Abstract
Objectives
The use of thio-urethane oligomers has been shown to significantly improve the mechanical properties of resin cements (RCs). The aim of this study was to use thio-urethane-modified RC to potentially reinforce the porcelain-RC structure and to improve the bond strength to zirconia and lithium disilicate.
Methods
Six oligomers were synthesized by combining thiols – pentaerythritol tetra-3-mercaptopropionate (PETMP, P) or trimethylol-tris-3-mercaptopropionate (TMP, T) – with di-functional isocyanates – 1,6-Hexanediol-diissocyante (HDDI) (aliphatic, AL) or 1,3-bis(1-isocyanato-1-methylethyl)benzene (BDI) (aromatic, AR) or Dicyclohexylmethane 4,4′-Diisocyanate (HMDI) (cyclic, CC). Thio-urethanes (20 wt%) were added to a BisGMA/UDMA/TEGDMA organic matrix. Filler was introduced at 60 wt%. The microshear bond strength (μSBS), Weibull modulus ( m ), and failure pattern of RCs bonded to zirconia (ZR) and lithium disilicate (LD) ceramics was evaluated. Biaxial flexural test and fractographic analysis of porcelain discs bonded to RCs were also performed. The biaxial flexural strength ( σ bf ) and m were calculated in the tensile surfaces of porcelain and RC structures (Z = 0 and Z = −t 2 , respectively).
Results
The μSBS was improved with RCs formulated with oligomers P_AL or T_AL bonded to LD and P_AL, P_AR or T_CC bonded to zirconia in comparison to controls. Mixed failures predominated in all groups. σ bf had superior values at Z = 0 with RCs formulated with oligomers P_AL, P_AR, T_AL, or T_CC in comparison to control; σ bf increased with all RCs composed by thio-urethanes at Z = −t 2 . Fractographic analysis revealed all fracture origins at Z = 0.
Conclusion
The use of specific thio-urethane oligomers as components of RCs increased both the biaxial flexural strength of the porcelain-RC structure and the μSBS to LD and ZR.
Clinical significance
The current investigation suggests that it is possible to reinforce the porcelain-RC pair and obtain higher bond strength to LD and ZR with RCs formulated with selected types of thio-urethane oligomers.
1
Introduction
Resin cements (RCs) have gained clinical popularity in Dentistry because of their ability to bond both to the tooth structure and to the majority of restorative materials when associated with chemical components such as silanes and phosphate-based primers [ ]. The use of RCs is indicated for several clinical applications that include adhesion of complete crowns, fixed partial prosthesis, partial coverage, and intracanal posts. The main advantages of RCs over other traditionally used cements, such as zinc-phosphates and glass-ionomers, are the reduced risk of microleakage, and the decreased sorption, solubility, and staining, which results in the maintenance of the interface integrity [ ].
Glass-ceramics and polycrystalline ceramics are available for use in clinical practice. Loss of retention might represent a technical complication, compromising the lifetime of the restoration [ ]. Glass-ceramics have been widely used for years due to the possibility of producing total or partial coverage, maintaining the maximum integrity of the remaining coronal structure. Considering that glass-ceramic restorations have been extensively used in partial coverage with preparations that do not have sufficient mechanical retention, high bond strength values are important for restoration successful clinical outcome. However, the current bond protocols are not very efficient for polycrystalline ceramics, since they are acid resistant and therefore cannot be treated by conventional etching [ ]. Moreover, silane treatments are also not adequate for materials such as highly crystalline zirconia, which do not form covalent bonds with the alkoxy silane group of the coupling agent [ ]. This problem has led to extensive research in the recent years focusing on the improvement of bonding strategies for this class of ceramic material, which has been used mostly for full coverage. More recently, partial polycrystalline structures have also been recommended [ ], increasing the need for resin cements that provide higher bond strength and retention stability.
