Abstract
Objectives: The objective is to review the current state of the art of dental composite materials. Methods: An outline of the most important aspects of dental composites was created, and a subsequent literature search for articles related to their formulation, properties and clinical considerations was conducted using PubMed followed by hand searching citations from relevant articles. Results: The current state of the art of dental composites includes a wide variety of materials with a broad range of mechanical properties, handling characteristics, and esthetic possibilities. This highly competitive market continues to evolve, with the major emphasis in the past being to produce materials with adequate strength, and high wear resistance and polishability retention. The more recent research and development efforts have addressed the issue of polymerization shrinkage and its accompanying stress, which may have a deleterious effect on the composite/tooth interfacial bond. Current efforts are focused on the delivery of materials with potentially therapeutic benefits and self-adhesive properties, the latter leading to truly simplified placement in the mouth. Significance: There is no one ideal material available to the clinician, but the commercial materials that comprise the current armamentarium are of high quality and when used appropriately, have proven to deliver excellent clinical outcomes of adequate longevity.
1
Introduction
The composition of resin-based dental composites has evolved significantly since the materials were first introduced to dentistry more than 50 years ago ( Fig. 1 ). Until recently, the most important changes have involved the reinforcing filler, which has been purposely reduced in size to produce materials that are more easily and effectively polished and demonstrate greater wear resistance. The latter was especially necessary for materials used in posterior applications, but the former has been important for restorations in all areas of the mouth. Current changes are more focused on the polymeric matrix of the material, principally to develop systems with reduced polymerization shrinkage, and perhaps more importantly, reduced polymerization shrinkage stress, and to make them self adhesive to tooth structure. Several articles recently have reviewed the current technology of dental composites and described future developments, such as self-repairing and stimuli-responsive materials . The current review will provide a brief historical perspective on dental resin composites to serve as a framework for a treatise on the current state of the art, primarily concentrating on work published in the past 5 years.

Resin composites are used for a variety of applications in dentistry, including but not limited to restorative materials, cavity liners, pit and fissure sealants, cores and buildups, inlays, onlays, crowns, provisional restorations, cements for single or multiple tooth prostheses and orthodontic devices, endodontic sealers, and root canal posts. It is likely that the use of these materials will continue to grow both in frequency and application due to their versatility. The rapidity by which the materials have evolved suggests a constantly changing state of the art.
The state of the art is defined as “the level of development (as of a device, procedure, process, technique, or science) reached at any particular time usually as a result of modern methods.” (Mirriam Webster Dictionary) When discussing patentable ideas, this has been more precisely defined (European Patent Convention) as “[the] state of the art shall be held to comprise everything made available to the public by means of a written or oral description, by use, or in any other way, before the date of filing of the European patent application.” Thus an idea may be based on prior art, but only is considered to be new if it does not form part of the current state of the art. Thus, the state of the art is constantly in flux. Further, the state of the art is usually distinguishable from what might be termed the “standard of care,” or the material/technique that generally has been adopted by the profession for a specific purpose.
This difference between state of the art and standard of care is made more evident when one examines the development of dental composites and follows the path of a new material ( Fig. 2 ). When a new dental composite material is conceived by an individual or a company, a patent application is typically filed to protect the concept. At the same time, or at some time in the future, the concept is reduced to practice, providing a material that has application for a specific purpose or set of purposes. The material comprises a portion of the current state of the art by virtue of its publication or presentation to the profession. However, the material must proceed through a more elaborate path to be considered a part of the current standard of care. In the ideal process, this requires a demonstration that the material is clinically efficacious. In other words, it must be shown to be used successfully in a controlled situation, such as a clinical trial. However, as has been seen many times, there is no guarantee that such a material will be shown also to be clinically effective when provided to all general practitioners for general use.

A material cannot achieve the level of standard of care, as defined by “the degree of care or competence that one is expected to exercise in a particular circumstance or role,” (Merriam-Webster’s Dictionary of Law) until it has been broadly accepted by the profession for its intended purpose(s). If efficacy and/or effectiveness cannot be proven for the new composite, then it may simply fade into history as many “other good ideas.” If the material does prove to be effective, it may still not become the standard of care, perhaps because there are better options available that are easier to use, of lower cost, or have some other benefit. The material may simply then become a useful adjunct to the current standard of care. The standard of care is also fluid, and a material that was the standard may simply with time relapse to becoming a useful adjunct or just another good idea. Thus, the state of the art and the standard of care are likely to be very different. This is emphasized by the fact that the time from which a new idea/practice option is introduced to when it becomes accepted by the majority of dental practitioners may be more than 10 years .
