Abstract
Objective
To investigate the remineralisation of chitosan pre-treated enamel white spot lesions (WSLs) by bioglass in the presence of the pellicle layer.
Methods
50 artificial enamel white spot lesions were created by acidic gel. Two lesions were used to investigate the formation of the pellicle layer by treating with human whole saliva for 3 min. 48 lesions were assigned to 6 experimental groups (n = 8): (1) bioactive glass slurry, (2) bioactive glass containing polyacrylic acid (BG + PAA) slurry, (3) chitosan pre-treated WSLs with BG slurry (CS-BG), (4) chitosan pre-treated WSLs with BG + PAA slurry (CS-BG + PAA), (5) “standard” remineralisation solution (RS) and (6) de-ionised water (negative control, NC). Remineralisation was carried out using a pH-cycling model for 7 days. Before each treatment using remineralising agents, 3-min pellicle was formed on lesions’ surfaces. Mineral content changes, surface and subsurface microhardness and ultrastructure were evaluated by Raman intensity mapping, Knoop microhardness and scanning electron microscopy, respectively. Data were statistically analysed using one-way ANOVA with Tukey’s test ( p < 0.05 is considered as significant).
Results
Despite the heterogeneously formed pellicle layer, all groups showed an increase in surface mineral content after pH-cycling. Chitosan pre-treatment enhanced the subsurface remineralisation of WSLs using bioglass as both pre-treated groups showed greater surface and subsurface microhardness compared to NC. CS-BG exhibited denser subsurface structure than BG, while in CS-BG + PAA the crystals were bigger in size but resemble more enamel-like compared to BG + PAA as shown in SEM observations. Remineralisation of RS was limited to the surface as no significant subsurface changes of mechanical properties and structure were found.
Conclusions
Chitosan pre-treatment can enhance WSL remineralisation with bioglass biomaterials when a short-term salivary pellicle is present. A further investigation using a long-term pH-cycling model with mature pellicle is suggested with regards to clinical application.
Clinical significance
Chitosan pre-treatment has the potential in clinical application to remineralise subsurface lesions to achieve lesion consolidation.
1
Introduction
The remineralisation of enamel white spot lesion (WSL), the earliest clinical sign of caries manifesting an intact surface zone and a porous subsurface [ ], is a complex physico-chemical process because the mineral crystals remained in the lesion are less reactive [ ]. Saliva is a biological factor in dental caries which plays numerous roles including neutralizing the acid, providing mineral ions to assist remineralisation and forming the protective acquired enamel pellicle [ ].
The acquired enamel pellicle (AEP) is an organic film forms on enamel surface within minutes after exposure to saliva [ ]. This tenacious layer is thought to be insoluble in oral fluids and can grow 0.1–1.0 μm in thickness and thus establishes the foundation for the development of dental plaque [ ]. Many saliva components are found to exist in the pellicle, including proline-rich proteins, statherin and mucinous proteins, and calcium ions chelated by salivary proteins [ ]. The pellicle has been found to play an important role in reducing enamel demineralisation. Investigations suggest that the pellicle protects enamel against acidic challenge by reducing the dissolution rate of enamel [ ]. Featherstone et al. revealed that only aged pellicles formed for 72 and 168 h reduced both surface mineral loss and lesion depth compared to untreated [ ]. In-situ study found that the pellicle as young as 3 min is effective in inhibiting acidic-induced demineralisation by acting as a perm-selective membrane and regulating the diffusion of the acidic agents [ ]. This study also concluded that the pellicle layer could also influence the diffusion of calcium and phosphate ions. In addition to these beneficial effects, the pellicle, however, has been found to inhibit crystal growth. In a study into the role of fluoride on apatite growth, crystal will not grow on the site optimally covered by PRP-3 proteins [ ], which is also one of major components found in the pellicle both in vivo and in vitro [ ]. Such an inhibitory effect is also found to be associated with pellicle statherin peptides, where a covalently linked phosphate group in statherin peptides modulates the inhibition of hydroxyapatite growth [ ]. Thus, the pellicle influences the de-/remineralisation balance as a complex biological role.
