Abstract
Objectives
To evaluate the collagen cross-linkers, riboflavin-ultraviolet-A (RF/UVA) and glutaraldehyde, with regard to their efficacy in cross-linking the dentinal collagen and improving dentin bonding.
Methods
Glutaraldehyde and different RF/UVA protocols (0.1%RF/1-minUV, 0.1%RF/2-minUV, and 1%RF/1-minUV) were first evaluated by gel electrophoresis to determine their abilities of collagen cross-linking. The mechanical properties of acid-etched dentin receiving these cross-linking treatments were examined in either dry or wet condition by a nanoindentation test. Fifteen teeth with exposed occlusal dentin received the microtensile bond strength (μTBS) test. The teeth were primed either with RF/UVA or glutaraldehyde, followed by adhesive treatment and composite restorations, and then cut into resin-dentin microbeams. Half of the microbeams received the μTBS test after 24 h, and the other half received test after 5000 thermocycles. Nanoleakage at the bond interface was examined under TEM. The alignments of collagen fibrils in the hybrid layers were also defined by an image analysis.
Results
Gel electrophoresis showed that glutaraldehyde induced strong collagen gelation, while RF/UVA generated milder collagen cross-linking. Glutaraldehyde, 0.1%RF/2-min-UVA, and 1%RF/1-minUV showed higher stiffness compared to untreated and 0.1%RF/1-minUV in wet condition. All the crosslinking treatments improved early μTBS, but 0.1%RF/2-minUVA treatment maintained high μTBS after theromocycles. Under TEM, glutaraldehyde-treated dentin showed dense and enclosed collagen network on the adhesive interface. 0.1%RF/2-minUVA showed the least nanoleakage, and this could be associated with the suspended collagen fibrils in the hybrid layer.
Significance
0.1%RF/2-minUVA treatment enhanced resin-dentin bond possibly through enhancing the stiffness and maintaining the expanding collagen matrix in the hybrid layer.
1
Introduction
Dentin is a complex mineralized tissue that consists of minerals, organic components, and water. The organic components of dentin consist of mostly type I collagen, and several noncollagenous proteins . The fibrillar collagen constitutes a network to support the dentin tissue and allows the accommodation of hydroxyapatite minerals . The basic unit of type I collagen is procollagen which is made up of two genetically identical α1 chains and one α2 chain . The intrinsic cross-links increase the structural stability and strength of dentin collagen fibers.
For contemporary dentin bonding procedures, the dentin is etched to be susceptible to the infiltration of resin monomers into the collagen fibrils. The resultant hybrid layer is crucial to establish effective and stable resin-dentin bonding . Although the collagen matrix is required to establish the hybrid layer, it is also responsible for the unstable adhesion. Collagen fibrils exposed after acid-etching may collapse during the air-drying process and impede the adhesive infiltration. The maintenance of expanding state of the exposed collagen network is technique-sensitive, and varies with the systems and dentin conditions . Collagen incompletely enclosed by adhesive is prone to a hydrolytic and chemical degradation . The mineral-depleted dentin may also activate a series action of endogenous collagenase to cause a rapid breakdown of the collagen framework, and eventually affects the durability of dentin adhesion .
With the advance of bioprostheses, synthetic and natural chemicals have been applied to enhance inter- and intramolecular collagen cross-linking and improve their structural stability . Recently, collagen cross-linking is proposed as an adjunct treatment of dentin adhesion to strengthen dentinal collagen and improve bond durability. Glutaraldehyde (GA) is a well-known collagen fixative for the preparation of implanted biomaterials. GA increases crosslinking through reacting with the amino groups of collagen fibrils. The reaction of GA may fix the collagen tissues quickly at a minimal dose . Some investigators advocated that applying GA during the bonding procedures effectively improved resin-dentin adhesion on both sound and carious dentin . However, contradictory studies showed that GA generates unstable collagen cross-linking on bovine dentin, and shows cytotoxicity to dental pulp . Recently, a number of natural cross-linkers, including proanthocyanidins, genipin, and tannic acid, have been shown to increase dentin stiffness and strengthen the resin-dentin adhesion . Moreover, carbodiimides have also been shown to enhance the stability of resin-dentin interfaces . With these promising results, the uses of collagen cross-linkers have been considered as strategies to improve dentin bonding.
