Abstract
Objectives
To examine effects of shrinkage and modulus on the dynamic development of shrinkage stress as a function of methacrylate conversion and filler loading in a model photocurable dimethacrylate-based resin with a silanized barium glass filler.
Methods
BisGMA/TEGDMA samples with filler loading levels of 0–70 wt% were evaluated. Irradiation times and intensities were varied to achieve a wide range of conversion. Shrinkage stress measurements were accompanied with real-time conversion monitoring, while shrinkage and modulus measurements were made at different static conversion points.
Results
Shrinkage increased nearly linearly with respect to conversion, while for a given value of conversion, it decreased proportionally with increasing filler content. Modulus advanced in an exponential fashion with conversion and also increased incrementally with filler content; however, modulus values rose disproportionately rapidly for the highest filler loading. At either high or low filler loading levels, stress at limiting conversion, which was inversely proportional to the filler load, was high while at an intermediate filler content, a minimum in stress was observed due to the combined effects of filler based shrinkage reduction, restricted limiting conversion and only moderately enhanced modulus. The level of polymerization stress predicted from the conversion-indexed shrinkage and modulus measurements over-estimated the experimental stress states as modulus evolved due to system compliance that to some degree mimics the clinical situation presented by photocuring bonded composite restorations.
Significance Measurement of monomer conversion provides a common basis by which different material properties can be rationally compared.
1
Introduction
Dental composite restoratives comprise a tremendously large segment of the biomaterials market. While these esthetic materials continue to be improved, polymerization shrinkage and the resulting shrinkage stress can be extremely detrimental to the performance of polymer-based dental restoratives . The vast majority of these dental restoratives continue to be dimethacrylate-based and the monomers undergo significant shrinkage during polymerization . In a clinical setting, shrinkage is restricted as the polymerizing composite is strongly attached to the tooth substrate to maintain mechanical integrity and an effective seal between the restoration and tooth. Interfacial failure can result in marginal leakage between the tooth and the restoration further leading to problems such as marginal staining, secondary caries and post-operative sensitivity . This constraint placed on shrinkage as the viscous composite paste converts to a glassy, high modulus material is the primary source for the buildup of stress within the composite . The resulting stress is also transferred to the adhesive interface and to the adjacent tooth structure. The magnitude of the stress that develops during polymerization depends on the shrinkage and modulus of the composite , the interaction between the reinforcing filler and the developing polymer matrix and the boundary conditions under which the composite is cured . It has also been suggested that the exotherm generated due to the polymerization reaction is an additional factor that affects stress development . All the parameters responsible for stress development are affected by the formulation of the composite, which at the most basic level is composed of an organic resin matrix, reinforcing filler particles, coupling agent between the resin matrix and filler and an initiator system.
Since their introduction in the 1950s, polymeric dental restoratives have undergone significant changes in their formulation , along with a steady improvement in performance. Until the 1990s, most of the research contributing towards the evolution of dental composites was in the area of filler development . Filler sizes and composition changed drastically down from dimensions of 10–50 μm or more in the early dental composites to sub-micron range and even extending down to 5 nm in some of the modern nanocomposites . Along with variations in size and composition of the fillers, the filler loading level has also been varied over a considerable range. The volume fraction of fillers in commercial composites can span from about 20% up to about 75% . Low filled composites typically show lower moduli and strength but exhibit significant flow and adaptability prior to curing. Materials of this type are used in applications where there are limited masticatory forces and as flowable composite liners that produce a somewhat adaptable interface . Highly filled composites are used in posterior restorations where there is a need for sustaining higher recurring loads and greater wear resistance. As shrinkage is one of the main factors leading to stress formation in polymerizing dental restoratives, research over the last few years has focused on developing low shrinkage resins . The changes in formulations also lead to a range of vitrification-limited double bond conversion levels of the various resin systems that comprise the matrix phase of the composite. The practical variation in polymer conversion is also expanded by differences in photocuring conditions (i.e., wavelength, irradiance, exposure time) as well as differences in light transmission character of composites due to their composition .
