Abstract
Objectives
After selective excavation, bacteria are sealed beneath restorations. Leaking restorations could maintain carbohydrate-supply, leading to lesion progression and pulp damage. It is unclear if fluid (and thereby carbohydrate) exchange occurs through any interfacial gaps, or if such exchange only occurs in case gaps exceed a certain threshold size. We investigated how different restoration gap sizes impact on survival of sealed bacterial in vitro.
Methods
Bacterially contaminated artificial residual lesions were induced on the pulpo-axial walls of standardized dentin micro-cavities using acetic-acid demineralization and a continuous-culture Lactobacillus rhamnosus biofilm-model. Adhesive restorations with different gap sizes (0/100/200/400 μm) were placed (n = 24/group). Restorations were submitted to cyclic loading (42 g/0.2 Hz) under highly cariogenic conditions in a mastication-simulating artificial mouth. After 25 days, the number of sealed viable bacteria was determined as colony-forming units.
Results
After 25 days, CFU were significantly reduced in all groups (−99.99%, p < 0.001/Mann-Whitney). Significantly more viable bacteria remained in restorations with gaps ≥200 μm (p < 0.001).
Conclusions
Restoration gaps needed to exceed a threshold size to impede lesion arrest in vitro. There is great need to better understand why such threshold exists and which factors (mastication forces, restoration material, lesion location) could moderate the observed association.
Clinical significance
A certain gap sizes was necessary to allow sufficient fluid exchange for bacterial survival in vitro. It is not possible to deduct clinical recommendations at present.
1
Introduction
For deep lesions in teeth with vital, asymptomatic pulps, selective excavation is recommended, resulting in bacterially-contaminated dentin being sealed beneath restorations. Sealed bacteria are thought to inactivate due to nutritional deprivation under the placed restoration . Such lesion arrest might be impeded by restoration leakage, i.e. interfacial gaps allowing fluid exchange between lesions and the oral cavity. By such exchange, fermentable carbohydrates might be transported into the lesions, allowing re-activation of bacteria and subsequently leading to lesion progression and pulpal damage. Leakage could occur in case of imperfect seal, for example in case of moisture control not being fully successful during bonding .
It remains unclear if any degree of leakage (i.e. any kind of interfacial gap size) allows a sufficient fluid exchange for bacteria to survive. This fluid exchange could be further moderated by mastication forces: A “pumping” effect has been found to affect fluid transport along interfacial gaps, facilitating for example the induction of secondary carious lesions adjacent to small gaps, which were otherwise not associated with mineral loss if no such loading was provided .
The clinical exploration of these effects is ethically difficult, and in situ models have so far not been employed for loading simulations. Thus, in vitro models might be best suited to investigate the impact of different interfacial gap sizes on the inactivation of dentinal bacteria by sealing. We aimed to study these effects using a novel mastication-simulating artificial mouth model. We hypothesized that with increasing interfacial gap sizes, the number of remaining viable bacteria sealed under adhesive restorations increases significantly.
2
Materials and methods
2.1
Specimen preparation
Extracted bovine incisors without any caries lesions were obtained, cleaned, and L-shaped dentin blocks ( Fig. 1 ) prepared (Band Saw 300cl, Exakt Apparatebau, Norderstedt, Germany, and ceramic burs 806314, Hager & Meisinger, Neuss, Germany) and polished (Mikroschleifsystem 400CS, abrasive Paper 1200, 2400 and 4000). Specimens were covered with acid-resistant nail varnish (Rival de Loop, Rossmann, Hannover, Germany) except a window (2 × 2 mm) on the simulated pulpo-proximal wall, which was left uncovered ( Fig. 1 ). Artificial residual caries lesions were created in the unprotected area by storing the specimens in 1 l of a demineralizing solution (pH 5.0) containing 50 mM acetic acid, 3 mM CaCl 2 × H 2 O, 3 mM KH 2 PO 2 and 6 mM methyl-hydroxydiphosphonate for 2 days. The pH of the solution was monitored daily (InLab micro, Mettler-Toledo, Giessen, Germany) and if necessary adjusted using HCl (Roth, Karlsruhe, Germany) or 10 M KOH. Specimens were sterilized at 121 °C, 2.1 bar for 34 min, and then submitted to a computer-controlled continuous-culture biofilm model : Overnight cultures of Lactobacillus rhamnosus (DSM 20247, DSMZ, Braunschweig, Germany) were prepared in deMan-Rogosa-Sharpe (MRS) medium (Roth, Germany) at 37 °C. Specimens and bacterial suspension were incubated within the biofilm chamber at 100% humidity at 37 °C for 30 min, after which 15 ml MRS supplemented with 2% sucrose (MRS-S) was provided over 10 min using peristaltic multi-canal pumps (8152 Standard, MCP, Glattbrugg, Germany). Specimens were then allowed to rest for 120 min. This cycle was repeated for 2 days.