Two mechanisms underlie the ability of collagen cross-linkers to improve dentin bonding. First, they maintain the collagen network in a relatively expanded state, which is a prerequisite for the interdiffusion of solvents and hydrophilic resin . The suspended collagen also reduces the technique sensitivity regarding drying dentin after acid-etching . Second, treated demineralized dentin is stiffer and thus more able to resist the plasticization effect of water. New findings revealed that these treatments also inhibited collagenase activity and reduced the biodegradation rate of dentin/adhesive interface . However, most cross-linkers need an immersion time of longer than 1 h, which limits their clinical use.
Riboflavin (RF) combined with ultraviolet-A (UVA) irradiation has been used for years in ophthalmology to strengthen the cornea . The high energy of UVA light (365 nm) breaks down weak intrinsic cross-links in the collagen and generates free oxygen radicals. Functional hydroxyl groups in RF attach to the proline or lysine in the collagen . Additionally, RF acts as a photosensitizer to UVA, thus promoting the formation of new cross-linkages . Applying RF/UVA in dentin adhesive treatment has been reported by Cova et al. . They found that a dentin treatment of a primer containing 0.1% RF and 2 min UVA irradiation increased bond strength and reduced interfacial nanoleakage. This treatment is also advocated in inactivating dentin matrix metalloproteinases, thus to improve the durability of resin adhesion.
A stable collagen network is essential to establishing a hybrid layer and preventing subsequent degradation, and RF/UVA induced collagen cross-linking appears a potential adjunctive treatment for dentin bonding. However, the facts about the optimal protocol of RF/UVA treatments in related applications are inadequate. The purpose of this study was thus to investigate the effects of various RF/UVA treatments on the resin-dentin adhesion via both mechanical and morphological evaluations. The null hypothesis tested was that the RF/UVA treatments would not change the stiffness and structure of collagen matrix in the hybrid layer, or affect the resin-dentin bonding.
2
Materials and methods
The study protocol was approved by the Institutional Review Board, National Cheng Kung University Hospital. Twenty sound extracted human molars were stored in 4 °C normal saline containing 0.02% sodium azide until use. These teeth were prepared for the nanoindentation and the microtensile bond strength (μTBS) tests.
2.1
Gel electrophoresis analysis
The cross-linking effect of RF/UVA on type I collagen was examined by sodium dodecyl sulfate–polyacrylamide gel electrophoresis (SDS-PAGE) . The test cross-linkers were 0.1% and 1% riboflavin-5′-phosphate (Sigma–Aldrich, St. Louis, MO, USA), which were separately added to 1.5 mg/ml bovine collagen solution (Sigma–Aldrich) in equal amounts. Collagen mixed with distilled water worked as the negative control. 5% GA (GlumaDesensitizer, Heraeus Kulzer, South Bend, IN, USA) and its diluent 0.5% GA were used as the positive control. The RF-collagen mixtures were irradiated under UVA light (Bio-LX E-365, 6 × 8 W, 365 nm, Vilber Lourmat, Marne-la-Vallée, France). The different riboflavin/UVA combinations to test were: 0.1% RF treatment followed by 1 min UVA irradiation, R0.1U1; 0.1% RF treatment followed by 2 min irradiation, R0.1U2; and 1% RF treatment followed by 1 min irradiation, R1U1.
Samples were diluted with sample buffer. After heat denaturation at 100 °C for 15 min, the sample was loaded onto an SDS-8% polyacrylamide separating gel with an SDS-4.5% stacking gel on top. Electrophoresis was carried out at 100 V Subsequently, the gels were stained with 0.1% Coomassie blue and photographed. Prestained molecular mass marker (Spectra Multicolour Broad Range Protein Ladder, Fermentas, Hanover, MD, USA) was run in a parallel lane as standard.