Describing the state of the art in dental composites requires a discussion of the formulation of current materials and the potential for future developments, the properties and limitations of the currently marketed products, and the important considerations for their clinical use. This manuscript will discuss each of these issues.
2
Dental composite formulation
2.1
Types of dental composites and their development
Dental composites can be distinguished by differences in formulation tailored to their particular requirements as restoratives, sealants, cements, provisional materials, etc. These materials are similar in that they are all composed of a polymeric matrix, typically a dimethacrylate, reinforcing fillers, typically made from radiopaque glass, a silane coupling agent for binding the filler to the matrix, and chemicals that promote or modulate the polymerization reaction. The many types of fillers in use recently have been reviewed . The predominant base monomer used in commercial dental composites has been bis-GMA, which due to its high viscosity is mixed with other dimethacrylates, such as TEGDMA, UDMA or other monomers . Some of these monomers, or modified versions of them, also serve as base monomers in many commercial materials. While there have been attempts to develop different polymerization promoting systems, most composites are light-activated, either as the sole polymerization initiator or in a dual cure formulation containing a chemically cured component. The most common photoinitiator system is camphoroquinone, accelerated by a tertiary amine, typically an aromatic one . Some commercial formulations have included other photoinitiators, such as PPD (1-phenyl-1,2-propanedione) , Lucirin TPO (monoacylphosphine oxide), and Irgacure 819 (bisacylphosphine oxide) , which are less yellow than CQ and thus potentially more color stable. Additional photoinitiators, such as OPPI (p-octyloxy-phenyl-phenyl iodonium hexafluoroantimonate) have been proposed based on encouraging experimental results .
The different types of composite materials are distinguished by their consistency. The universal restorative capable of being placed with a syringe or instrument may have a variety of consistencies depending upon its formulation. These materials are distinguished from the flowable composites, designed to be dispensed from very fine bore syringes into tight spaces for enhanced adaptation, and from the packable composites, designed to provide significant resistance to an amalgam condenser or other instrument in order to avoid slumping and to enhance the formation of tight interproximal contacts. Flowable composites are typically produced with a lower viscosity by reducing the filler content of the mixture, or by adding other modifying agents, such as surfactants, which enhance the fluidity while avoiding a large reduction in filler content that would significantly reduce mechanical properties and increase shrinkage . Packable composites achieve their thicker consistency through modification of the filler size distributions or through the addition of other types of particles, such as fibers, but generally not by increasing overall filler level .
Within each type of composite, the materials are further distinguished by the characteristics of their reinforcing fillers, and in particular their size ( Fig. 3 ). Conventional dental composites had average particle sizes that far exceeded 1 μm, and typically had fillers close to or exceeding the diameter of a human hair (∼50 μm). These “macrofill” materials were very strong, but difficult to polish and impossible to retain surface smoothness. To address the important issue of long-term esthetics, manufacturers began to formulate “microfill” composites, admittedly inappropriately named at the time, but probably done to emphasize the fact that the particles were “microscopic”. In truth, these materials were truly nanocomposites, as the average size of the amorphous spherical silica reinforcing particles was approximately 40 nm. The field of nanotechnology is defined at the nanoscale, and includes the 1–100 nm size range. Thus, the original “microfills” would have more accurately been called “nanofills”, but likely were not due to the lack of recognition of the concept of “nano” at the time. The filler level in these materials was low, but could be increased by incorporating highly filled, pre-polymerized resin fillers (PPRF) within the matrix to which additional “microfill” particles were added.