Besides providing bonding stability for ceramic restorations, RCs have been recognized to significantly strengthen bonded restoration [ ]. Studies have demonstrated that the increase in the elastic modulus of RCs has a favorable impact on the mechanical strength of a dental feldsphatic porcelain [ ]. Moreover, cement characteristics such as the reduced volumetric shrinkage and, as a consequence, low polymerization stress might have a positive impact on the ceramic strength [ ]. These cement characteristics are of great interest, especially for restorations made of dental porcelains or glass-ceramics. These materials provide excellent esthetic characteristics and have been used for years in different applications. However, they are intrinsically fragile structures, i.e., have very low fracture toughness in comparison to other dental ceramics.
Recent publications showed that RCs formulated with thio-urethane oligomers as part of the organic matrix presented increased mechanical properties when compared to materials composed only by traditional monomers. Mechanical properties such as flexural strength, flexural modulus, and fracture toughness are the ones mostly improved by the addition of thio-urethanes [ ]. These improvements are achieved because of a combination of factors, including the low Tg of the additive [ ], which provides opportunities for stress relaxation within the glassy methacrylate network [ ], but also possibly due to active strand behavior on the thio-urethane oligomer [ ]. In addition to that, the fact that the additive is pre-polymerized leads to a reduction in volumetric shrinkage and polymerization stress, observed in conjunction with an increase in the degree of conversion and delayed gelation/vitrification, which are in turn a function of the chain-transfer-capable thiol pending functionalities [ ].
The objective of the present study was to determine the bond strength of resin cements to ceramic materials as function of the cement formulation (six oligomers) and the ceramic substrate (zirconia or lithium disilicate). In addition, the Weibull parameter of bilayered discs (0.15 mm of cement and 0.8 mm of ceramic) was determined for porcelain/RC specimens using the six experimental cement compositions. The discs were tested with the cement layer positioned on the tensile side of the biaxial flexural strength test. The hypothesis was that the properties evaluated would be significantly improved by the formulation of RCs containing thio-urethanes.
2
Materials and methods
2.1
Resin cements composition
The experimental resin cements formulated for the study were composed of Bis-phenol A diglycidyl dimethacrylate (Bis-GMA; Esstech, Essington, PA, USA), urethane dimethacrylate (UDMA; Esstech) and tri-ethylene glycol dimethacrylate (TEGDMA; Esstech) in a 50:30:20 mass ratio. Photoinitiators were added to the monomers as follows: 0.6 wt% of a tertiary amine (EDMAB – ethyl 4-dimethylaminobenzoate; Avocado, Heysham, England), 0.2 wt% of dl-camphoroquinone (Polysciences Inc., Warrington, PA, USA), and 0.5 wt% inhibitor (BHT-2,6-di-tert-butyl-4-methylphenol; SigmaAldrich, St. Louis, MO, USA).
Six different oligomers were synthesized in solution in the presence of catalytic amounts of trimethylamine. Multi-functional thiols were combined with di-functional isocyanates in 4 × volume of dichloromethane (very diluted solution) as presented in Table 1 . In addition, the isocyanate:thiol ratio was kept at 1:2 (by mol) to avoid gelation of the oligomer during reaction, according to the Flory-Stockmeyer theory [ ], leaving pendant thiols. Oligomers were purified by precipitation in hexanes and rotaevaporation, and then characterized by 1 H NMR and mid-IR spectroscopy [ ]. The disappearance of the isocyanate peak at 2270 cm −1 and the appearance of resonance signals at 3.70 ppm were used as evidence for completion of isocyanate reaction and thio-urethane bond formation, respectively [ ]. The thiol group (SH) concentration for each oligomer was determined using a titration method with Ellman’s reagent well established in the literature [ ]. Thio-urethane oligomers were added to the methacrylate organic phase in proportion of 20 wt%, as defined in our previous investigation [ ]. The final oligomer product presented as a viscous liquid at room temperature, and was completely miscible with methacrylate monomers. A resin cement formulated without oligomer served as control.
