Abstract
Objectives
Aim of the study was the investigation of the repairability of a silorane (Filtek Silorane, 3M Espe, Seefeld, Germany) after different surface pretreatments in vitro .
Methods
54 silorane specimens (5 mm × 5 mm × 5 mm) were fabricated and stored in saline solution (24 h/37 °C). Their surface was polished with abrasive paper (600 grit), etched with phosphoric acid (10 s) and rinsed with water (30 s). Repair was performed with a silorane based on one of the 9 treatment protocols (each n = 6): no additional treatment (NAT), silorane primer (P) and silorane bond (B), B only, sandblasting (SB), SB plus P/B, SB plus B, CoJet and silane (CJ), CJ plus P/B, CJ plus B. Whole silorane specimens (5 mm × 5 mm × 10 mm) with no repair served as control. Specimens were sectioned and microtensile bond strength (μTBS) was measured (30 beams per group, surface area approx. 1.2 mm 2 , crosshead-speed 1 mm/min) statistical analysis (ANOVA, Tukey HSD, p < 0.05) was performed.
Results
μTBS of the specimens was significantly influenced by the surface pretreatment ( p < 0.001). The highest μTBS was determined for CJ/B and SB/B, which were not significantly different from the control. NAT, SB and CJ benefited from an additional treatment with B ( p < 0.01). The additional use of P did not improve μTBS, but was detrimental for the SB and CJ groups ( p < 0.05).
Significance
Siloranes can be repaired with either SB or CJ in combination with a silorane bond, the additional use of silorane primer is disadvantageous.
1
Introduction
Tooth colored composite materials gained a wide popularity during the last decades. Apart from the development of a minimal invasive preparation technique as well as improved adhesion to tooth structures these materials exhibit predictable long-term-stability with annual failure rates that are comparable for composites and amalgam in stress-bearing class I- and class II-cavities . Due to polymerization shrinkage of composite materials which leads to shrinkage stress at the adhesive interface, possible consequences like cusp-deflection and loss of marginal integrity occur resulting in fractures at the interface, marginal staining, microleakage and gap-formation .
In order to overcome those shortcomings, dental siloranes that consist of a new organic matrix (i.e. monomers with a ring-opening oxirane) were marketed in 2007 . The goal of developing siloranes was to create a material with reduced polymerization shrinkage and less polymerization stress . So far, reduction of polymerization shrinkage could only be achieved if for example high molecular monomers or larger amounts of filler particles were added to the organic matrix. Siloranes reveal shrinkage of approx. 1 vol% . In posterior mod-cavities the application of siloranes causes a significantly lower cusp deflection in comparison to methacrylate-based materials, which was attributed to the lower shrinkage values of this material . Regarding the effect of a cavity’s c-factor, the silorane material revealed equal μTBS-values on flat surfaces when compared to a conventional methacrylate based composite resin. In contrast, a bulk filling technique leads to significantly decreased μTBS-values for the silorane . Clinically, the use of an inappropriate layering technique could cause a failure of the restoration.
Silorane-based materials are not investigated widely in vivo due to their relative short existence on the dental market. One clinical trial that examined a methacrylate-based composite resin material and a silorane in class II-cavities revealed an inferior marginal adoption for the silorane compared to a methacrylate based material both occlusally and approximately . Although to our knowledge only one study with a limited observation time is published so far, questions arise regarding the long-term-stability of silorane-based materials.
The long-term-stability of dental restorations improved within the last years, but one of the main reasons for placing new restorations is the replacement of preexisting fillings, which is associated with the risk of sacrificing sound tooth structure . In order to preserve healthy dental hard tissues the repair of dental restorations has become an important treatment option in modern operative dentistry. The complete removal of a tooth colored restoration, i.e. glass-ceramic or composite, caused a loss of tooth structure that was twice as high compared to amalgam or glass-ionomer .
