Abstract
Objective
This study investigates the potential of a novel guided tissue regeneration strategy, using fully demineralized dentin infiltrated with silica and hydroxyapatite (HA) nanoparticles (NPs), to remineralize dentin collagen that is completely devoid of native hydroxyapatite.
Methods
Dentin blocks were fully demineralized with 4 N formic acid and subsequently infiltrated with silica and HA NPs. The remineralizing potential of infiltrated dentin was assessed following a twelve week exposure to an artificial saliva solution by means of TEM, EDS and micro-CT. Measurements were taken at baseline and repeated at regular intervals for the duration of the study to quantify the P and Ca levels, the mineral volume percentage and mineral separation of the infiltrated dentin specimens compared to sound dentin and non-infiltrated controls.
Results
Infiltration of demineralized dentin with nano-HA restored up to 55% of the P and Ca levels at baseline. A local increase in the concentration of calcium phosphate compounds over a period of twelve weeks resulted in a higher concentration in P and Ca levels within the infiltrated specimens when compared to the non-infiltrated controls. Remineralization of demineralized dentin with silica NPs by immersion in artificial saliva was the most effective strategy, restoring 20% of the P levels of sound dentin. Micro-CT data showed a 16% recovery of the mineral volume in dentin infiltrated with silica NPs and a significant decrease in the mineral separation to levels comparable to sound dentin.
Significance
Demineralized dentin infiltrated with silica NPs appears to encourage heterogeneous mineralization of the dentin collagen matrix following exposure to an artificial saliva solution.
1
Introduction
Remineralization of partially demineralized dentin affected by caries or dietary/gastric acid is the process of restoring minerals to the hydroxyapatite’s latticework structure. While bacterial acids dissolve the inorganic structure of caries affected dentin, the collagen network in early-stage caries and in parts of extended carious lesions remains unaffected enabling the development of future caries treatments that remineralize the dentin . Different dentin remineralization strategies have been reported, most of which seek to restore partially demineralized dentin, focusing on the use of bioactive glass , fluoride-releasing materials , casein phosphopeptide–amorphous calcium phosphate (CPP–ACP) complexes , artificial saliva solutions , calcium hydroxide and Portland cement . Today, the science of engineered nanoparticles (NPs) offers alternative strategies for the remineralization of acid-affected enamel and dentin, either as a direct replacement of lost minerals or as a carrier for ions (Ca, P and F) that are released following particle dissolution. Nano-sized calcium fluoride (n-CaF 2 ) , nano-particulate hydroxyapatite (nHA) , nano-sized carbonated apatite (n-CAP) , carbonate-hydroxyapatite nano-crystals (nCHA) , nano-particulate bioactive glass and nano-precursors of amorphous calcium phosphates are among those nano-materials that have been reported to increase the mineral content of enamel and/or dentin. Notwithstanding, there is conflicting evidence on the efficacy of these various nano-particulate materials used as remineralizing agents for artificial carious lesions, that is most probably related to different methodologies; dentin models, demineralization techniques, type of remineralizing agents used, time of application, demineralization and remineralization cycles together with the inherent difficulty associated with analyzing the results, especially when these are of a modest order. A challenge remains in achieving effective structural and mechanical reconstitution of the remineralized dentin, which may be attributed to an imperfect arrangement of the newly deposited mineral within the demineralized tooth matrix .
A further approach using nano-particulate technology for the remineralization of partially demineralized dentin is the development of a guided tissue remineralization strategy as reported by Tay and Pashley , where they achieved intrafibrillar and interfibrillar remineralization of a 5 μm thick layer of demineralized dentin. This concept is based on the formation of an amorphous calcium phosphate phase within the collagen fibrils of demineralized dentin from first the reaction of Portland cement with water and subsequently with a phosphate containing fluid to create apatite nanocrystals and subsequently larger crystals.