The “microfill composites were polishable but generally weak due to their relatively low filler content, and a compromise was needed to produce adequate strength with enhanced polishability and esthetics. Therefore, the particle size of the conventional composites was reduced through further grinding to produce what was ultimately called “small particle hybrid” composites. These were further distinguished as “midifills,” with average particle sizes slightly greater than 1 μm but also containing a portion of the 40 nm-sized fumed silica “microfillers.” Further refinements in the particle size through enhanced milling and grinding techniques resulted in composites with particles that were sub-micron, typically averaging about 0.4–1.0 μm, which initially were called “minifills” and ultimately came to be referred to as “microhybrids.” These materials are generally considered to be universal composites as they can be used for most anterior and posterior applications based on their combination of strength and polishability. The most recent innovation has been the development of the “nanofill” composites, containing only nanoscale particles. Most manufacturers have modified the formulations of their microhybrids to include more nanoparticles, and possibly pre-polymerized resin fillers, similar to those found in the microfill composites, and have named this group “nanohybrids.” In general, it is difficult to distinguish nanohybrids from microhybrids. Their properties, such as flexure strength and modulus, tend to be similar, with the nanohybrids as a group being in the lower range of the microhybrids, and both being greater than microfills . While some have shown evidence for reduced stability during water storage for nano-hybrid or nano-fill composites vs. microhybrids , others have shown an opposite trend or fairly similar susceptibility to aging . It has been suggested that the slightly lower properties of some nanohybrid composites may be due to the incorporation of pre-polymerized resin fillers . Regarding clinical evaluations, two recent studies over 2 and 4 years, respectively, showed similar excellent results in class II cavities for a nanofill vs. a microhybrid and nanohybrid vs. a microhybrid, with slight evidence for better marginal integrity for the micro-hybrid in the latter study .
2.2
Composition of current composites
The state of the art of the composition of dental composites has been changing rapidly in the past few years. The nanofill and nanohybrid materials represent the state of the art in terms of filler formulation . Comprehensive electron microscopy and elemental analysis has been performed on many current composites to verify the significant differences in filler composition, particle size and shape . New options for reinforcing fillers generally have focused on nano-sized materials and hybrid organic-inorganic fillers . Years ago, novel organically modified ceramics (ORMOCERS) were developed and have been used in commercial products. However, significant progress has been made in the development of new monomers for composite formulations with reduced polymerization shrinkage or shrinkage stress, as well as those with self-adhesive properties.
The epoxy-based silorane system used in Filtek Silorane LS (3 M ESPE) , provides verified lower shrinkage than typical dimethacrylate-based resins, likely due to the epoxide curing reaction that involves the opening of an oxirane ring. This commercial composite has been shown to have good mechanical properties . In one clinical study, the marginal quality of the silorane composite was shown to be somewhat inferior to that of a nanohybrid composite . Perhaps this is not surprising in that contraction stress, and not contraction itself, is considered to be the more important phenomena, and it has been shown that Silorane LS does not produce lower contraction stress than other composites . Others have experimented with other monomers, such as tetraoxaspiroundecane (TOSU), added to silorane systems and showed stress reduction, but the reduced stress may also be due in part to a reduction in mechanical properties .
Other monomers with increased molecular weight have been developed for composites with reduced shrinkage. The modified urethane dimethacrylate resin DX511 from Dupont found in Kalore (GC) is said to reduce shrinkage due to its relatively high molecular weight compared with bis-GMA and traditional UDMA (895 g/mole vs. 512 g/mole vs. 471 g/mole, respectively). The urethane monomer TCD-DI-HEA found in Venus Diamond (Kulzer) has been shown to produce lower polymerization contraction stress than other composites marketed as low-shrinking . The dimer acid monomers used in N’Durance (Septodont) are also of relatively high molecular weight, i.e. 673–849 g/mole, and have been shown to have high conversion of carbon double bonds while undergoing lower polymerization shrinkage than bis-GMA-based systems .
The latest trend has been toward the development of flowable composites containing adhesive monomers, such as Vertise Flow (Kerr) and Fusio Liquid Dentin (Pentron Clinical). These formulations are based on traditional methacrylate systems, but incorporating acidic monomers typically found in dentin bonding agents, such as glycerolphosphate dimethacrylate (GPDM) in Vertise Flow, which may be capable of generating adhesion through mechanical and possibly chemical interactions with tooth structure. These materials are currently recommended for liners and small restorations, and are serving as the entry point for universal self-adhesive composites.