2.2
Nanoindentation test
For the nanoindentation tests, five 3 mm thick coronal dentin disks were prepared by cross-sectioning two molar teeth using a slow speed rotary saw (Isomet 2000; Buehler, Lake Bluff, IL, USA). The disks were embedded in epoxy resin, polished with abrasive papers (Nihon Kenshi, Osaka, Japan) to 1500 grit, and then by diamond suspensions to 1 μm. The dentin surface was divided into five tiny zones with area of 12–15 mm 2 , to receive various treatments: distilled water (as the control), R0.1U1, R0.1U2, R1U1, and GA. After acid-etching with 35% phosphoric acid (Ultraetch; Ultradent, Salt city, UT, USA) for 1 min, the experimental agents were applied on their respective zones by microbrushes (Benda MicroFine Applicators, Centrix, Santa Maria, CA, USA). The GA zone was treated with 5% GA (Gluma Desensitizer) for 1 min. The R0.1U2 zone was treated and irradiated under the UVA light for 1 min prior to the R0.1U1 and R1U1 zones.
The nanoindentation tests were conducted on a computer-controlled nanoindenter TI 700 UBI (Hysitron, MN, USA). The measurement was performed using a Vickers diamond indenter with a 50 nm radius, with a maximum load of 1000 μN at a load speed 50 μN/s. During indentation, the applied load and displacement were continuously recorded to derive the elastic modulus and nanohardness. For each group, at least twenty indentations were performed on the dentin disks in the dry condition. According to Kinney et al. , the nanoindentation test of the demineralized dentin in the hydrated state facilitates to determine the mechanical property of dentinal collagen matrix. Therefore, the same indentation test was also conducted after water-immersion for 30 min. The results were analyzed using the one-way ANOVA and post hoc Tukey tests for the differences among treatments at a 5% significance level. The differences in elastic modulus and microhardness under wet and dry conditions were analyzed by the t tests.
2.3
μTBS
Fifteen teeth were cut to expose coronal dentin, and then ground flat. The dentin surfaces were acid-etched for 15 s, water-rinsed, and then gently dried to prevent dehydration. The teeth were distributed into five treatment groups. The control received distilled water treatment for 1 min. The GA group was pretreated with 5% GA (Gluma Desensitizer) for 1 min. R0.1U1 and R0.1U2 were pretreated with 0.1% RF, and then UVA-irradiated for 1 or 2 min, respectively. R1U1 was treated with 1% RF and irradiated for 1 min. Subsequently, the dentin surface was treated with the Scotchbond Multi-purpose primer and adhesive (3M/ESPE, St. Paul, MN, USA) following the manufacturer’s instructions, and light cured for 20 s (Optilux 501; KerrHawe, Boggio, Switzerland). A microhybrid composite Z250 (3M/ESPE) was used to build up a 4 mm thick block in two increments, each light-cured for 40 s. After storage for 24 h in 37 °C distilled water, restored teeth were sectioned perpendicular to the bonded interface to generate 0.9 mm × 0.9 mm resin-dentin beams ( n = 24). Half of the resin-dentin beams received the μTBS test, while another half was further subject to 5000 thermocycles (5–60 °C) before the test. In the μTBS test, each beam was fixed to a jig on a universal testing machine (AG-1; Shimadzu, Kyoto, Japan), and stressed under tension at a crosshead speed of 1 mm/min until failure. μTBS was calculated by dividing the failure load by the precise cross-sectional area at the fracture site. Statistical analysis was performed by one-way ANOVA and Tukey tests.
To study the fracture patterns, these beams were mounted on aluminum stubs and gold sputtered. The fracture surfaces were examined with a scanning electron microscope (SEM) (JSM-6390 LV; JEOL, Tokyo, Japan) at 100× magnification. The fracture patterns were classified as: pre-test failure, adhesive failure, mixed adhesive and cohesive fracture, cohesive dentin fracture, and cohesive composite fracture. Data were analyzed using chi-square and Fisher’s exact tests.
2.4
Evaluation of nanoleakage and morphology of hybrid layer
Six resin-dentin beams from each group were immersed in 50 wt% ammoniacal silver nitrate for 24 h. They were then photo-developed for 8 h under a fluorescent light to reduce the silver ion. The beams were fixed for 2 h, and double stained with 1% uranyl acetate and 1% lead oxalate.
TEM specimens were prepared by the dual-beam focused ion beam (DB-FIB, FEI Nova-200 NanoLab, USA) which contained a gallium ion beam and a conventional field-emission SEM. The specimens were platinum-sputtered to enhance conductivity. To avoid electron charging during the milling process, a carbon thin film was sputtered onto the top of the section. The ion beam was operated at 5 kV to cut two parallel 15 μm long slots across the resin-dentin interfaces. A microplate was formed and trimmed to be 100 nm thick by gradually reducing the beam currents from 20,000 to 100 pA ( Fig. 1 ). The finished specimen was attached to a carbon-coated copper grid for TEM (JEOL HR-TEM 2100F Electron Microscope, Japan) examination. Nanoleakage was observed as the degree of infiltrated tracer silver grains. The thickness of hybrid layer was also measured on the TEM images.