Many protocols have been developed in an effort to remineralise enamel WSLs, including bioglass [ ]. Milly et al. [ ] studied enamel WSL remineralisation with 45S5 bioglass modified with polyacrylic acid (PAA) which mimics the functional role of non-collagenous proteins in binding the calcium and phosphate ions to form nano-precursors. Significant mineral deposition occurred as proved by Raman spectra and SEM images, despite the fact there was not significant reduction of lesion depth. Nevertheless, it appears that remineralisation is limited to the surface where the agent is applied, which is also an issue when using other materials such as fluoride [ ]. It is likely that fluoride is more effective in remineralising the surface layer of the carious lesion, leading to surface-blocking which may result in arrested lesion, whereas the remaining porous lesion body becomes less accessible for the remineralising agent and thus will restrict a fuller lesion consolidation [ ]. To overcome this, the delivery of ions into subsurface lesion is pivotal [ ]. In this regard, chitosan, an N -deacetylated derivative product of chitin, has been incorporated as a potential vehicle to deliver mineral formation ions into deep lesions due to its ability to penetrate enamel [ ]. The positive charge of chitosan allows it to adhere to negatively-charged surfaces, including demineralised enamel [ ]. Recent studies reveal that chitosan-amelogenin (CS-AMEL) hydrogel can induce in-vitro biomimetic remineralisation on enamel lesions, resembling formation of enamel-like crystals and reduction of lesion depth [ ]. Remineralisation kept proceeding even when pH decreased below 6.5 due to adhesion provided by the amino groups of chitosan.
However, seldom have investigations taken the pellicle into consideration when evaluating the remineralisation potential of these agents. The fact that the pellicle retards transportation of matter across the enamel may reduce the diffusion rate of ions and thereby affect subsequent remineralisation behaviour [ ]. Hence, the aim of this study was to assess the effects of chitosan pre-treatment on in-vitro dynamic remineralisation of artificial WSLs using bioglass biomaterials in the presence of the pellicle layer. Surface mineral content was evaluated by Raman intensity mapping. Surface and cross-sectional microhardness were assessed by Knoop microhardness testing. Scanning electron microscopy was used to study the ultrastructure changes after pH-cycling. The two null hypotheses tested in this study were that the pellicle had no effect on remineralisation and chitosan pre-treatment had no effect on enhancing remineralisation when the pellicle was present.
2
Materials & methods
2.1
Lesion formation
Fifty caries-free enamel slabs were sectioned off from human molar teeth with ethics approval from NHS Health Research Authority (Reference 16/SW/0220). Slabs were included in acrylic resin (Oracyl™, Bracon, UK) in a customised mould for 1 h with the natural surface facing down. The natural surface was then polished (LabForce-100, Struers, Denmark) with P500 for 10 s, P1200 for 15 s, P2000 for 30 s and P4000 for 2 min. 1 min and 4 min ultrasonication was carried out between each step and after P4000, respectively, to remove smear layer. Finished surface was protected by nail varnish (Lasting Gel Nail Colour, Collection, UK) to expose a working window about 1 mm wide and 3 mm long.
Demineralisation was conducted using an acidic gel system [ ], consisting 8 wt% methylcellulose gel and 0.1 M lactic acid (pH 4.60). The gel was prepared by dissolving methylcellulose powder (Sigma-Aldrich, USA) in boiling deionised water and stirring until room temperature was reached. Maximum five samples were placed on the base of one 250 mL glass beaker and subsequently covered by 100 mL gel, followed by storing in 4 °C fridge overnight. Afterwards, a filter paper and lactic acid were sequentially added. The demineralisation was performed in an incubator (MIR-262, Sanyo, Japan) at 37 °C for 21 days. Demineralisation solution was refreshed on a weekly basis.
After lesion formation, the acidic gel was removed and rinsed under running deionised water. Nail varnish was removed by acetone, followed by rinsing deionised water.
2.2
In-vitro formation of the pellicle layer
Human whole saliva was collected from one subject. Before collection, the subject was prohibited from eating or drinking. The subject was instructed to chew paraffin gum and saliva was generated, collected in a polystyrene tube (Sterilin™, Thermo Fisher Scientific, UK) and stored in −80 °C freezer. The collected saliva sample had a high buffer capacity (Saliva-Check Buffer Kit, GC, Japan) with a pH of 7.60. The rheological property (spinnbarkeit) was measured to be 2.60 ± 0.32 mm (IMI-0501 NEVA Meter, Ishikawa Ironworks Co., Japan). Before each use, saliva samples were fully defrosted in the tube in a water bath at room temperature.
Two lesion samples were used to investigate the in-vitro formation of the pellicle layer. Saliva was dropped to cover half of the lesion. The culture was conducted for 3 min in a mini orbital shaker (SO5, Stuart Scientific, UK) at a shaking speed of 62.5 RPM. Once completed, excessive saliva was gently washed off under running deionised water. Subsequently, lesions were carefully dried using compressed air, and gold-coated for SEM observations (JCM-6000, JEOL, Japan) in secondary electron mode with acceleration voltage of 15 keV.
2.3
Group assignment
All remaining lesions were assigned to six groups (n = 8): (1) NovaMin™ (BG) slurry, (2) NovaMin™ containing polyacrylic acid (BG + PAA) slurry, (3) chitosan pre-treated WSLs with NovaMin™ slurry (CS-BG), (4) chitosan pre-treated WSLs with NovaMin™ + PAA slurry (CS-BG + PAA), (5) remineralisation solution (RS) and (6) de-ionised water (negative control, NC).