2.3
Future developments
A recent review noted that efforts to modify fillers have been aimed at improving the properties of composites by the addition of polymer nonofibers, glass fibers, and titania nanoparticles . There is also very interesting work incorporating silsesquioxane nanocomposites which are essentially an organic–inorganic hybrid molecule that reduce shrinkage, but also reduce mechanical properties if used in too high of a concentration . Perhaps the most promising work in composites with modified fillers for both enhanced mechanical properties and remineralizing potential by virtue of calcium and phosphate release has been the work with fused silica whiskers and dicalcium or tetracalcium phosphate nanoparticles . These composites may be stronger and tougher, but the optical properties are not ideal and their opacity requires them to be self-cured or heat-processed at this point. Calcium fluoride containing fillers also have been added to filled dental resins and have shown high fluoride release and good mechanical properties . There are other monomers that are in various stages of development for potential use in dental composites, such as the (meth)acrylate vinyl ester hybrid polymerization system which exhibits phase separation during curing , thiolene monomers , multimethacrylate derivatives of bile acids, and others . It is expected that universal restorative materials based on the self-adhesive monomers being used or proposed in the flowable systems also will be forthcoming.
Other areas of development have included the incorporation of anti-bacterial agents and remineralizing agents into composites. Examples of compounds that have been added to resin composites to kill bacteria or inhibit biofilm formation include fluoride , chlorhexidine , zinc oxide nanoparticles , quaternary ammonium polyethyleneimine nanoparticles , and MDPB monomer . The effectiveness of the various fluoride-releasing restorative materials have been critically reviewed, and it was concluded that the clinical results are not conclusive for dental restorative materials, including composites . Remineralization may be promoted by the slow release of calcium and phosphate ions followed by the precipitation of new calcium-phosphate mineral . Years ago a material was developed which was purported to exhibit “smart release” of these ions as a result of an acidic challenge, as occurs during caries formation. This material, Ariston pHc, was not ultimately successful, in large part due to the fact that it absorbed too much water which affected its dimension and properties. But the idea of a “smart” material that reacts to its environment to release remineralizing ions or anti-microbial agents is attractive and still a focal point of research.
2
Dental composite formulation
2.1
Types of dental composites and their development
Dental composites can be distinguished by differences in formulation tailored to their particular requirements as restoratives, sealants, cements, provisional materials, etc. These materials are similar in that they are all composed of a polymeric matrix, typically a dimethacrylate, reinforcing fillers, typically made from radiopaque glass, a silane coupling agent for binding the filler to the matrix, and chemicals that promote or modulate the polymerization reaction. The many types of fillers in use recently have been reviewed . The predominant base monomer used in commercial dental composites has been bis-GMA, which due to its high viscosity is mixed with other dimethacrylates, such as TEGDMA, UDMA or other monomers . Some of these monomers, or modified versions of them, also serve as base monomers in many commercial materials. While there have been attempts to develop different polymerization promoting systems, most composites are light-activated, either as the sole polymerization initiator or in a dual cure formulation containing a chemically cured component. The most common photoinitiator system is camphoroquinone, accelerated by a tertiary amine, typically an aromatic one . Some commercial formulations have included other photoinitiators, such as PPD (1-phenyl-1,2-propanedione) , Lucirin TPO (monoacylphosphine oxide), and Irgacure 819 (bisacylphosphine oxide) , which are less yellow than CQ and thus potentially more color stable. Additional photoinitiators, such as OPPI (p-octyloxy-phenyl-phenyl iodonium hexafluoroantimonate) have been proposed based on encouraging experimental results .
The different types of composite materials are distinguished by their consistency. The universal restorative capable of being placed with a syringe or instrument may have a variety of consistencies depending upon its formulation. These materials are distinguished from the flowable composites, designed to be dispensed from very fine bore syringes into tight spaces for enhanced adaptation, and from the packable composites, designed to provide significant resistance to an amalgam condenser or other instrument in order to avoid slumping and to enhance the formation of tight interproximal contacts. Flowable composites are typically produced with a lower viscosity by reducing the filler content of the mixture, or by adding other modifying agents, such as surfactants, which enhance the fluidity while avoiding a large reduction in filler content that would significantly reduce mechanical properties and increase shrinkage . Packable composites achieve their thicker consistency through modification of the filler size distributions or through the addition of other types of particles, such as fibers, but generally not by increasing overall filler level .