2.2
Restoration and gap simulation
Biofilms were now removed using a sterile swap. Nail varnish was removed except on the outer surfaces, and a self-etching adhesive (Scotchbond Universal, 3 M Espe, St. Paul, USA) used to condition the L-shaped cavity surface, not touching the lesion area. The adhesive layer was placed according to manufacturer’s recommendations and light-cured for 10 s using an LED curing light (Valo, Ultradent, Salt Lake City, USA) with an intensity of 1400 mW/cm 2 . The buccal and oral wall of each cavity was then restored with two pre-polymerized cubicles of Tetric EvoCeram resin composite (Ivoclar Vivadent, Schaan, Liechtenstein), which were cut from composite rods. Rods had been standardized via polyvinyl-siloxane moulds (Silagum, DMG, Hamburg, Germany), with plan-parallelized and polished surfaces. Blocks had been light-cured for 40 s as described. Cubicles were bonded to the dentin using Tetric EvoFlow, which was light-cured for 20 s ( Fig. 1 a).
On the simulated cervical surface and in continuation of the induced artificial lesion, different restoration gaps sizes were simulated using standardized copper foils (Gemmelmetalle, Berlin, Germany): 0, 100, 200 and 400 μm. Tetric EvoCeram was incrementally placed into the cavity to restore it, with each increment being light-cured as described. The foils could be completely removed afterwards. Gap size height and continuity of gaps were controlled on a pilot of 4 samples per gap size (total n = 16) as follows. Restored samples were twice cut sagittally (from the outer surface to the lesion), resulting in two discs, which were inspected at 2.5× magnification in a stereo microscope (Stemi SV11, Zeiss, Oberhausen, Germany) and digitally analyzed (AxioCam, Zeiss, Jena, Germany). The smallest gaps size in each sagittal section was measured. We found 100 μm foils to result in mean (range) continuous gaps of 121 (108–129) μm. Respective results for 200 μm were 220 (180–248) μm, those for 400 μm were 450 (440–490) μm. Note that for clarity purposes, groups are labelled as 100, 200 and 400 μm ( Fig. 1 b).
2.3
Mastication-simulating artificial mouth
Specimens were then mounted in one of four custom-made load-distribution discs ( Fig. 1 c and d), which allowed loading a total of 96 specimens in a chewing simulator (Kausimulator CS-4.8, Willytech, Feldkirchen-Westerham, Germany). Mounting was performed using dual-curing resin composite (Luxacore, DMG, Hamburg, Germany). Effect of load on gap size along the restoration had been tested a priori. The mean gap size reduction at the outer restoration surface was found to be 10% (range: 9–13%) in all groups at a load of 1 kg. As we neither aimed to fully compress gaps nor to change gap size by fatigue (as is usually done by mechanical cycling), this low-level load was applied in low frequency (0.2 Hz, 6 s loading time) at minimum loading speed. We had found gap sizes to not change significantly over 25 days with such loading in a pilot study (i.e. restorations were not significantly “aged” by repeated loading).
The load was applied onto the distribution discs, each of which transformed loads onto the restorations via 24 stainless steel metal pins (0.5 mm diameter). Loading was performed centrally onto the composite “restoration”. Precise loading was ensured by two-dimensional positioning of the movable pins ( Fig. 1 d). As 24 samples were loaded per disc, effective load was 42 g per sample. Note that this estimate was calculated, not measured. Given that both pins and the distributional discs were relatively rigid, we do not assume system-elasticity to introduce great variation in effective load per sample under the applied low-level loading. However, individual mounting might well lead to minimal deviations, which should be taken into account when interpreting our findings. After mounting specimens, a highly cariogenic artificial mouth was simulated: Within a closed chamber, 100% humidity and 37 °C were maintained, while specimens were submitted to sterile saline supplemented with 2% saccharose. Medium was replaced daily. After 25 days, specimens were removed, and restorations carefully lifted off the L-shaped samples using controlled force, while not touching the simulated lesion area.
2.4
Evaluation
Bacteria-contaminated dentin was now excavated from the lesion area using a rose head bur excavator (Hager & Meisinger) and the net weight of excavated dentin assessed (Analytical Plus, Ohaus, Nänikon, Switzerland). Dentin was then transferred to 0.9% sodium chloride, the medium serially diluted from 10 −1 and 10 −3 , and plated onto MRS agar for enumeration of colony-forming units (CFU) per mg dentin after 48 h.
2.5
Statistical analysis
Statistical analysis was performed using SPSS 20 (IBM, Armonk, USA). Data was controlled for normal distribution using Shapiro-Wilk-test. CFU in different groups were compared using Mann-Whitney- U test. Level of significance was set at p < 0.05 and tests were performed two-sided.