In this investigation we present and assess a novel guided tissue regeneration strategy to remineralize dentin that is fully demineralized and thus completely devoid of native hydroxyapatite. Nucleating NPs embedded within the remaining collagen matrix may encourage heterogeneous mineralization of the dentin collagen matrix following immersion in an artificial saliva solution. The hypothesis being, that NPs in the inter- and intra-fibrillar collagen spaces will reduce the energy barrier required for the formation of a cluster of inorganic ions; further aided by immersion in an artificial saliva solution. We have created, and verified using scanning electron microscopy (SEM), transmission electron microscopy (TEM) and energy dispersive X-ray spectroscopy (EDS), nano-particulate scaffolds within the collagen matrix of demineralized dentin using nHA (primary particle size < 5 nm) and 12 nm colloidal silica (SiO 2 ) NPs . The aim of this study is to assess the effectiveness of this strategy as a mechanism for the remineralization of fully demineralized dentin following immersion in artificial saliva.
2
Methods and materials
2.1
Specimen preparation
Blocks of human dentin ( n = 117) of an oblong shape were obtained from the crowns of intact premolar teeth that had been extracted for orthodontic purposes. Ethical approval for the use of extracted human teeth was obtained in accordance with guidelines from the University of Sheffield. A low-speed precision blade saw (VC-50, Leco, MI, USA) with a diamond wafering blade (Buehler, Dusseldorf, Germany) was used to section the specimens (5 mm × 1 mm × 1 mm). The blocks were sonicated for 10 min to remove cutting debris and then fixed overnight at 4 °C with 3% glutaraldehyde in 0.1 M cacodylate buffer. Fixed samples were rinsed (3× 3 min) with 0.1 M cacodylate buffer to remove glutaraldehyde. Specimens were subsequently sub-divided into different experimental groups as per Table 1 . Nine of the samples remained stored in cacodylate buffer at 4 °C and assigned as ‘sound control’ samples. The remaining samples ( n = 108) were fully demineralized by storing specimens in 15 ml glass vials containing 4 N formic acid for 48 h. Preliminary experiments were conducted to identify formic acid as the optimal acid to employ and also the time required to achieve complete demineralization of the dentin samples without damaging the non-mineral components of dentin. Other demineralizing agents considered (e.g. phosphoric acid, EDTA) were found to be either too aggressive or too mild (data not shown). After the demineralization process was complete, demineralized dentin specimens were rinsed with distilled water (3× 3 min) to remove the acid; in accordance with a previously reported protocol . The demineralized dentin specimens were then equally and randomly sub-divided in four groups ( n = 27). The specimens of the first group were assigned as ‘non-infiltrated controls’, whereas the specimens in the remaining three groups were infiltrated with nano-particulate solutions as per Table 1 .
The three groups of ‘infiltrated specimens’ were stored separately in glass vials containing 15 ml of the respective infiltration solution at room temperature (25 °C). Specimens were fully immersed and the containers were kept under continuous low speed rotation (4 RPM) to avoid possible particle precipitation. Following a 24 h infiltration process, the demineralized dentin specimens infiltrated with NPs (dd-NPs) were removed from the solutions and blot dried. Three specimens from each infiltrated sample group and from the non-infiltrated controls were prepared to be examined by TEM. An equal number of specimens were also prepared for EDS ( n = 12) and micro-CT ( n = 12) analysis to determine baseline reference values in triplicate. The remaining dd-NPs specimens and non-infiltrated controls were transferred to new 50 ml polystyrene universal containers (one container per group) containing the artificial saliva solution ( Table 3 ). The artificial saliva was not renewed during the study period to allow monitoring of the remineralization potential in a close system and also to avoid accidentally rinsing the initial infiltration material. pH measurements were taken at regular intervals to ensure that the pH remained stable.