Within each type of composite, the materials are further distinguished by the characteristics of their reinforcing fillers, and in particular their size ( Fig. 3 ). Conventional dental composites had average particle sizes that far exceeded 1 μm, and typically had fillers close to or exceeding the diameter of a human hair (∼50 μm). These “macrofill” materials were very strong, but difficult to polish and impossible to retain surface smoothness. To address the important issue of long-term esthetics, manufacturers began to formulate “microfill” composites, admittedly inappropriately named at the time, but probably done to emphasize the fact that the particles were “microscopic”. In truth, these materials were truly nanocomposites, as the average size of the amorphous spherical silica reinforcing particles was approximately 40 nm. The field of nanotechnology is defined at the nanoscale, and includes the 1–100 nm size range. Thus, the original “microfills” would have more accurately been called “nanofills”, but likely were not due to the lack of recognition of the concept of “nano” at the time. The filler level in these materials was low, but could be increased by incorporating highly filled, pre-polymerized resin fillers (PPRF) within the matrix to which additional “microfill” particles were added.
The “microfill composites were polishable but generally weak due to their relatively low filler content, and a compromise was needed to produce adequate strength with enhanced polishability and esthetics. Therefore, the particle size of the conventional composites was reduced through further grinding to produce what was ultimately called “small particle hybrid” composites. These were further distinguished as “midifills,” with average particle sizes slightly greater than 1 μm but also containing a portion of the 40 nm-sized fumed silica “microfillers.” Further refinements in the particle size through enhanced milling and grinding techniques resulted in composites with particles that were sub-micron, typically averaging about 0.4–1.0 μm, which initially were called “minifills” and ultimately came to be referred to as “microhybrids.” These materials are generally considered to be universal composites as they can be used for most anterior and posterior applications based on their combination of strength and polishability. The most recent innovation has been the development of the “nanofill” composites, containing only nanoscale particles. Most manufacturers have modified the formulations of their microhybrids to include more nanoparticles, and possibly pre-polymerized resin fillers, similar to those found in the microfill composites, and have named this group “nanohybrids.” In general, it is difficult to distinguish nanohybrids from microhybrids. Their properties, such as flexure strength and modulus, tend to be similar, with the nanohybrids as a group being in the lower range of the microhybrids, and both being greater than microfills . While some have shown evidence for reduced stability during water storage for nano-hybrid or nano-fill composites vs. microhybrids , others have shown an opposite trend or fairly similar susceptibility to aging . It has been suggested that the slightly lower properties of some nanohybrid composites may be due to the incorporation of pre-polymerized resin fillers . Regarding clinical evaluations, two recent studies over 2 and 4 years, respectively, showed similar excellent results in class II cavities for a nanofill vs. a microhybrid and nanohybrid vs. a microhybrid, with slight evidence for better marginal integrity for the micro-hybrid in the latter study .
2.2
Composition of current composites
The state of the art of the composition of dental composites has been changing rapidly in the past few years. The nanofill and nanohybrid materials represent the state of the art in terms of filler formulation . Comprehensive electron microscopy and elemental analysis has been performed on many current composites to verify the significant differences in filler composition, particle size and shape . New options for reinforcing fillers generally have focused on nano-sized materials and hybrid organic-inorganic fillers . Years ago, novel organically modified ceramics (ORMOCERS) were developed and have been used in commercial products. However, significant progress has been made in the development of new monomers for composite formulations with reduced polymerization shrinkage or shrinkage stress, as well as those with self-adhesive properties.
The epoxy-based silorane system used in Filtek Silorane LS (3 M ESPE) , provides verified lower shrinkage than typical dimethacrylate-based resins, likely due to the epoxide curing reaction that involves the opening of an oxirane ring. This commercial composite has been shown to have good mechanical properties . In one clinical study, the marginal quality of the silorane composite was shown to be somewhat inferior to that of a nanohybrid composite . Perhaps this is not surprising in that contraction stress, and not contraction itself, is considered to be the more important phenomena, and it has been shown that Silorane LS does not produce lower contraction stress than other composites . Others have experimented with other monomers, such as tetraoxaspiroundecane (TOSU), added to silorane systems and showed stress reduction, but the reduced stress may also be due in part to a reduction in mechanical properties .