This work examines the development of shrinkage, stress and flexural modulus as a resin converts from the uncured state to a fully cured material, with methacrylate conversion used as a coordinating index to look at interrelationships between these properties as a function of varied filler loading. Several studies have probed the effect of filler loading on shrinkage, modulus and stress in isolation or in some combination . However, only a few report the functional group conversion levels at which the property measurements are actually done. The contention here is that an accurate assessment of conversion is necessary to accompany any property measurement that displays conversion dependence to ensure that comparisons between different materials or even within a given material are legitimate. There is a lack of understanding of the effect of filler loading and monomer conversion on shrinkage, modulus and stress as well as how all these factors relate to the development of physical and mechanical properties of composite materials. Given a certain formulation, shrinkage stress would depend on shrinkage and modulus, the C-factor (configuration factor, representation of the constrained or bonded to unbonded surface area), viscoelastic behavior of the material, as well as the curing and boundary conditions. In this study the C-factor and boundary conditions were kept constant for different samples in the same measurement class.
2
Materials and methods
2.1
Materials
2,2-Bis[4-(2-hydroxy-3-methacrylyloxypropoxy)phenyl]propane (Bis-GMA) and triethylene glycol dimethacrylate (TEGDMA) (both from Esstech, Essington, PA) were used in a 70:30 mass ratio as a model dental resin. A visible light initiating system consisting of 0.3 wt% camphorquinone (CQ) as initiator and 0.8 wt% ethyl 4-dimethylaminobenzoate (EDAB) (Sigma–Aldrich, Milwaukee, WI) as co-initiator was incorporated. Barium glass (mean particle size 0.7 μm) surface treated with a coating of 5 wt% γ-methacryloxypropyltrimethoxysilane (γ-MPS), was used as the inorganic filler (Esstech). The filler was mixed with resin in varying amounts to obtain total final weight percentages of 0, 20, 40, 60 and 70, which correspond to filler volume fractions of 0, 9.4, 21.6, 38.3 and 49.1 respectively, based on densities of filler (2.71 g/cm 3 ) and uncured resin (1.12 g/cm 3 ) . Fillers and resin were blended in a centrifugal mixer (DAC 150 FVZ, Flakteck) to ensure thorough mixing.
2.2
Sample curing
Visible light irradiation was provided by a halogen dental curing lamp (VIP, Bisco, Schaumburg, IL). Light intensities and irradiation times were varied in order to achieve a wide range of conversions for specimens used in the various testing protocols. A radiometer (Model 100, Demetron Research, Danbury, CT, USA) was used to measure the output light intensity.
2.3
Infrared spectroscopy
Double bond conversion in the resin and composite specimens was monitored in transmission mode using near-infrared (NIR) spectroscopy (Nexus 670, Nicolet Instruments, Madison, WI). The area of the peak at 6165 cm −1 signifying the CH 2 absorption was used to track the double bond conversion. All NIR measurements were taken at a wavenumber resolution of 4 cm −1 . For the static measurements, 64 scans per spectrum were taken for, while 2 scans per spectrum were used for collection of real-time series measurements with approximate 1 Hz sampling temporal resolution.
2.4
Shrinkage stress measurement
Shrinkage stress was measured using a cantilever beam-based tensometer, which was designed and fabricated at the Paffenberger Research Center of the American Dental Association Health Foundation (ADAHF–PRC, Gaithersburg, MD, USA). This device measures the dynamic tensile forces generated by a polymerizing sample that causes deflection of the calibrated beam. A more detailed description of the experimental technique has been reported in a previous study . Briefly, a composite sample is placed between two cylindrical quartz rods that have been treated with a methacrylate functional silane to promote bonding with the resin in the composite. The lower quartz rod is fixed whereas the upper quartz rod is attached to the cantilever. A curing light is connected by an adapter to the bottom of the lower quartz rod and light is transmitted through the rod to the sample causing polymerization and deflection of the cantilever with system compliance determined by positioning on the beam and designed to generally reflect tooth flexure. An LVDT (linear variable differential transformer) continuously tracks the beam deflection and this data is converted to a force measurement, which when divided by the composite cross-sectional area, provides the stress in the composite. The cavity in which the samples were placed was 6 mm in diameter and 1.5 mm in thickness. For the simultaneous measurement of conversion with shrinkage stress, the NIR signal was guided to/from the sample using optical fiber cables (1 mm diameter).