2.2
Nano-particulate infiltration solutions
The dd-NPs specimens were created by infiltration of fully demineralized dentin with one of the three different nano-particulate solutions; two nHA solutions and one nano-silica (nSiO 2 ) solution ( Table 2 ). The first nHA solution consisted of commercially synthesized nHA, manufactured according to the precipitation technique, in water (Plasma Biotal Ltd., UK); referred to as ‘control nHA’. The other nHA solution consisted of nHA, synthesized by the research group following the sol–gel technique, with sodium hexametaphosphate (SHMP) in a distilled water and acetone solution; referred to as ‘experimental nHA’. A 15% (w/v) concentration was employed for the experimental nHA to match that of the control nHA product. The nSiO 2 solution used (Ludox ® CL, Grace Davison, UK) consisted of positively charged SiO 2 NPs with a nominal particle size of 12 nm in diameter. The synthesis of the experimental nHA and material characterization of all three nano-particulate solutions have been previously described in detail by Besinis et al. .
Infiltration solution | Description of infiltration solution and manufacturer’s information | a Mean primary particle size (nm) |
---|---|---|
Control nHA | 15% nano-paste HA (Batch number: P275S, Plasma Biotal Ltd., UK) in a distilled water solution | 27.23 ± 23.96 |
Experimental nHA | 15% sol–gel nHA mixed with 5% sodium hexametaphosphate in a 50:50 distilled water and acetone solution | 3.51 ± 0.87 |
nSiO 2 | Positively charged colloidal silica (Lot number: 2007850480, Ludox ® CL, Grace Davison, UK) | 15.73 ± 2.36 |
a Primary particle diameter calculated manually from measurements on multiple TEM images. Values are mean ± SD.
2.3
Artificial saliva solution
The artificial saliva solution selected has been previously employed and successfully validated as a substitute of natural saliva for in vitro remineralization studies . The solution was prepared with distilled–deionized water and the pH was adjusted to 7.2 using 1 M HCl. Methyl hydroxybenzoate serves as an antimicrobial preservative, while sodium carboxymethyl cellulose increases the viscosity of the solution to mimic that of natural saliva. The remaining constituents provided the inorganic components required for the remineralization process at levels analogous to that of natural saliva ( Table 3 ).
IUPAC name | Chemical formula | Concentration (mM) |
---|---|---|
Methyl hydroxybenzoate | C 8 H 8 O 3 | 13.2 |
Sodium carboxymethyl cellulose | C 6 H 7 O 2 (OH) 2 OCH 2 COONa | 41.3 |
Potassium chloride | KCl | 8.4 |
Magnesium chloride hexahydrate | MgCl 2 . 6H 2 O | 0.3 |
Potassium hydrogen phosphate | K 2 HPO 4 | 4.6 |
Potassium dihydrogen phosphate | KH 2 PO 4 | 2.4 |
Calcium chloride dehydrate | CaCl 2 . 2H 2 O | 1.1 |
2.4
Transmission electron microscopy
TEM was employed as a qualitative microstructural analysis technique to characterize the various samples ( n = 15) at baseline and confirm the initial infiltration of demineralized dentin with HA and SiO 2 NPs ( Table 1 ). Following completion of the infiltration process, specimens from each sample group were prepared for TEM examination in accordance to standard specimen preparation techniques. Ultra-thin sections were obtained using a Leica EM UC6 ultramicrotome. TEM images were acquired using FEI Tecnai Spirit G2 120 kV.
2.5
Energy dispersive X-ray spectroscopy characterization
EDS was employed as a chemical microanalysis technique to characterize the various samples at baseline and confirm the initial infiltration of demineralized dentin with HA and SiO 2 NPs. EDS was further used to determine the degree of remineralization by analyzing the dd-NPs specimens from each group at regular time intervals (one week, four weeks, eight weeks and twelve weeks) and comparing them with the respective non-infiltrated controls. The degree of remineralization was assessed by measuring the net intensity of phosphorus (P) and calcium (Ca) in the infiltrated specimens after immersion in the artificial saliva solution. Calcium hydroxyapatite [Ca 10 (PO 4 ) 6 (OH) 2 ] is the mineral phase of dentin and thus an increase in the levels of P and Ca recorded suggests remineralization of dentin. The mean intensity values for P and Ca in the sound dentin controls were used as a reference (100% mineralized dentin) and all results were expressed as a percentage of the mineralization of sound dentin (mean mineralization values). This provides more meaningful categorical information as to the effectiveness of the different regimes.