Other monomers with increased molecular weight have been developed for composites with reduced shrinkage. The modified urethane dimethacrylate resin DX511 from Dupont found in Kalore (GC) is said to reduce shrinkage due to its relatively high molecular weight compared with bis-GMA and traditional UDMA (895 g/mole vs. 512 g/mole vs. 471 g/mole, respectively). The urethane monomer TCD-DI-HEA found in Venus Diamond (Kulzer) has been shown to produce lower polymerization contraction stress than other composites marketed as low-shrinking . The dimer acid monomers used in N’Durance (Septodont) are also of relatively high molecular weight, i.e. 673–849 g/mole, and have been shown to have high conversion of carbon double bonds while undergoing lower polymerization shrinkage than bis-GMA-based systems .
The latest trend has been toward the development of flowable composites containing adhesive monomers, such as Vertise Flow (Kerr) and Fusio Liquid Dentin (Pentron Clinical). These formulations are based on traditional methacrylate systems, but incorporating acidic monomers typically found in dentin bonding agents, such as glycerolphosphate dimethacrylate (GPDM) in Vertise Flow, which may be capable of generating adhesion through mechanical and possibly chemical interactions with tooth structure. These materials are currently recommended for liners and small restorations, and are serving as the entry point for universal self-adhesive composites.
2.3
Future developments
A recent review noted that efforts to modify fillers have been aimed at improving the properties of composites by the addition of polymer nonofibers, glass fibers, and titania nanoparticles . There is also very interesting work incorporating silsesquioxane nanocomposites which are essentially an organic–inorganic hybrid molecule that reduce shrinkage, but also reduce mechanical properties if used in too high of a concentration . Perhaps the most promising work in composites with modified fillers for both enhanced mechanical properties and remineralizing potential by virtue of calcium and phosphate release has been the work with fused silica whiskers and dicalcium or tetracalcium phosphate nanoparticles . These composites may be stronger and tougher, but the optical properties are not ideal and their opacity requires them to be self-cured or heat-processed at this point. Calcium fluoride containing fillers also have been added to filled dental resins and have shown high fluoride release and good mechanical properties . There are other monomers that are in various stages of development for potential use in dental composites, such as the (meth)acrylate vinyl ester hybrid polymerization system which exhibits phase separation during curing , thiolene monomers , multimethacrylate derivatives of bile acids, and others . It is expected that universal restorative materials based on the self-adhesive monomers being used or proposed in the flowable systems also will be forthcoming.
Other areas of development have included the incorporation of anti-bacterial agents and remineralizing agents into composites. Examples of compounds that have been added to resin composites to kill bacteria or inhibit biofilm formation include fluoride , chlorhexidine , zinc oxide nanoparticles , quaternary ammonium polyethyleneimine nanoparticles , and MDPB monomer . The effectiveness of the various fluoride-releasing restorative materials have been critically reviewed, and it was concluded that the clinical results are not conclusive for dental restorative materials, including composites . Remineralization may be promoted by the slow release of calcium and phosphate ions followed by the precipitation of new calcium-phosphate mineral . Years ago a material was developed which was purported to exhibit “smart release” of these ions as a result of an acidic challenge, as occurs during caries formation. This material, Ariston pHc, was not ultimately successful, in large part due to the fact that it absorbed too much water which affected its dimension and properties. But the idea of a “smart” material that reacts to its environment to release remineralizing ions or anti-microbial agents is attractive and still a focal point of research.