For the stress measurements, the samples ( n = 3) were irradiated with the VIP curing light at an incident irradiance of 250 mW/cm 2 for 60, 10 and 5 s and also at 35 mW/cm 2 for 20, 10 and 5 s, to obtain a wide range of conversion and associated stress values. Irradiance was measured at the end of the quartz rod in contact with the sample. The coupled stress/conversion data was collected continuously for 15 min.
2.5
Shrinkage measurement
Shrinkage was measured using a linometer (ACTA Foundation, Netherlands). In this method a liquid monomer or uncured composite paste sample was placed between a fixed upper glass plate and a freely moving aluminum disc, both of which were lightly greased to provide radial freedom of movement for the shrinking sample, which allows the linear post-gel shrinkage to be recalculated in terms of volumetric shrinkage. Disc specimens ( n = 3) of 6 mm in diameter and 1.5 mm in thickness were irradiated from the upper surface and the displacement of the aluminum disc was monitored by a LVDT. Irradiation intensity and times were used to coincide with those used for the stress measurements. Shrinkage was monitored continuously for 10 min. NIR scans of each specimen were taken prior to polymerization and immediately after removal from the linometer to avoid any significant post-cure conversion development.
2.6
Flexure modulus measurement
Flexural modulus was measured in three-point bending (MTS 858 Mini Bionix II). The specimen dimensions were 2 mm × 2 mm × 10 mm. Samples ( n = 5) were irradiated on one side and immediately inverted and irradiated under equivalent conditions from the opposite side to optimize conversion uniformity throughout the sample. A range of light intensities, from 25 to 575 mW/cm 2 , were used. Samples were cured for 30–60 s on each side. Conversion was measured by NIR spectroscopy directly from the flexural test specimens before and immediately after curing. As soon as the NIR polymer spectrum was collected, the sample was submitted to mechanical testing. Again this was done to ensure well correlated conversion and mechanical property results.
2.7
Vicker’s microhardness test
Disc-shaped specimens (2 mm thickness and 10 mm diameter, n = 3) of each formulation were obtained by photopolymerization with the dental light at 700 mW/cm 2 for three minutes on each flat surface. The Vicker’s hardness was measured using a microhardness tester (LM700AT, Leco, St. Joseph, MI). The specimens were indented with a diamond tip using a force of 300 gf for 15 s. The length of the indents was measured and the VHN was calculated using the following formula:
H = 1854 × 4 F d 2
where F is the applied force in gf and d is the size of the indent in millimeters.
2
Materials and methods
2.1
Materials
2,2-Bis[4-(2-hydroxy-3-methacrylyloxypropoxy)phenyl]propane (Bis-GMA) and triethylene glycol dimethacrylate (TEGDMA) (both from Esstech, Essington, PA) were used in a 70:30 mass ratio as a model dental resin. A visible light initiating system consisting of 0.3 wt% camphorquinone (CQ) as initiator and 0.8 wt% ethyl 4-dimethylaminobenzoate (EDAB) (Sigma–Aldrich, Milwaukee, WI) as co-initiator was incorporated. Barium glass (mean particle size 0.7 μm) surface treated with a coating of 5 wt% γ-methacryloxypropyltrimethoxysilane (γ-MPS), was used as the inorganic filler (Esstech). The filler was mixed with resin in varying amounts to obtain total final weight percentages of 0, 20, 40, 60 and 70, which correspond to filler volume fractions of 0, 9.4, 21.6, 38.3 and 49.1 respectively, based on densities of filler (2.71 g/cm 3 ) and uncured resin (1.12 g/cm 3 ) . Fillers and resin were blended in a centrifugal mixer (DAC 150 FVZ, Flakteck) to ensure thorough mixing.
2.2
Sample curing
Visible light irradiation was provided by a halogen dental curing lamp (VIP, Bisco, Schaumburg, IL). Light intensities and irradiation times were varied in order to achieve a wide range of conversions for specimens used in the various testing protocols. A radiometer (Model 100, Demetron Research, Danbury, CT, USA) was used to measure the output light intensity.
2.3
Infrared spectroscopy
Double bond conversion in the resin and composite specimens was monitored in transmission mode using near-infrared (NIR) spectroscopy (Nexus 670, Nicolet Instruments, Madison, WI). The area of the peak at 6165 cm −1 signifying the CH 2 absorption was used to track the double bond conversion. All NIR measurements were taken at a wavenumber resolution of 4 cm −1 . For the static measurements, 64 scans per spectrum were taken for, while 2 scans per spectrum were used for collection of real-time series measurements with approximate 1 Hz sampling temporal resolution.