To examine the orientation of collagen fibrils, the method by Lai et al. to quantify the alignment of fibers in tissue scaffold was used. The collagen matrix in the top of hybrid layer was defined as the region of interest to conduct a two-dimensional fast Fourier transform (FFT) analysis with ImageJ (National Institutes of Health) software. The spatial distribution of pixel intensity was translated into a plot of frequency domain. A graphical depiction of a circular projection on the FFT output image conducted a radial summation of the pixel intensities for each degree between 0° and 360°. The degree of anisotropy of the collagen fibrils was determined by the FFT plot since dual resonant peaks of a 180° period suggest a higher degree of similar fibril alignment whereas multiple broader peaks represent the anisotropy of the matrix.
2
Materials and methods
The study protocol was approved by the Institutional Review Board, National Cheng Kung University Hospital. Twenty sound extracted human molars were stored in 4 °C normal saline containing 0.02% sodium azide until use. These teeth were prepared for the nanoindentation and the microtensile bond strength (μTBS) tests.
2.1
Gel electrophoresis analysis
The cross-linking effect of RF/UVA on type I collagen was examined by sodium dodecyl sulfate–polyacrylamide gel electrophoresis (SDS-PAGE) . The test cross-linkers were 0.1% and 1% riboflavin-5′-phosphate (Sigma–Aldrich, St. Louis, MO, USA), which were separately added to 1.5 mg/ml bovine collagen solution (Sigma–Aldrich) in equal amounts. Collagen mixed with distilled water worked as the negative control. 5% GA (GlumaDesensitizer, Heraeus Kulzer, South Bend, IN, USA) and its diluent 0.5% GA were used as the positive control. The RF-collagen mixtures were irradiated under UVA light (Bio-LX E-365, 6 × 8 W, 365 nm, Vilber Lourmat, Marne-la-Vallée, France). The different riboflavin/UVA combinations to test were: 0.1% RF treatment followed by 1 min UVA irradiation, R0.1U1; 0.1% RF treatment followed by 2 min irradiation, R0.1U2; and 1% RF treatment followed by 1 min irradiation, R1U1.
Samples were diluted with sample buffer. After heat denaturation at 100 °C for 15 min, the sample was loaded onto an SDS-8% polyacrylamide separating gel with an SDS-4.5% stacking gel on top. Electrophoresis was carried out at 100 V Subsequently, the gels were stained with 0.1% Coomassie blue and photographed. Prestained molecular mass marker (Spectra Multicolour Broad Range Protein Ladder, Fermentas, Hanover, MD, USA) was run in a parallel lane as standard.
2.2
Nanoindentation test
For the nanoindentation tests, five 3 mm thick coronal dentin disks were prepared by cross-sectioning two molar teeth using a slow speed rotary saw (Isomet 2000; Buehler, Lake Bluff, IL, USA). The disks were embedded in epoxy resin, polished with abrasive papers (Nihon Kenshi, Osaka, Japan) to 1500 grit, and then by diamond suspensions to 1 μm. The dentin surface was divided into five tiny zones with area of 12–15 mm 2 , to receive various treatments: distilled water (as the control), R0.1U1, R0.1U2, R1U1, and GA. After acid-etching with 35% phosphoric acid (Ultraetch; Ultradent, Salt city, UT, USA) for 1 min, the experimental agents were applied on their respective zones by microbrushes (Benda MicroFine Applicators, Centrix, Santa Maria, CA, USA). The GA zone was treated with 5% GA (Gluma Desensitizer) for 1 min. The R0.1U2 zone was treated and irradiated under the UVA light for 1 min prior to the R0.1U1 and R1U1 zones.