As a result, numerous repair modalities have been evaluated in vitro for conventional methacrylate-based composites: surface pretreatment with sandblasting, silica-coating and silanization, roughening of the surface with diamond burs or silicon carbide paper, use of phosphoric acid, different adhesive techniques and preparation methods . In class II-cavities with the composite surface pretreated with silicon-carbide-burs, best fracture-resistance was achieved after approximal repair for parallel box-preparations without undercuts . Regarding different repair procedures, the treatment of composite surfaces with silica-coating and a bonding agent yielded the highest shear bond strength among different repair procedures . 50-μm aluminium oxide air abrasion followed by 37% phosphoric acid etching and the application of a one-step total-etch adhesive resulted in the highest μTBS values for composite repair . Another recently published study also confirmed the superiority of sandblasting with aluminium oxide over surface roughening with a diamond bur which led to a lower μTBS . Minimal invasive interventions such as sealing of margins and repair of defective filling areas can improve the long-term-stability of resin-based composite materials after 2 years in vivo .
The repair of siloranes was not yet investigated in detail. If a silorane filling has to be repaired, no protocol about the surface pretreatment of these restorations exists so far. The pretreatment of the surface could be performed equivalent to the repair of methacrylate-based composite materials, as done by , where the surface was roughened with silicon-carbide paper, cleaned with 37% phosphoric acid and coated with the silorane bonding agent. The repair protocol for silorane class-V restorations described by included surface roughening with a diamond bur, etching of the cavity with 36% phosphoric acid and application of the complete silorane adhesive system.
Therefore, the aim of this study was to evaluate different pretreatment protocols for the repair of a dental silorane. The null hypothesis that was set forth was that different repair procedures do not influence the microtensile bond strength (μTBS) of a repaired silorane-based restoration.
2
Materials and methods
54 silorane specimens fabricated in Teflon moulds (5 mm × 5 mm × 5 mm) were used for these experiments. For each specimen, 2 layers of silorane were placed and cured separately. The last layer was covered with a transparent matrix in order to obtain a flat surface. Each layer (thickness max. 2.5 mm) was cured for 40 s with an LED polymerization light (Elipar Freelight 2, 3 M Espe, Seefeld, Germany) with an output of at least 1000 mW/cm 2 . The LED was placed directly at the surface of the mould. The output of the curing device was controlled during each polymerization circle with a digital radiometer (bluephase meter, Ivovlar Vivadent, Schaan, Liechtenstein). Afterwards, the specimens were stored in saline solution for 24 h at 37 °C. The surfaces of all specimens were wet-polished with abrasive paper (600 grit, Buehler GmbH, Düsserdorf, Germany) in order to remove the oxygen-inhibited layer, etched with phosphoric acid (30 s) and rinsed with water (30 s). All used materials, their manufacturers and the manufacturers’ instructions for use are shown in Table 1 .
Product | Manufacturer | Lot | Application |
---|---|---|---|
DeTrey ® Conditioner 36 | Dentsply, Konstanz, Germany | 0805002679 | Etching of the surface for 10 s, rinsing with water for 30 s, air-drying |
CoJet Sand | 3M Espe, Seefeld, Germany | 306931 | Applying particles with sandblaster for 10 s at a distance of 5 mm |
Monobond S | Ivoclar Vivadent, Schaan, Liechtenstein | 15382 | Applying, air-drying after 60 sec |
Aluminium Oxide | RØnvig Dental Mfg. A/S Daugaard, Denmark | Item no. 1906 | Applying particles (size 50 μm) with sandblaster for 10 s at a distance of 5 mm |
Silorane Primer | 3M Espe, St. Paul, USA | 7AH | Applying self-etch primer for 15 s, air-blowing, polymerization for 10 s |
Silorane Bond | 3M Espe, St. Paul, USA | 7AG | Applying for 10 s, air-blowing, polymerization for 10 s |
Filtek Silorane B2 | 3M Espe, St. Paul, USA | 7AK, 8BB | Placing the material in Teflon mould, each increment max. 2.5 mm, polymerization for 40 s |
Filtek Silorane A3 | 3M Espe, St. Paul, USA | 8BR, 8CP | Placing the material in Teflon mould, each increment max. 2.5 mm, polymerization for 40 s |
After surface pretreatment, the specimens were randomly assigned to one of the 9 test groups (each n = 6):
- –
no additional treatment (NAT);
- –
silorane primer (P) and silorane bond (B);
- –
B only;
- –
sandblasting (SB) with aluminium oxide;
- –
SB plus P & B;
- –
SB with B only;
- –
Chairside silica-coating (CoJet) and silane (CJ);
- –
CJ plus P & B;
- –
CJ with B only.