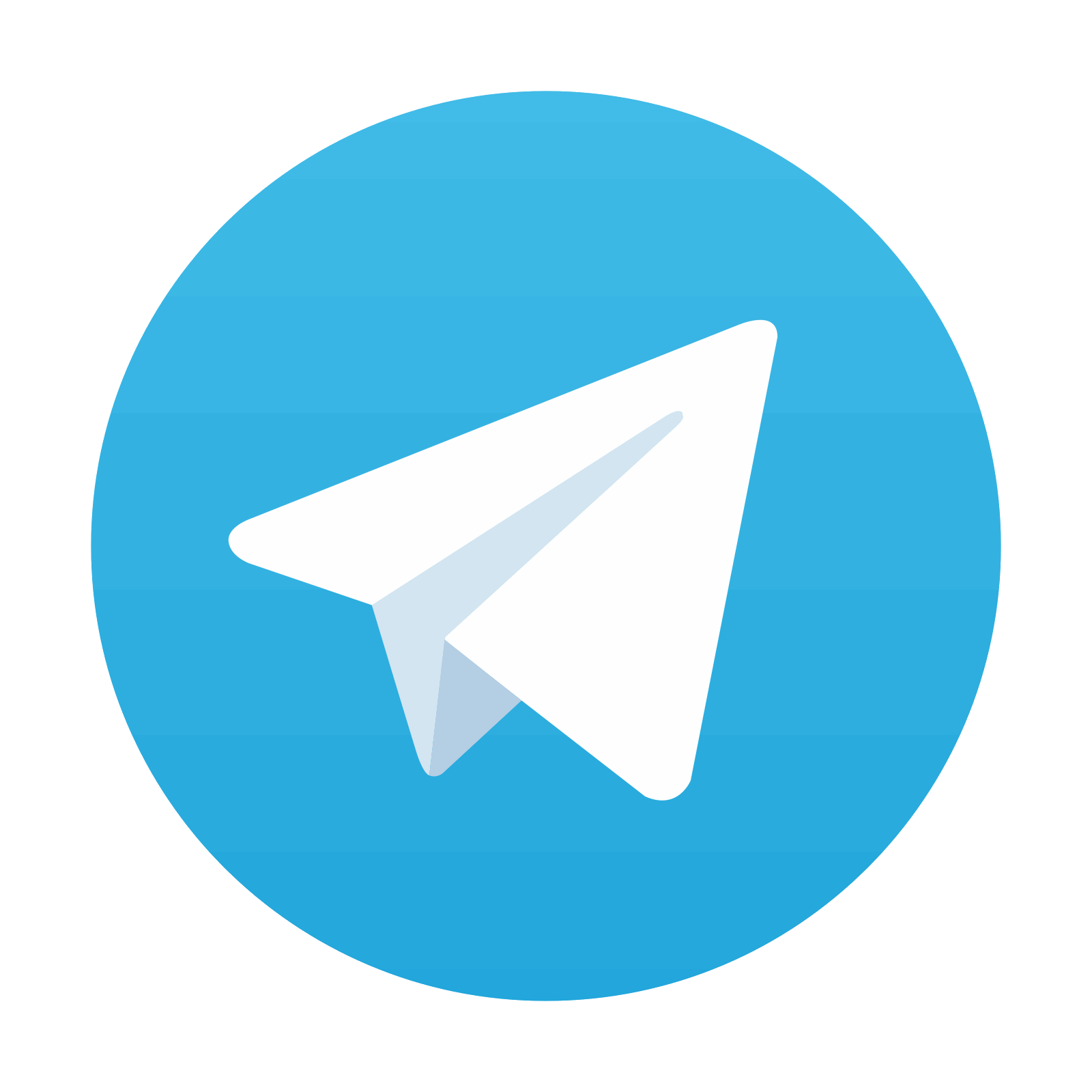
Stay updated, free dental videos. Join our Telegram channel

VIDEdental - Online dental courses
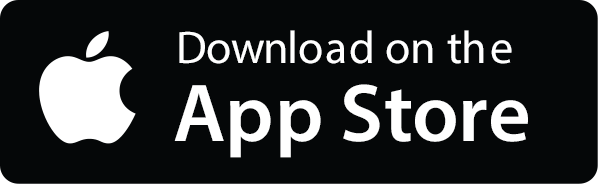
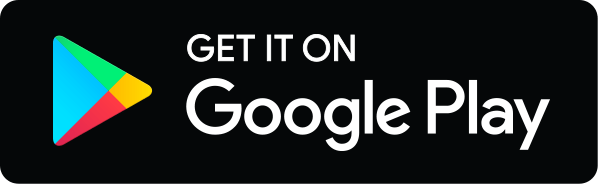