At each end point, three samples were removed from each of the four containers, rinsed with distilled water and left to air-dry at room temperature for 72 h. Samples for EDS characterization were taken by slicing the oblong specimens with an ultra-thin skin-graft blade, in a manner analogous to a loaf of bread ( Fig. 1 A). The end slice was discarded, as the outer surface of this would have been exposed directly to the artificial saliva solution. Each slice was laid on its side and measurements were taken from three different zones of the internal aspect of the sample ( Fig. 1 B). Zone A corresponds to the outermost region (0–6 μm deep); zone B is the region between 6 and 30 μm in depth from the external walls of the block and zone C is the inner core of the specimen. All specimens were carbon coated prior to EDS analysis.
In this study, we employed the standardless EDAX ZAF quantification method which allows elemental microanalysis of a sample from the measured X-ray intensities (with atomic number, Z ; X-ray absorption, A ; and X-ray fluorescence, F correction). Identical operating conditions of beam energy and scanning parameter settings were used for all EDS scans in this study in order to further standardize the analysis method, increase results repeatability and allow comparison between samples. Specimen orientation and position were also kept the same. The spot size was set at 3.0 and the voltage at 15 kV. Accuracy was further enhanced by selecting the full frame scan mode instead of the spot scan mode. The surface area examined in each scan was 900 μm 2 and the scan duration was 180 s for all samples. The EDS examination was performed using a FEI Inspect F high resolution scanning electron microscopy (SEM) device equipped with an EDS detector. Data and spectra analysis was achieved using EDAX Genesis 5.21 software.
2.6
Micro-computed tomography
A micro-CT system (Skyscan 1172 micro-CT scanner, Kontich, Belgium) was employed to detect and quantify variations in the mineral composition of the dd-NPs specimens during the remineralization process. The system consisted of a sealed X-ray tube (operated with the source voltage set at 100 kV and the source current at 100 μA) and a 10 Mp Hamamatsu CCD camera (camera pixel size: 11.65 μm; object to source 31.3 mm; camera to source 348.0 mm). All specimens were positioned vertically by partially embedding them in polystyrene foam ( Fig. 1 C). Each scan yielded an image data set of 4000 × 4000 pixels (highest camera resolution available was selected). The 2D axial slices were acquired in 1 μm cross-sections. The minimum image pixel size (1.05 μm) was selected to increase the detection sensitivity of the system. All scans were performed with no filter selection in order to increase contrast between the samples and the background. The total scanning duration for each specimen was 39 min. In regards to data reconstruction, the ring artifact correction factor was set at 10 and the beam hardening correction at 49%. Data analysis was performed using the Skyscan CTAn software (version 1.9.1.0). All the micro-CT results presented in this study include data generated from 150 consecutive cross-sections (total stack height in the Z-position: 150 μm). Prior to scanning all samples had been left to dry at room temperature for 72 h in order to avoid artifacts due to shrinkage and deformation of dentin occurring during the scans.
The mineral content of the specimens was measured in two different regions; the ‘internal zone of interest’ and the ‘external zone of interest’. The internal zone of interest refers to the inner core of the dd-NPs specimens, whereas the external zone corresponds to the outermost 30 μm region ( Fig. 1 D). The changes in the mineral content were assessed by measuring the mineral volume percentage (equivalent to bone volume percentage in bone micro-CT measurements) and the mineral separation (equivalent to trabecular separation in bone micro-CT measurements). Control and dd-NPs specimens were analyzed at baseline and measurements were repeated one week and four weeks after immersion in the artificial saliva solution. All specimens were analyzed in triplicate. The mineral volume percentage was calculated according to the equation MV/NMV × 100%, where MV is the mineralized volume and NMV refers to the non-mineralized volume. Mineral separation expresses the dentin porosity and is equal to the distance between two mineralized regions separated by a non-calcified tissue (e.g. collagen) or a void. Thus in this study, high mineral percentage and low mineral separation values would indicate an increase in the mineral content of the dd-NPs specimens. The micro-CT study period was limited to the initial four weeks as the results obtained suggested no tendency for additional mineral increase and thus did not warrant further investigation with this particular analytical technique.