2.4
Shrinkage stress measurement
Shrinkage stress was measured using a cantilever beam-based tensometer, which was designed and fabricated at the Paffenberger Research Center of the American Dental Association Health Foundation (ADAHF–PRC, Gaithersburg, MD, USA). This device measures the dynamic tensile forces generated by a polymerizing sample that causes deflection of the calibrated beam. A more detailed description of the experimental technique has been reported in a previous study . Briefly, a composite sample is placed between two cylindrical quartz rods that have been treated with a methacrylate functional silane to promote bonding with the resin in the composite. The lower quartz rod is fixed whereas the upper quartz rod is attached to the cantilever. A curing light is connected by an adapter to the bottom of the lower quartz rod and light is transmitted through the rod to the sample causing polymerization and deflection of the cantilever with system compliance determined by positioning on the beam and designed to generally reflect tooth flexure. An LVDT (linear variable differential transformer) continuously tracks the beam deflection and this data is converted to a force measurement, which when divided by the composite cross-sectional area, provides the stress in the composite. The cavity in which the samples were placed was 6 mm in diameter and 1.5 mm in thickness. For the simultaneous measurement of conversion with shrinkage stress, the NIR signal was guided to/from the sample using optical fiber cables (1 mm diameter).
For the stress measurements, the samples ( n = 3) were irradiated with the VIP curing light at an incident irradiance of 250 mW/cm 2 for 60, 10 and 5 s and also at 35 mW/cm 2 for 20, 10 and 5 s, to obtain a wide range of conversion and associated stress values. Irradiance was measured at the end of the quartz rod in contact with the sample. The coupled stress/conversion data was collected continuously for 15 min.
2.5
Shrinkage measurement
Shrinkage was measured using a linometer (ACTA Foundation, Netherlands). In this method a liquid monomer or uncured composite paste sample was placed between a fixed upper glass plate and a freely moving aluminum disc, both of which were lightly greased to provide radial freedom of movement for the shrinking sample, which allows the linear post-gel shrinkage to be recalculated in terms of volumetric shrinkage. Disc specimens ( n = 3) of 6 mm in diameter and 1.5 mm in thickness were irradiated from the upper surface and the displacement of the aluminum disc was monitored by a LVDT. Irradiation intensity and times were used to coincide with those used for the stress measurements. Shrinkage was monitored continuously for 10 min. NIR scans of each specimen were taken prior to polymerization and immediately after removal from the linometer to avoid any significant post-cure conversion development.
2.6
Flexure modulus measurement
Flexural modulus was measured in three-point bending (MTS 858 Mini Bionix II). The specimen dimensions were 2 mm × 2 mm × 10 mm. Samples ( n = 5) were irradiated on one side and immediately inverted and irradiated under equivalent conditions from the opposite side to optimize conversion uniformity throughout the sample. A range of light intensities, from 25 to 575 mW/cm 2 , were used. Samples were cured for 30–60 s on each side. Conversion was measured by NIR spectroscopy directly from the flexural test specimens before and immediately after curing. As soon as the NIR polymer spectrum was collected, the sample was submitted to mechanical testing. Again this was done to ensure well correlated conversion and mechanical property results.