The nanoindentation tests were conducted on a computer-controlled nanoindenter TI 700 UBI (Hysitron, MN, USA). The measurement was performed using a Vickers diamond indenter with a 50 nm radius, with a maximum load of 1000 μN at a load speed 50 μN/s. During indentation, the applied load and displacement were continuously recorded to derive the elastic modulus and nanohardness. For each group, at least twenty indentations were performed on the dentin disks in the dry condition. According to Kinney et al. , the nanoindentation test of the demineralized dentin in the hydrated state facilitates to determine the mechanical property of dentinal collagen matrix. Therefore, the same indentation test was also conducted after water-immersion for 30 min. The results were analyzed using the one-way ANOVA and post hoc Tukey tests for the differences among treatments at a 5% significance level. The differences in elastic modulus and microhardness under wet and dry conditions were analyzed by the t tests.
2.3
μTBS
Fifteen teeth were cut to expose coronal dentin, and then ground flat. The dentin surfaces were acid-etched for 15 s, water-rinsed, and then gently dried to prevent dehydration. The teeth were distributed into five treatment groups. The control received distilled water treatment for 1 min. The GA group was pretreated with 5% GA (Gluma Desensitizer) for 1 min. R0.1U1 and R0.1U2 were pretreated with 0.1% RF, and then UVA-irradiated for 1 or 2 min, respectively. R1U1 was treated with 1% RF and irradiated for 1 min. Subsequently, the dentin surface was treated with the Scotchbond Multi-purpose primer and adhesive (3M/ESPE, St. Paul, MN, USA) following the manufacturer’s instructions, and light cured for 20 s (Optilux 501; KerrHawe, Boggio, Switzerland). A microhybrid composite Z250 (3M/ESPE) was used to build up a 4 mm thick block in two increments, each light-cured for 40 s. After storage for 24 h in 37 °C distilled water, restored teeth were sectioned perpendicular to the bonded interface to generate 0.9 mm × 0.9 mm resin-dentin beams ( n = 24). Half of the resin-dentin beams received the μTBS test, while another half was further subject to 5000 thermocycles (5–60 °C) before the test. In the μTBS test, each beam was fixed to a jig on a universal testing machine (AG-1; Shimadzu, Kyoto, Japan), and stressed under tension at a crosshead speed of 1 mm/min until failure. μTBS was calculated by dividing the failure load by the precise cross-sectional area at the fracture site. Statistical analysis was performed by one-way ANOVA and Tukey tests.
To study the fracture patterns, these beams were mounted on aluminum stubs and gold sputtered. The fracture surfaces were examined with a scanning electron microscope (SEM) (JSM-6390 LV; JEOL, Tokyo, Japan) at 100× magnification. The fracture patterns were classified as: pre-test failure, adhesive failure, mixed adhesive and cohesive fracture, cohesive dentin fracture, and cohesive composite fracture. Data were analyzed using chi-square and Fisher’s exact tests.
2.4
Evaluation of nanoleakage and morphology of hybrid layer
Six resin-dentin beams from each group were immersed in 50 wt% ammoniacal silver nitrate for 24 h. They were then photo-developed for 8 h under a fluorescent light to reduce the silver ion. The beams were fixed for 2 h, and double stained with 1% uranyl acetate and 1% lead oxalate.
TEM specimens were prepared by the dual-beam focused ion beam (DB-FIB, FEI Nova-200 NanoLab, USA) which contained a gallium ion beam and a conventional field-emission SEM. The specimens were platinum-sputtered to enhance conductivity. To avoid electron charging during the milling process, a carbon thin film was sputtered onto the top of the section. The ion beam was operated at 5 kV to cut two parallel 15 μm long slots across the resin-dentin interfaces. A microplate was formed and trimmed to be 100 nm thick by gradually reducing the beam currents from 20,000 to 100 pA ( Fig. 1 ). The finished specimen was attached to a carbon-coated copper grid for TEM (JEOL HR-TEM 2100F Electron Microscope, Japan) examination. Nanoleakage was observed as the degree of infiltrated tracer silver grains. The thickness of hybrid layer was also measured on the TEM images.
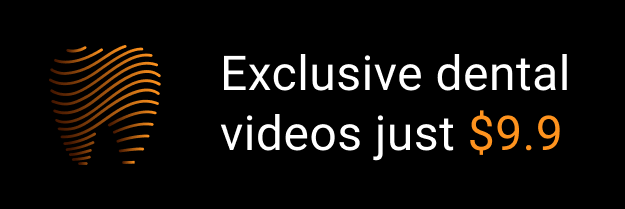