2.7
Statistics
All data are presented as mean ± standard deviation and were analyzed using version 20.0 of SPSS software for Windows. The differences between the infiltrated groups and the non-infiltrated controls for each of the zones of interest and end points (treatment effect), as well as the differences within each group (depth of infiltration effect and time effect), were evaluated using one-way analysis of variance (one-way ANOVA, Tukey post hoc test). Demineralized dentin infiltrated with control nHA was tested against demineralized dentin infiltrated with experimental nHA and demineralized dentin infiltrated with nSiO 2 using two-way ANOVA with Tukey’s post hoc test. All statistical analysis used a 95% confidence limit, so that p values equal to or greater than 0.05 were not considered statistically significant.
2
Methods and materials
2.1
Specimen preparation
Blocks of human dentin ( n = 117) of an oblong shape were obtained from the crowns of intact premolar teeth that had been extracted for orthodontic purposes. Ethical approval for the use of extracted human teeth was obtained in accordance with guidelines from the University of Sheffield. A low-speed precision blade saw (VC-50, Leco, MI, USA) with a diamond wafering blade (Buehler, Dusseldorf, Germany) was used to section the specimens (5 mm × 1 mm × 1 mm). The blocks were sonicated for 10 min to remove cutting debris and then fixed overnight at 4 °C with 3% glutaraldehyde in 0.1 M cacodylate buffer. Fixed samples were rinsed (3× 3 min) with 0.1 M cacodylate buffer to remove glutaraldehyde. Specimens were subsequently sub-divided into different experimental groups as per Table 1 . Nine of the samples remained stored in cacodylate buffer at 4 °C and assigned as ‘sound control’ samples. The remaining samples ( n = 108) were fully demineralized by storing specimens in 15 ml glass vials containing 4 N formic acid for 48 h. Preliminary experiments were conducted to identify formic acid as the optimal acid to employ and also the time required to achieve complete demineralization of the dentin samples without damaging the non-mineral components of dentin. Other demineralizing agents considered (e.g. phosphoric acid, EDTA) were found to be either too aggressive or too mild (data not shown). After the demineralization process was complete, demineralized dentin specimens were rinsed with distilled water (3× 3 min) to remove the acid; in accordance with a previously reported protocol . The demineralized dentin specimens were then equally and randomly sub-divided in four groups ( n = 27). The specimens of the first group were assigned as ‘non-infiltrated controls’, whereas the specimens in the remaining three groups were infiltrated with nano-particulate solutions as per Table 1 .
The three groups of ‘infiltrated specimens’ were stored separately in glass vials containing 15 ml of the respective infiltration solution at room temperature (25 °C). Specimens were fully immersed and the containers were kept under continuous low speed rotation (4 RPM) to avoid possible particle precipitation. Following a 24 h infiltration process, the demineralized dentin specimens infiltrated with NPs (dd-NPs) were removed from the solutions and blot dried. Three specimens from each infiltrated sample group and from the non-infiltrated controls were prepared to be examined by TEM. An equal number of specimens were also prepared for EDS ( n = 12) and micro-CT ( n = 12) analysis to determine baseline reference values in triplicate. The remaining dd-NPs specimens and non-infiltrated controls were transferred to new 50 ml polystyrene universal containers (one container per group) containing the artificial saliva solution ( Table 3 ). The artificial saliva was not renewed during the study period to allow monitoring of the remineralization potential in a close system and also to avoid accidentally rinsing the initial infiltration material. pH measurements were taken at regular intervals to ensure that the pH remained stable.
