Introduction
The purpose of this study was to examine changes in registration accuracy after including occlusal surface and incisal edge areas in addition to the buccal surface when integrating laser-scanned and maxillofacial cone-beam computed tomography (CBCT) dental images.
Methods
CBCT scans and maxillary dental casts were obtained from 30 patients. Three methods were used to integrate the images: R1, only the buccal and labial surfaces were used; R2, the incisal edges of the anterior teeth and the buccal and distal marginal ridges of the second molars were used; and R3, labial surfaces, including incisal edges of anterior teeth, and buccal surfaces, including buccal and distal marginal ridges of the second molars, were used. Differences between the 2 images were evaluated by color-mapping methods and average surface distances by measuring the 3-dimensional Euclidean distances between the surface points on the 2 images.
Results
The R1 method showed more discrepancies between the laser-scanned and CBCT images than did the other methods. The R2 method did not show a significant difference in registration accuracy compared with the R3 method.
Conclusions
The results of this study indicate that accuracy when integrating laser-scanned dental images into maxillofacial CBCT images can be increased by including occlusal surface and incisal edge areas as registration areas.
Highlights
- •
Registration areas were compared for integrating laser-scanned and CBCT images.
- •
Using facial surfaces only caused the greatest discrepanices.
- •
Using incisal edge and marginal ridge was comparable with using them plus facial surfaces.
- •
Incisal edge and marginal ridge are appropriate for integrating laser-scanned and CBCT images.
With the advent of computed tomography (CT), the 3-dimensional (3D) shape and position of the jaw bone can be accurately represented. The potential to manage patients with dentofacial deformity in 3 dimensions is now available, since CT scan data can provide accurate and detailed information. The recently developed cone-beam CT (CBCT) system also improves on conventional CT by providing comparable images to conventional CT at reduced radiation dose and cost.
By using CBCT images, much virtual reality surgical planning and simulation of orthognathic surgery can be attempted. However, a drawback of CBCT imaging is that detailed occlusal data cannot be obtained. Moreover, artifacts are commonly encountered in clinical CBCT images. The many different types of artifacts reported include beam hardening, inhomogeneity, and truncation influence on image contrast and bone border definition in CBCT. In addition, if the patient has an orthodontic bracket or metallic dental restoration, metallic artifacts are observed on the images as a result of the radiographic scattering effect, making it more difficult to assess the shape of teeth or their state of occlusion. Thus, the image quality obtained from CBCT is not sufficient for a precise representation of the dentition, particularly for a 3D surface model.
Laser surface scanning is common in industry and medicine as a noninvasive alternative for generating a 3D computerized image. Accurate 3D data can be obtained by using a surface laser scanner. For these reasons, many authors have tried to incorporate digital dental models into a computerized 3D bone model, creating a composite model, which resolves the limitations of current planning systems.
Since registration accuracy has a direct effect on the precision of the 3D composite head model, clinicians are seeking methods to reduce any errors in registration. Marker-based registration is widely used, with efforts being made to reduce errors by increasing the number of markers. In addition, markers that are preoperatively fixed to the bones or teeth for a more precise registration can cause inconvenience to the patient, and the procedures are rather complicated for practitioners. Swennen et al, who used fiducial markers for registration, also adopted a new approach to augment a 3D virtual skull model with detailed occlusal and intercuspation data without fiducial markers, using the so-called “triple CBCT scan” procedure. Although fiducial markers were not used, a drawback was that the subjects needed to be scanned by CT more than once. Thus, surface-based registration was introduced that uses surface information from 2 data sets to calculate the rotation and translation between the data sets, further advancing the field.
Kim et al showed that the integration can be made with good accuracy with the use of sequential point- and surface-based registration: in other words, without the aid of fiducial markers. Noh et al showed that registration accuracy can be increased by using a broad area for registration. Because accuracy can be increased by increasing the registration area, including the incisal edges of the anterior teeth and some visible occlusal surfaces on the posterior teeth might contribute to increased registration accuracy. The purpose of this study was to examine whether registration accuracy improves when the visible occlusal surface and incisal edge areas are included in the registration area along with the buccal surfaces when integrating a laser-scanned dental image into a maxillofacial CBCT image.