2.4
Baseline surface Raman intensity mapping
Five samples from each group were used for obtaining baseline surface Raman intensity maps. The acquisition was carried out by a micro-Raman spectrometer (inVia Raman Spectroscopy, Renishaw, UK) assembled with a 1200 line/mm grating, using Streamline™ mode at the wavelength of 785 nm. The scanning area was determined by drawing a rectangle on a montage image which included lesion area and untouched sound enamel on both sides. Each area contained 91,000 acquisition points with a step size of 2.7 μm in x and y direction. 960 cm −1 was chosen as the monitored peak because it is the strongest peak of phosphate in tooth enamel and can be used to indicate the mineral content [ ].
After acquisition, all maps were processed using in-house software. Afterwards, fitted peak height maps were analysed by ImageJ (NIH, US) for the quantification of surface mineral content. The intensity ratio of lesion/sound enamel (I lesion /I sound ) at 960 cm −1 was calculated to indicate the mineral content of the artificial lesion assuming sound enamel was 100% in mineral content.
2.5
Baseline surface microhardness
Surface microhardness (SMH) was carried out on the same samples by a microhardness tester fitted with a Knoop diamond indenter (Duramin, Struer, Denmark). A load of 10 gf and dwell time of 5 s were used. For each sample, 5 indentations were measured with a spacing of at least 50 μm.
2.6
pH-cycling regime
In the present study, a pH-cycling model adapted from Karlinsey [ ] was incorporated as described in Fig. 1 . All chemicals were summarised in Table 1 . In general, each cycle included a three-minute treatment in the morning, an immersion in artificial saliva for two hours, an acidic challenge in the lesion formation gel for four hours, followed by another two-hour immersion in artificial saliva and three-minute treatment, and finally a storage in artificial saliva overnight.

Material | Compositions |
---|---|
BG slurry (1 g/L) | NovaMin™ 4516 bioactive glass a (1 g), deionised water (1 mL) |
BG + PAA slurry (1 g/L) | NovaMin™ 4516 bioactive glass a (0.6 g), polyacrylic acid powder (0.4 g), deionised water (1 mL) |
Chitosan solution (2.5 mg/mL) | Chitosan powder (25 mg), 0.1 M acetic acid solution (10 mL) |
“Standard” remineralisation solution [ ] | HEPES (20 mM), KCl (130 mM), CaCl 2 (1.5 mM), KH 2 PO 4 (0.9 mM), pH adjusted to 7.0 by 1 M NaOH |
Artificial saliva | CaCl 2 (0.7 mM), MgCl 2 (0.2 mM), KH2PO4 (4.0 mM), HEPES (20 mM), KCl (30.0 mM), pH adjusted to 7.0 by 1 M NaOH |
Before the first cycle, sound enamel was protected with acid-resistant tape to minimise contaminants from treatments. Prior to each three-minute treatment, the pellicle layer was formed in the fashion as described in Section 2.2 . Afterwards, CS-BG and CS-BG + PAA groups were pre-treated with chitosan solution, which was prepared by dissolve 25 mg chitosan (50–190 kDa, Sigma-Aldrich, US) in 10 mL acetic acid (0.1 M) and stirred overnight at room temperature to form an aqueous solution of 2.5 mg/mL [ ]. After pre-treatment, excessive chitosan was carefully washed off by deionised water.
To prepare BG slurry (1 g/mL), 1 g powder was fully mixed with 1 mL deionised water using a whirlmixer for 1 min. The pH was 12.7. To prepare BG-PAA slurry (1 g/mL, 60/40 wt/wt), 0.6 g BG, 0.4 g PAA powder and 1 mL deionised water were mixed by a whirlmixer for 1 min. The pH was 8.1. The treatment was carried out using a toothbrush machine (Toothbrush, Syndicad, Germany) adapted with microbrush (Benda ® Microtwin ® , CENTRIX, Australia) at a speed of 1 s per stroke (180 strokes overall). The applied load was approximately 10 g. Samples were fixed and remineralising slurries or solutions were applied directly on the lesion surface. After brushing, samples were thoroughly rinsed with deionised water.
After pH-cycling, samples were rinsed with running deionised water to remove any remnants on the lesion surfaces. Protection tape was removed and samples were stored in deionised water at 4 °C before use.