2.2
Nano-particulate infiltration solutions
The dd-NPs specimens were created by infiltration of fully demineralized dentin with one of the three different nano-particulate solutions; two nHA solutions and one nano-silica (nSiO 2 ) solution ( Table 2 ). The first nHA solution consisted of commercially synthesized nHA, manufactured according to the precipitation technique, in water (Plasma Biotal Ltd., UK); referred to as ‘control nHA’. The other nHA solution consisted of nHA, synthesized by the research group following the sol–gel technique, with sodium hexametaphosphate (SHMP) in a distilled water and acetone solution; referred to as ‘experimental nHA’. A 15% (w/v) concentration was employed for the experimental nHA to match that of the control nHA product. The nSiO 2 solution used (Ludox ® CL, Grace Davison, UK) consisted of positively charged SiO 2 NPs with a nominal particle size of 12 nm in diameter. The synthesis of the experimental nHA and material characterization of all three nano-particulate solutions have been previously described in detail by Besinis et al. .
Infiltration solution | Description of infiltration solution and manufacturer’s information | a Mean primary particle size (nm) |
---|---|---|
Control nHA | 15% nano-paste HA (Batch number: P275S, Plasma Biotal Ltd., UK) in a distilled water solution | 27.23 ± 23.96 |
Experimental nHA | 15% sol–gel nHA mixed with 5% sodium hexametaphosphate in a 50:50 distilled water and acetone solution | 3.51 ± 0.87 |