Thiol (abbreviation) | Isocyanate (abbreviation) | Thio-urethane formed |
---|---|---|
Pentaerythritol tetra-3-mercaptopropionate (PETMP) | 1,6-Hexanediol-diissocyante (aliphatic, AL) | P_AL |
Pentaerythritol tetra-3-mercaptopropionate (PETMP) | 1,3-bis(1-isocyanato-1-methylethyl)benzene (aromatic, AR) | P_AR |
Pentaerythritol tetra-3-mercaptopropionate (PETMP) | Dicyclohexylmethane 4,4′-Diisocyanate (cyclic, CC) | P_CC |
Trimethylol-tris-3-mercaptopropionate (TMP) | 1,6-Hexanediol-diissocyante (aliphatic, AL) | T_AL |
Trimethylol-tris-3-mercaptopropionate (TMP) | 1,3-bis(1-isocyanato-1-methylethyl)benzene (aromatic, AR) | T_AR |
Trimethylol-tris-3-mercaptopropionate (TMP) | Dicyclohexylmethane 4,4′-Diisocyanate (cyclic, CC) | T_CC |
Filler was introduced to all groups at 60 wt% (Barium glass 0.7 μm, density 3.0 g/ml, refractive index 1.553 – V117 4107, Esstech), with the aid of a mechanical mixer (DAC 150 Speed mixer, Flacktek, Landrum, SC, USA) for 5 min at 2400 rpm. All procedures were carried out under yellow lights.
2.2
Microshear bond strength (μSBS) to ceramics
Forty-two specimens (14 mm long, 12 mm wide and 3 mm thick) of yttrium-stabilized tetragonal polycrystalline zirconia (Y-TZP) (inCoris TZI mono L, Sirona Dental Inc, USA) or lithium disilicate ceramic (LD) (IPS e.max CAD, HT, shade A1, Ivoclar Vivadent, Liechtenstein) were produced (n = 6 per group). Materials were processed as per the manufacturer’s instructions. After sintering, the Y-TZP bonding surface was sandblasted with 30 μm silica-coated aluminum oxide particles (CoJet™ Sand S30, 3 M ESPE, USA) for 15 s (at a distance of 5 mm and 2.5 bar of pressure) and ultrasonically cleaned for 10 min. Samples were silanated (Silano, Angelus, Brazil) and coated with a layer of 10-MDP (Metal/Zirconia Primer, Ivoclar Vivadent). LD specimens were etched with 10% hydrofluoric acid (Condac porcelain, FGM Dental Products, Brazil) for 20 s, cleaned with water and oil-free compressed air for 30 s and silanated (Silano, Angelus).
RC application on the ceramic treated surfaces was performed by filling plastic cylinders (0.8 mm wide and 1 mm long, Tygon ® Tubing, Bio-Rad Laboratories, USA) with RC – 04 RC cylinders for each ceramic specimen, totalizing 24 bonded cylinders in each group. The RC was light activated for 60 s at 800 mW/cm 2 (Bluephase, Ivoclar Vivadent). The plastic cylinders were removed after RC polymerization. Samples were water-stored for one week at a temperature of 37 ° C. Specimens were tested in a universal testing machine at a crosshead speed of 0.5 mm/min using a metal wire. Data were obtained in MPa by dividing the force in Newton (N) by the bonding surface area (A) (A = π* r 2 , where r is the RC cylinder radius = 0.4 mm). The mean fracture strength (MPa) in each specimen was calculated.
The fractured surfaces were evaluated by stereomicroscope (Olympus, Japan) and the failure patterns classified as adhesive, cohesive in cement, cohesive in ceramic, or mixed.