Material and methods
This study was approved by the institutional review board of Chonnam National University, Gwangju, Korea. All patients in the study gave informed consent to participate. Thirty adults with normal occlusion and no missing teeth were enrolled in this study. Patients with prosthetic crown restorations were excluded. The scans were obtained with a CBCT scanner (Alphard Vega; Asahi Roentgen, Kyoto, Japan) under the following conditions: 80 kV; 5 mA; voxel size, 0.39 × 0.39 × 0.39 mm; and field of view, 200 × 179 mm. Each maxillary dental plaster cast was obtained from impressions taken with alginate (Cavex Impressional; Cavex Holland, Haarlem, The Netherlands). The plaster casts were then scanned by using a 3D laser scanner (Orapix, Seoul, Korea).
The DICOM file obtained from the CBCT was reconstructed into 3D images and then converted to stereolithography format by using a DICOM viewer program (version 1.0; Orapix). The laser-scanned file of the plaster casts was converted to a 3D image by using a 3D reverse engineering software program (Rapidform 2006; Inus, Seoul, Korea). The laser-scanned data, which was a Rapidform points file (Rapidform points file; Inus), was changed from the data consisting of points to the polygonal form by using the “triangulate” function. At this time, all data with a side of the polygon greater than 0.5 mm and a facing angle greater than 70° were excluded. After auto alignment, the dental images were completed by filling the detailed parts that could not pass through a laser beam while being scanned; these were mainly interproximal areas, and they were incorporated by repeating the “smooth and curvature option” of the “fill hole” function. In addition, the data were converted to an Rapidform polygon file (Rapidform polygon file; Inus). Since the soft tissue area could increase the error range, the remaining areas were cut out along with the gingival margin to superimpose the clinical crowns alone.
Each laser-scanned image of a maxillary arch was registered onto a CBCT image by using the Rapidform 2006 software. First, the initial registration was performed by selecting 3 points corresponding to each laser-scanned image and CBCT image. Subsequently, the regional registration was used to finalize the registration.
When the region of interest was selected for regional registration, all anterior teeth and the second molars were included. The first and second premolars, and the first molar areas were excluded due to artifact development. To evaluate the effect of the incisors on the visible occlusal surface and incisal edge as the registration region, 3 types of implementation were performed according to the region: (1) only the buccal and labial surfaces (R1), (2) the incisal edges of anterior teeth, and the buccal and distal marginal ridges of second molars (R2), and (3) labial surfaces including incisal edges of anterior teeth and buccal surfaces including buccal and distal marginal ridges of second molars (R3) ( Fig 1 ).

Registration was performed with an iterative closest point algorithm by using the “shell/shell deviation” function, and the threshold value was set at 1 mm in the program. In addition, the differences between the 2 images were evaluated with color-mapping methods.
The maxillary teeth were removed from the CBCT model, leaving the laser-scanned digital dental model in place. After this, the “composite models” were created by selecting the “merge” function in the Rapidform 2006 program. Bone-to-tooth measurements were made from a bony landmark to a dental landmark and were used to assess the accuracy of the alignment of the laser-scanned digital dental model with the CBCT model. The definitions and measurements are listed in the Table . All measurements were performed by the same investigator (L.J.S.), and each measurement was repeated 3 times on different days. The difference in measurements between the CBCT models and the generated “composite model” was recorded as the absolute value of the deviation to either positive or negative.
R1 | R2 | R3 | Significance ( P value) |
|
---|---|---|---|---|
Mean ± SD | Mean ± SD | Mean ± SD | ||
Shell/shell deviation | 0.33 ± 0.05 a | 0.28 ± 0.04 b | 0.27 ± 0.04 b | <0.001 |
Statistical analysis
All data were transferred to an Excel spreadsheet (Microsoft, Redmond, Wash) and analyzed using the SPSS statistical program software (version 21; IBM, Armonk, NY). The means and standard deviations of the shell/shell deviation were calculated for all registration methods. One-way analysis of variance was used to analyze the differences in shell/shell deviation according to the registration area, and the Tukey test was used for post-hoc comparisons.
The differences in 3D cephalometric measurements data among the 3 methods were compared using the Wilcoxon signed rank test. The Pearson correlation test was performed to identify any possible differences between the CBCT model and the generated “composite model.”