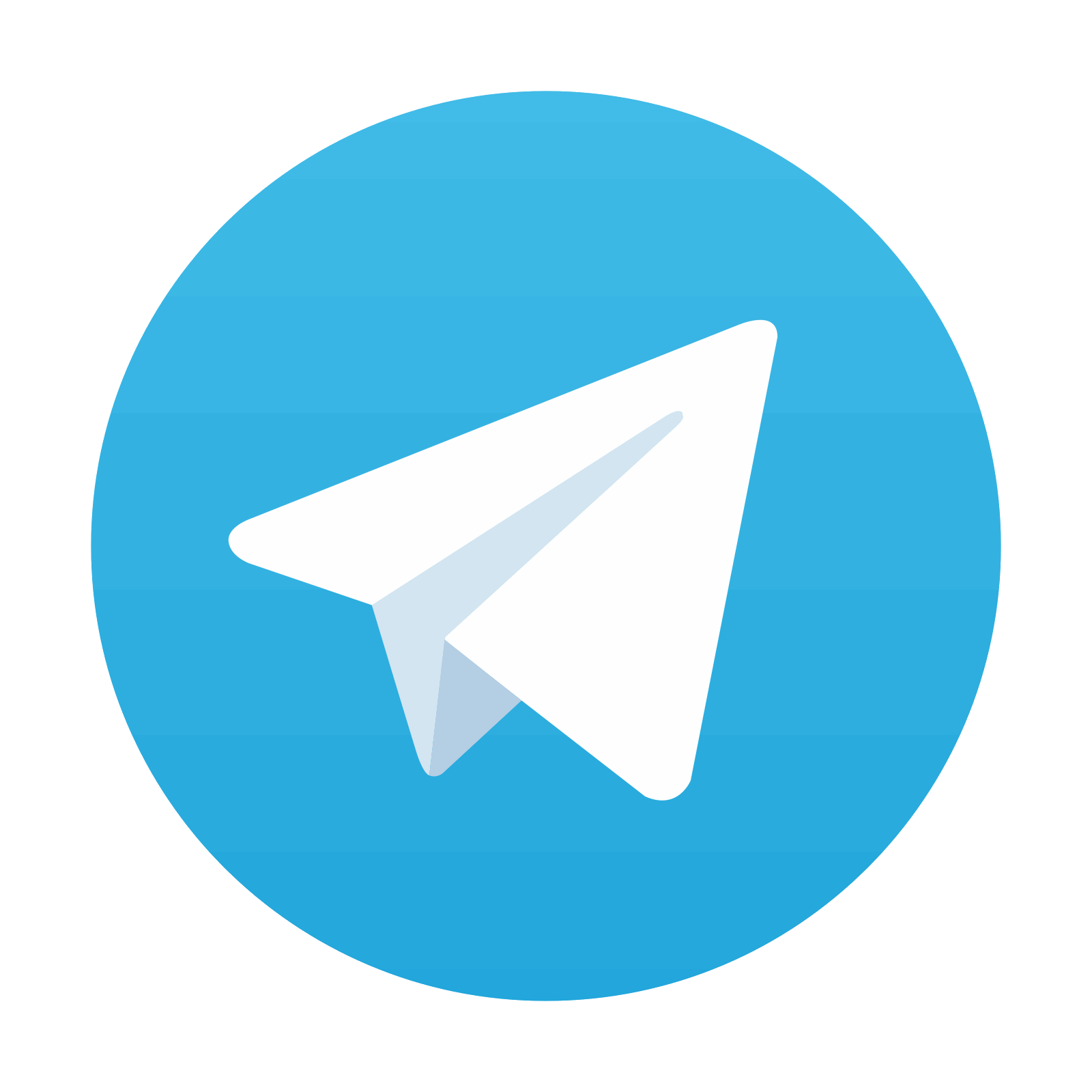
Stay updated, free dental videos. Join our Telegram channel

VIDEdental - Online dental courses
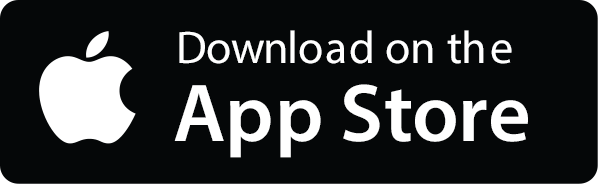
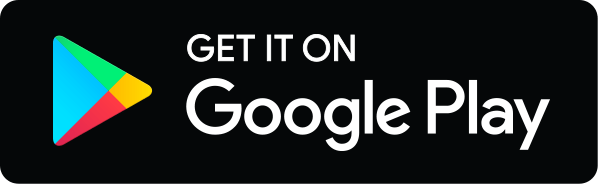