2.3
Porcelain-RC biaxial flexural strength
Two hundred and ten disc-shaped porcelain specimens were produced. The porcelain powder (Super Porcelain EX-3, Noritake, Japan) was mixed with the modeling liquid to produce a thick slurry, which was condensed into a metallic mold (12-mm diameter, 1-mm thickness). The mold was overfilled and placed on a vibrating table for 90 s, with excess liquid removed with absorbent paper. The surface was leveled with a razor blade to produce discs of uniform thickness. Each disc was removed carefully from the mold, placed on a refractory substrate, and fired in a ceramic furnace (Vacumat 40, Vita Zahnfabrik) according to the manufacturer’s instruction. The discs were cooled to room temperature and visually inspected. Discs with defects or visible cracks observed under a stereo microscope were discarded and replaced. The discs were manually wet ground on both sides with 320-grit SiC abrasive papers (Norton S.A., Brazil) to produce flat surfaces 0.8 ± 0.1 mm in thickness. Wet polishing was performed with 600- and 1200-grit SiC abrasive papers for 60 s on each surface. Final dimensions were checked with a digital caliper (Absolute Digimatic, Mitutoyo, Japan), and the porcelain discs were randomly divided into seven groups (n = 30 per group) according to the RC tested.
The bonding surface of each porcelain disc was etched with 10% hydrofluoric acid (Condac porcelain, FGM) for 60 s, washed for 30 s with water and oil-free compressed air for 30 s and silanated (Silano, Angelus). The resin cement coating was applied with the aid of a digital caliper were a cement layer of 150 μm was standardized [ ]. The RC was light activated with 800 mW/cm 2 (Bluephase, Ivoclar Vivadent) by 60 s through the porcelain disc. Samples were stored for 24 h in dark containers until final degree of conversion was obtained. The biaxial flexural strength ( σ bf ) of the porcelain-RC specimens was determined on the mechanical testing machine using a ball-on-ring setup. The discs were centrally placed on a 10-mm diameter knife-edged support and loaded with a spherical indenter (4-mm diameter) at a crosshead speed of 1 mm/min.