nSiO 2 | Positively charged colloidal silica (Lot number: 2007850480, Ludox ® CL, Grace Davison, UK) | 15.73 ± 2.36 |
a Primary particle diameter calculated manually from measurements on multiple TEM images. Values are mean ± SD.
2.3
Artificial saliva solution
The artificial saliva solution selected has been previously employed and successfully validated as a substitute of natural saliva for in vitro remineralization studies . The solution was prepared with distilled–deionized water and the pH was adjusted to 7.2 using 1 M HCl. Methyl hydroxybenzoate serves as an antimicrobial preservative, while sodium carboxymethyl cellulose increases the viscosity of the solution to mimic that of natural saliva. The remaining constituents provided the inorganic components required for the remineralization process at levels analogous to that of natural saliva ( Table 3 ).
IUPAC name | Chemical formula | Concentration (mM) |
---|---|---|
Methyl hydroxybenzoate | C 8 H 8 O 3 | 13.2 |
Sodium carboxymethyl cellulose | C 6 H 7 O 2 (OH) 2 OCH 2 COONa | 41.3 |
Potassium chloride | KCl | 8.4 |
Magnesium chloride hexahydrate | MgCl 2 . 6H 2 O | 0.3 |
Potassium hydrogen phosphate | K 2 HPO 4 | 4.6 |
Potassium dihydrogen phosphate | KH 2 PO 4 | 2.4 |
Calcium chloride dehydrate | CaCl 2 . 2H 2 O | 1.1 |
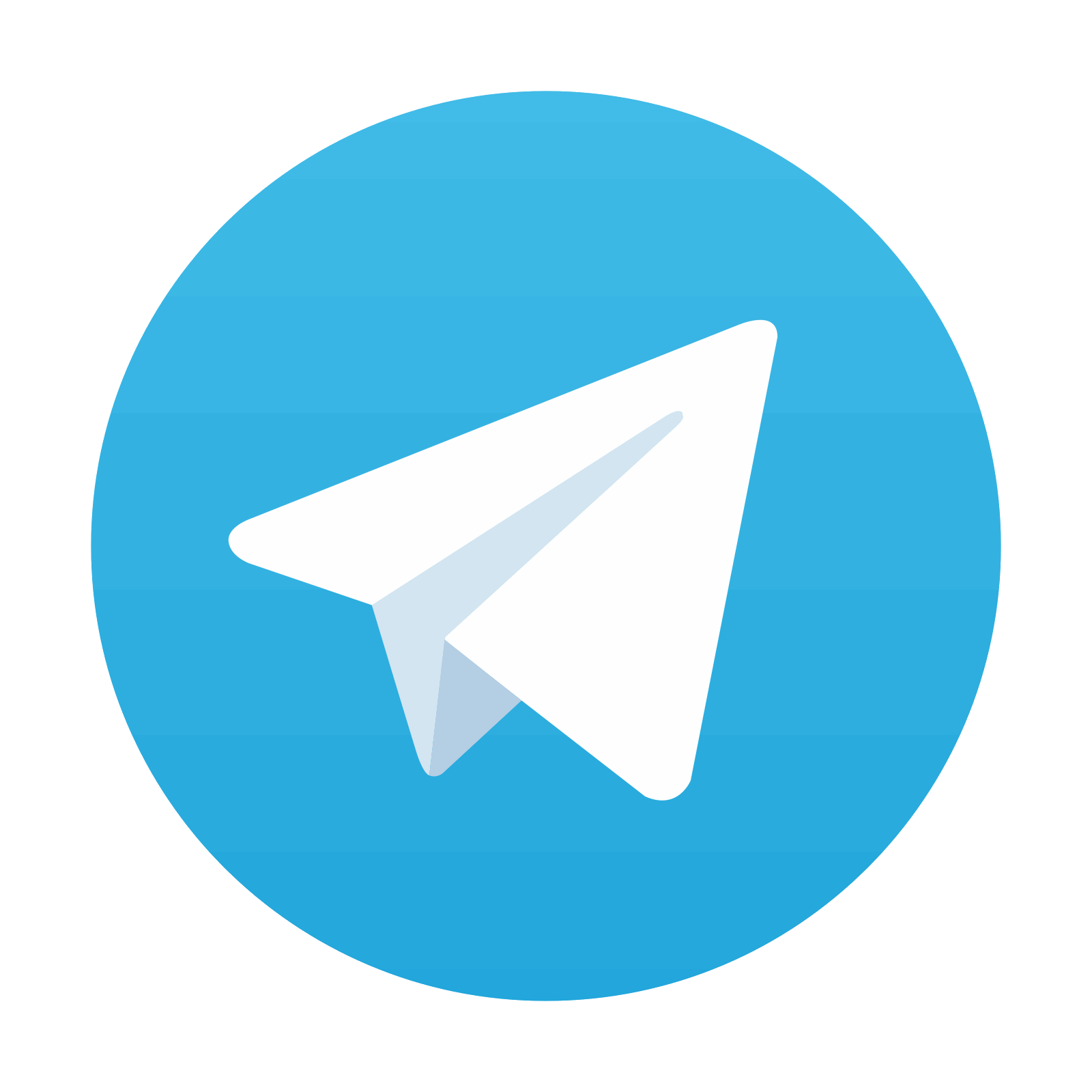
Stay updated, free dental videos. Join our Telegram channel

VIDEdental - Online dental courses
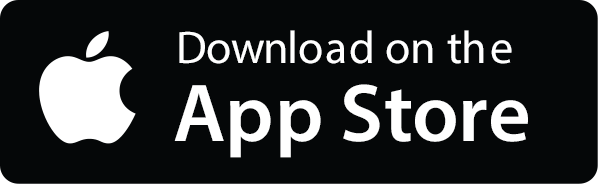
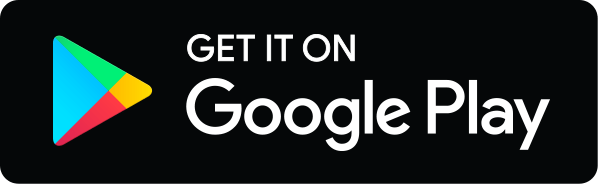
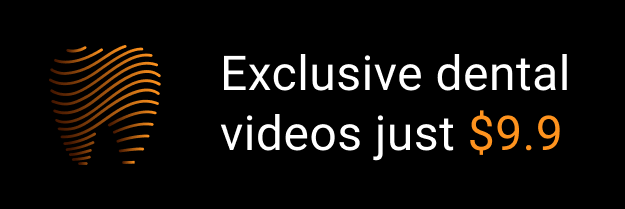