotary endodontic instruments originally produced to operate in continuous rotation and tested using different reciprocating motions to compare their lifespans after use [93–95], as well as instruments specially designed for use in reciprocating motion [96–98]. Moreover, manufacturers’ guidelines recommend not to reuse reciprocating instruments and have placed a nonsterilisable plastic in the handle that limits a second use. Both combined the short usage time, and the extended lifespan of these instruments limits the risk of fatigue fracture of instruments in reciprocating motion in the clinical setting. New heat treatment processes (i.e. Gold and Blue) have also increased the CF resistance of previous-generation reciprocating instruments [99, 100].
13.4.3 Torsional Performance
Rotary and reciprocating instruments may also fracture due to torsional loading when the tip of the rotating instrument binds in the canal and prevents the free rotation of the instrument. It has been reported that rotary instruments fracture clinically often in the apical 3–5 mm and for this reason torsional failure of rotary instruments is simulated in laboratory studies by clamping the apical 3 mm and loading the instrument until fracture, according to the ISO standard 3630-1 or ADA No. 28 originally written for hand instruments. It can also be assessed using numerical simulation. Finite Element Analysis (FEA) allows the further benefit of estimating instrument performance simulating different alloys or design changes.
FEA studies have analysed the torsional behaviour of different NiTi rotary files [101, 102] and hinted at cross-sectional configurations more prone to fracture by torsional stresses [103]. Another aspect of such studies (Figure 13.4) is the improvement of torsional stiffness by increasing the taper and the helix angle or decreasing the flute length [104] and the distribution of stress from the periphery to the centre in different cross-sections of NiTi rotary files [105–107]. It is also very useful to numerically evaluate stresses of instrument with complicated design and motions like those of reciprocating instruments.

Figure 13.4 Torsional behaviour of nickel-titanium instruments simulated in Finite Element Analysis (FEA) showed the typical biphasic curve, due to the martensitic transformation for both M-Wire and SE stock (a). In a simulated twist according to ISO3630-1, stress distribution depends on cross-sectional design at D3 (b). Reproduced from Bonessio et al. [108] / with permission from John Wiley & Sons.
For example, WaveOne (originally manufactured from M-Wire) has been compared to the same virtual instrument but made of SE-NiTi, and researchers have reported how changes in alloy properties can considerably affect the performance of WaveOne instruments (see Figure 13.5). M-Wire instruments demonstrated better torsional behaviour due to the considerably higher flexibility at small deflections with respect to NiTi instruments [108].

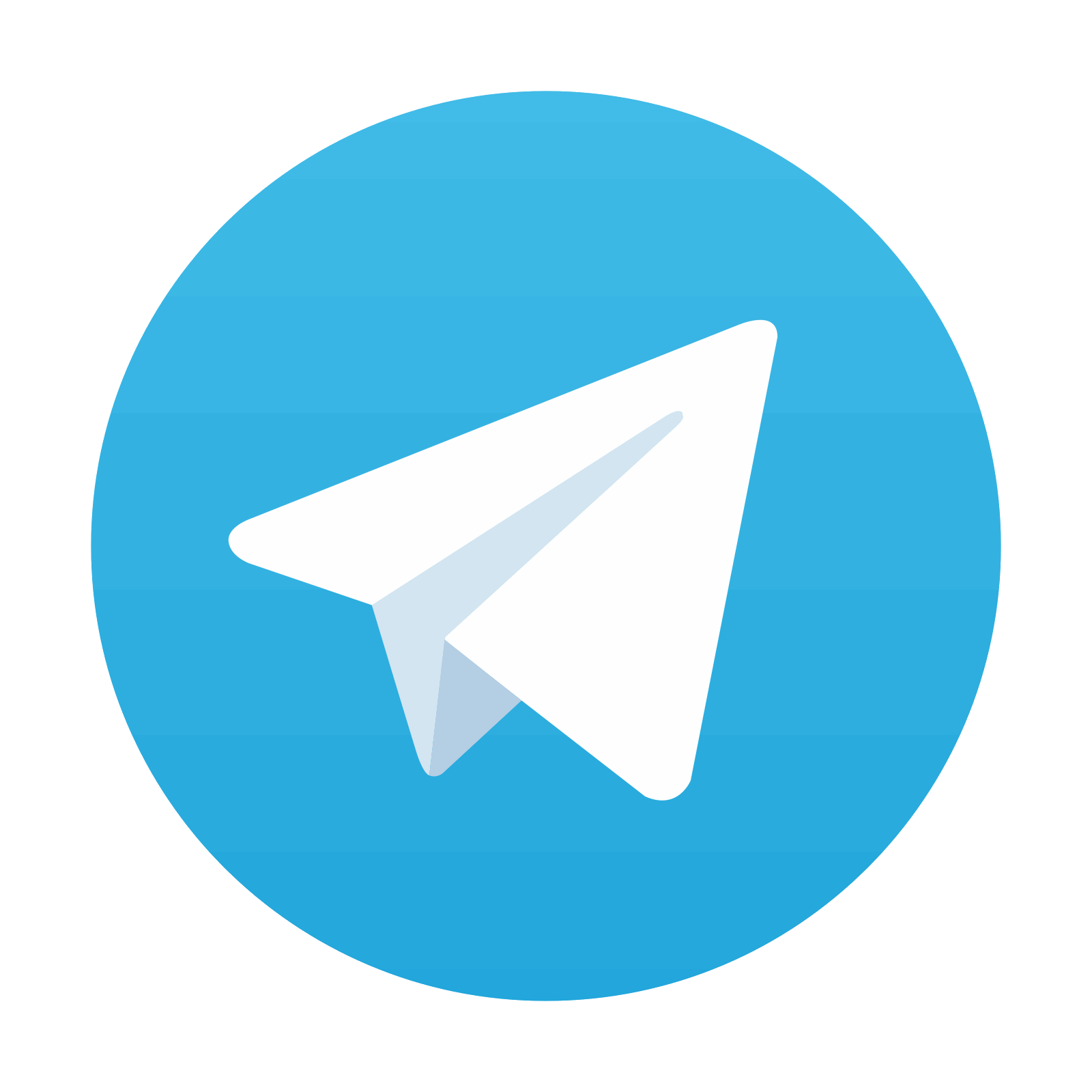
Stay updated, free dental videos. Join our Telegram channel

VIDEdental - Online dental courses
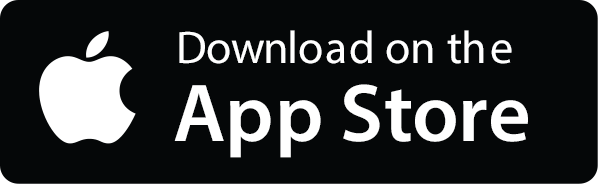
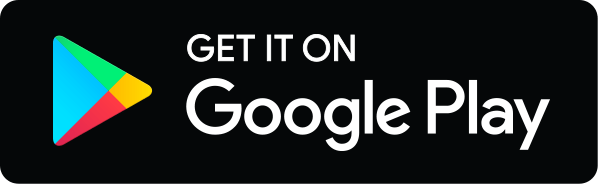