2.7
Vicker’s microhardness test
Disc-shaped specimens (2 mm thickness and 10 mm diameter, n = 3) of each formulation were obtained by photopolymerization with the dental light at 700 mW/cm 2 for three minutes on each flat surface. The Vicker’s hardness was measured using a microhardness tester (LM700AT, Leco, St. Joseph, MI). The specimens were indented with a diamond tip using a force of 300 gf for 15 s. The length of the indents was measured and the VHN was calculated using the following formula:
H = 1854 × 4 F d 2
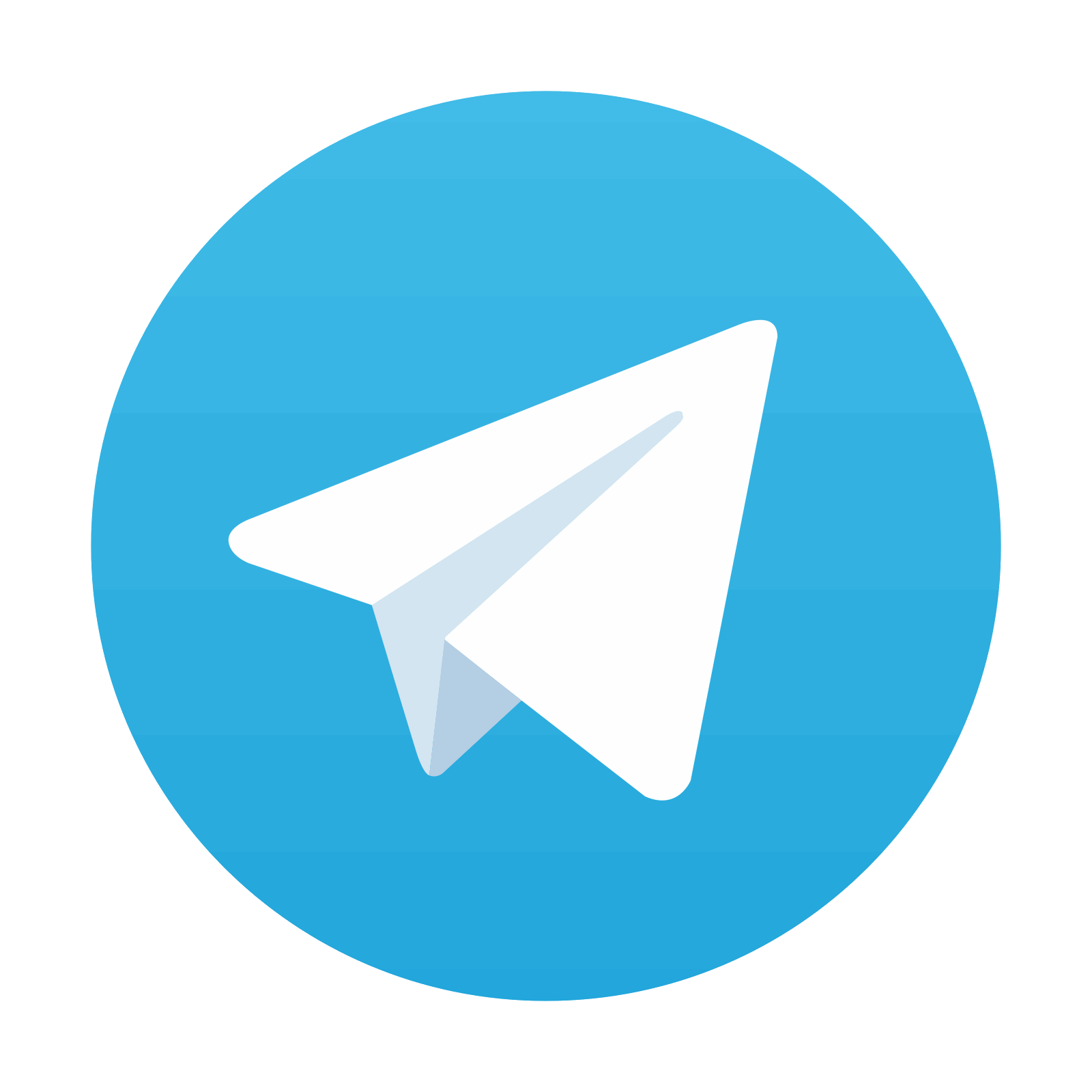
Stay updated, free dental videos. Join our Telegram channel

VIDEdental - Online dental courses
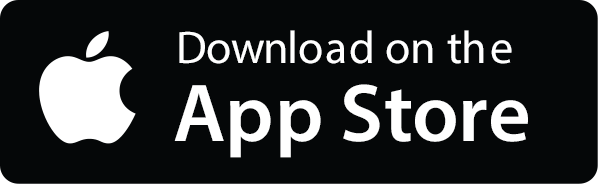
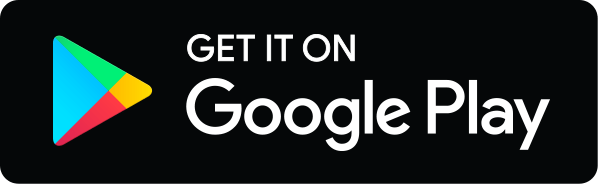