The σ bf was calculated according to the analytical solutions described and tested before [ ]. First, the E of the porcelain ( <SPAN role=presentation tabIndex=0 id=MathJax-Element-1-Frame class=MathJax style="POSITION: relative" data-mathml='E1*’>E∗1E1* E 1 * ) and RC ( <SPAN role=presentation tabIndex=0 id=MathJax-Element-2-Frame class=MathJax style="POSITION: relative" data-mathml='E2*’>E∗2E2* E 2 * ) were calculated as a function of the Poisson’s ratio of the porcelain and RC, according to Eq. (1) :
where E 1 is the E of porcelain [ ], and E 2 the E of the RC defined previously according to ISO 4049 [ ]. ν 1 and ν 2 the Poisson’s ratios of the porcelain (0.25) [ ] and resin-based luting agents (0.27) [ ]. The neutral plane ( tn ) of the coated porcelain specimens was calculated as a function of the porcelain and RC thicknesses ( t 1 and t 2 ) and the calculated <SPAN role=presentation tabIndex=0 id=MathJax-Element-4-Frame class=MathJax style="POSITION: relative" data-mathml='E1*’>E∗1E1* E 1 * and <SPAN role=presentation tabIndex=0 id=MathJax-Element-5-Frame class=MathJax style="POSITION: relative" data-mathml='E2*’>E∗2E2* E 2 * , using Eq. (2) :
The σ bf was calculated at z-axial positions at the center of the discs, where the porcelain surface at the bonded interface is located (position z = 0) and the RC surface that contacts the ring of the ball-on-ring setup is located (position z = −t 2 ), according to Eqs. (3) – (5) :
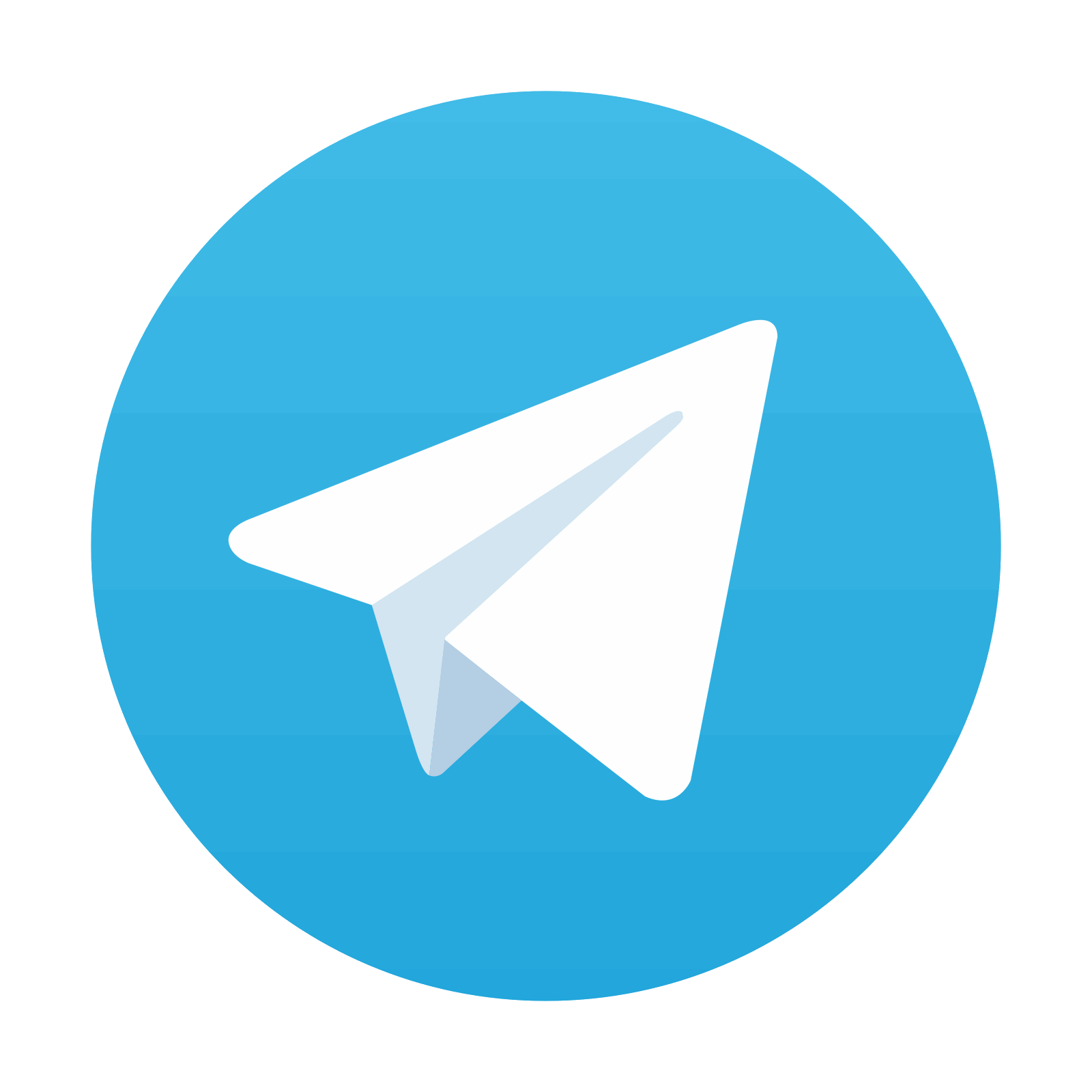
Stay updated, free dental videos. Join our Telegram channel

VIDEdental - Online dental courses
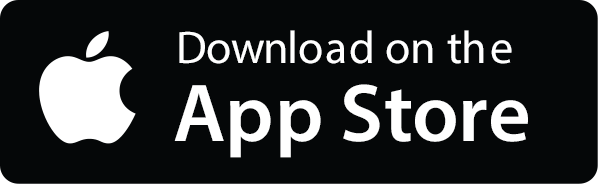
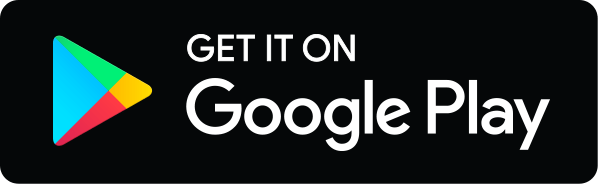