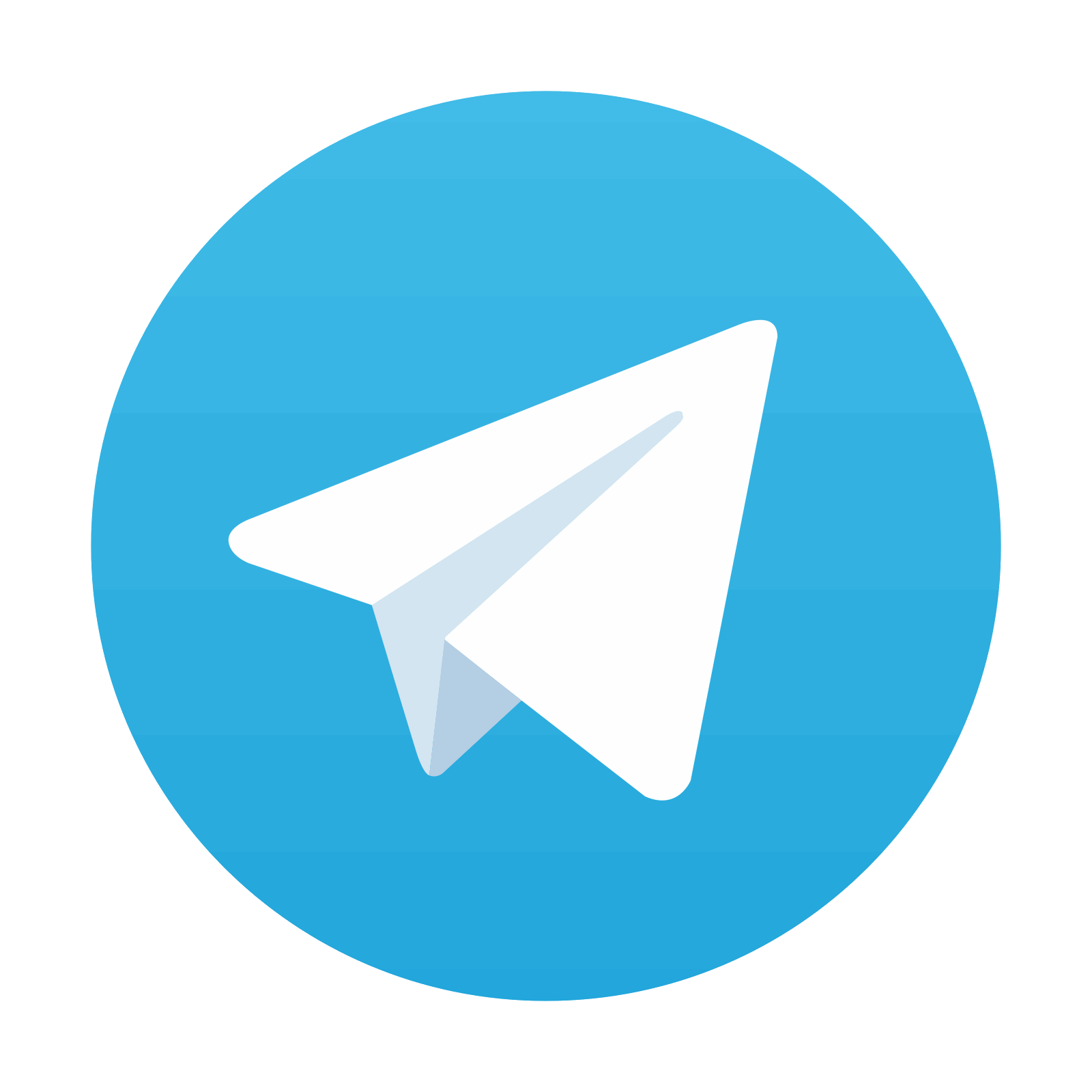
Stay updated, free dental videos. Join our Telegram channel

VIDEdental - Online dental courses
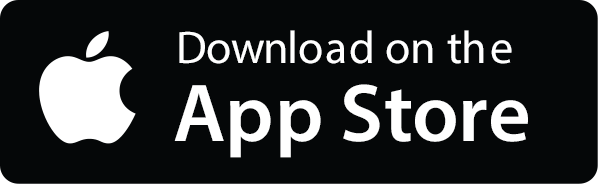
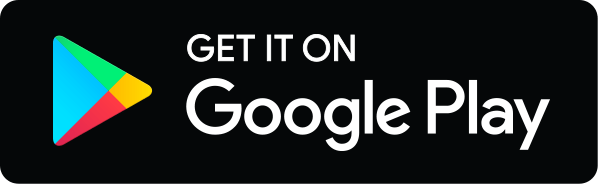