2.7
Characterisations post-treatment
To quantify the change of surface mineral content post treatment, Raman intensity mapping was repeated on the same samples used in Section 2.4 at the same test area. The change of I lesion /I sound was calculated as:
I n t e n s i t y C h a n g e = ( I l e s i o n I s o u n d ) p o s t − ( I l e s i o n I s o u n d ) b e f o r e
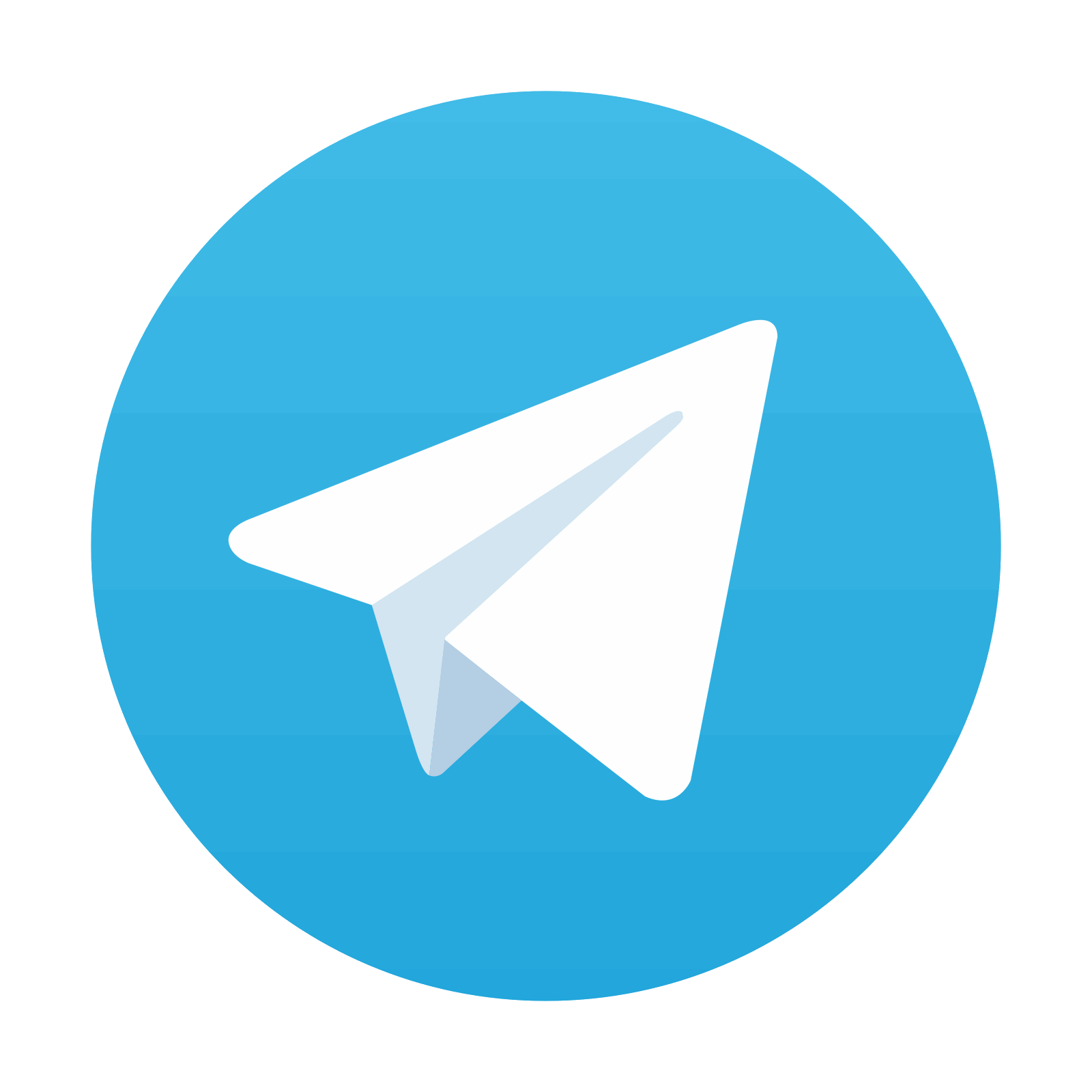
Stay updated, free dental videos. Join our Telegram channel

VIDEdental - Online dental courses
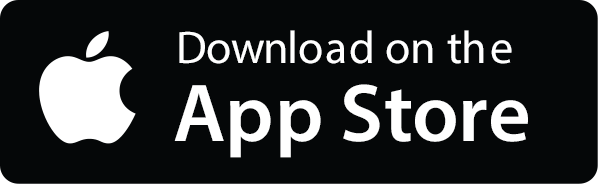
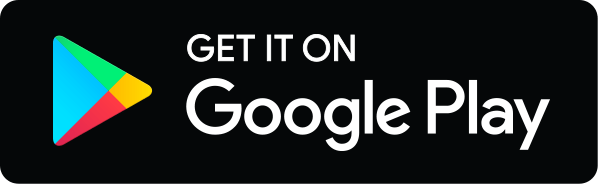