A dental microblaster was used to apply aluminium oxide (groups SB, SB/B and SB/P/B) or CoJet Sand (groups CJ, CJ/B and CJ/P/B) at the surface (Dento-Prep, RØnvig Dental Mfg. A/S Daugaard, Denmark). After surface treatment, the specimens were transferred to a second Teflon mould (10 mm × 5 mm × 5 mm) and the repair was performed with a silorane placed on top of the pretreated surface using the layering technique described above. For repair, a different shade was selected in order to identify the demarcation between the lower part of the specimen (substrate for repair) and the upper part (‘repair’ silorane placed after surface pretreatment). Whole silorane specimens (5 mm × 5 mm × 10 mm) without repair served as control. The groups NAT, SB and CJ served as internal negative controls within each group of additional surface treatment (application of the silorane adhesive or bonding agent only). All specimens were mounted with sticky wax to an acrylic mount and sectioned vertically with a low speed diamond saw (Isomet, Buehler GmbH, Düsserdorf, Germany) under water cooling as described by . The non-trimming technique was used in order to obtain at least 30 beams per group. All silorane sticks were stored in saline solution for 24 h at 37 °C before microtensile bond strength (μTBS) testing. The diameter of the beams was measured with digital calipers (Powerfix ® Electronic Digital Caliper, Padget Services, London, England) with an accuracy of at least 0.01 mm and the area of the repaired surface area was calculated. Each beam was checked for voids or defects within the material with a light microscope (Stemi SV 6, Firma Zeiss, Jena, Germany). The μTBS-test was performed with a crosshead-speed of 1 mm/min in a universal testing machine (MTD-500, SD Mechatronik, Feldkirchen-Westerham, Germany). Load was applied until failure and beams were removed from the testing machine for microscopic examination using a light microscope at 8- and 50-fold magnification. As no mixed fracture occurred, the fractured beams were assigned to either adhesive or cohesive fracture type.
Statistical analysis (one-way ANOVA, Tukey HSD, p < 0.05) was performed using SPSS 15.0 software (SPSS Inc., Chicago, IL, USA).
Specimens that fractured during the mounting procedure were excluded from statistical analysis. No zero-bonds were present after water storage. In order to analyze the influence of the surface area of the specimens on the data, beams of every group were divided into specimens smaller 1.2 mm 2 and specimens not less than 1.2 mm 2 . This size of the square cross section was described by as upper limit for the non-trimming technique. Within each group, data were statistically analyzed again by Tukey HSD ( p < 0.05).
2
Materials and methods
54 silorane specimens fabricated in Teflon moulds (5 mm × 5 mm × 5 mm) were used for these experiments. For each specimen, 2 layers of silorane were placed and cured separately. The last layer was covered with a transparent matrix in order to obtain a flat surface. Each layer (thickness max. 2.5 mm) was cured for 40 s with an LED polymerization light (Elipar Freelight 2, 3 M Espe, Seefeld, Germany) with an output of at least 1000 mW/cm 2 . The LED was placed directly at the surface of the mould. The output of the curing device was controlled during each polymerization circle with a digital radiometer (bluephase meter, Ivovlar Vivadent, Schaan, Liechtenstein). Afterwards, the specimens were stored in saline solution for 24 h at 37 °C. The surfaces of all specimens were wet-polished with abrasive paper (600 grit, Buehler GmbH, Düsserdorf, Germany) in order to remove the oxygen-inhibited layer, etched with phosphoric acid (30 s) and rinsed with water (30 s). All used materials, their manufacturers and the manufacturers’ instructions for use are shown in Table 1 .
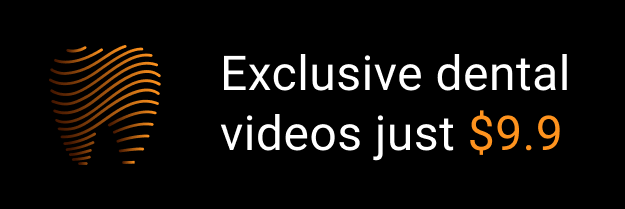