Highlights
- •
A RAFT-based initiating system successfully provided nanogels with latent reactivity.
- •
The nanogel additives progressively reduced photopolymerization shrinkage and stress.
- •
RAFT residual functionality on nanogels affected conversion but not polymer strength.
Abstract
Objective
This study examines how nanogel structure correlates with photopolymerization and key polymer properties upon addition of nanogels with latent reactivity into a monomer dispersant to produce polymer/polymer composites.
Methods
Two nanogels that retained RAFT functionality based on the synthetic approach were prepared to have different branching densities. These reactive nanogels were dispersed in triethylene glycol dimethacrylate at 0–40 wt%. Reaction kinetics, volumetric shrinkage and shrinkage stress associated with the photopolymerization of nanogel-modified formulations were measured in real time with mechanical properties of the polymers also evaluated. The basic structure of RAFT-derived nanogel particles was examined by the preparation of a separate nanogel constructed with degradable disulfide crosslinking groups. The model nanogel molecular weight and polydispersity were compared before and after degradation.
Results
Despite the controlled radical synthetic approach, the nanogels, which are composed of multiple interconnected, short primary chains, presented relatively high polydispersity. Through addition of the reactive nanogels to a monomer that both infiltrates and disperses the nanogels, the photopolymerization rate was moderately reduced with the increase of nanogel loading levels. Volumetric shrinkage decreased proportionally with nanogel concentration; however, a greater than proportional reduction of polymerization-induced stress was observed. Mechanical properties, such as flexural strength, storage modulus were maintained at the same levels as the control resin for nanogel systems up to 40 wt%.
Significance
This study demonstrated that beyond the use of RAFT functionality to produce discrete nano-polymeric structures, the residual chain end groups are important to maintain reactivity and mechanical properties of nanogel-modified resin materials.
1
Introduction
Polymeric particles composed of multiple chains with relatively dense internal crosslinking or cyclization can be considered as highly branched polymers, or nanogels when the dimensions are below 100 nm. Dendrimers, represent a class of commonly studied highly branched polymers, which are monodisperse tree-like polymer with precise control over the polymer architecture. Significant attention has been directed toward the synthesis of dendrimers with different chemistries and properties for various applications . However, it generally requires multiple steps to obtain the final dendrimer structure and the number of generations or final molecular weight is normally limited due to the de Gennes dense packing effect . To overcome these limitations, one-pot synthesis has been applied to form hyperbranched polymers. A step-growth process is generally used with either single monomer (e.g., AB 2 ) or multi-monomer (e.g., A 2 + B 3 ) methodology to generate imperfect (compared to dendrimers) hyperbranched structures due to the non-uniform growth of branching points . Sherrington and coworkers developed a facile method to polymerize methyl methacrylate in the presence of modest amounts of a dimethacrylate crosslinker based on a free radical chain-growth mechanism. Polymeric nanoparticles were obtained with chain transfer agent and solvent used to prevent macrogelation. High molecular weight, multi-chain polymeric structures were formed by these reactions. Different from typical polycondensation reactions, branch points in these nanogels are based on cyclization and crosslinking introduced by the reaction of pendent vinyl groups with radicals either from the same molecule or another propagating polymer. As a simple technique with versatile monomer choices, this method has been applied to make a variety of nanogels with different monomers by either free radical or controlled radical polymerization methods . Due to the low concentration of crosslinker (typically 1–2 mol% of monomers), limited crosslinks are formed for each individual chain so there is significant degree of linear polymer formation during nanogel synthesis. It was demonstrated that the nanogel synthesis often led to significant amounts of linear species formation with either free radical or controlled radical polymerization . Recently, our group has extended the synthesis of nanogel structures through similar approaches but with the use of significantly higher concentrations of crosslinker in the system . This generated nanogel structures with substantially higher crosslinking/branching density ([crosslinker]/[monomer] = 30/70 mole ratio) than other approaches resulting in high numbers of branching points per nanogel. In order to prevent macrogelation, relatively high concentrations of chain transfer agent were applied to limit primary chain length. An additional study with up to 50 mol% of crosslinker in the system was demonstrated for nanogel synthesis through a similar approach using higher solvent concentration to enhance cyclization and suppress macrogelation .
These nanogel materials have been applied to form discontinuous and co-continuous nanocomposite morphologies with resin matrices as a means to modify polymer network properties for alleviation of shrinkage stress during photopolymerization. During polymerization, volumetric shrinkage is typically observed due to the decrease of free volume during the transition from a mobile liquid monomer to a constrained polymer. Particularly in crosslinking bulk polymerizations, polymerization-induced stress starts to accumulate beyond the gel point with a decrease of stress relaxation as modulus of the polymer network develops. Shrinkage stress can affect material performance and lead to premature failure based on the development of interfacial or bulk defects. A variety of methods have been applied to reduce shrinkage and stress including development of new monomers , adoption of step-growth curing mechanisms and addition of secondary fillers . Incorporation of inorganic fillers has a demonstrated ability of shrinkage reduction determined by volume fraction but depending on the effect of the filler on bulk modulus, higher residual shrinkage stress can result for these composite materials . Nanogel, as an organic filler, was recently developed and showed significant potential for shrinkage stress reduction . The utilization of nanogels swollen by monomers, has the advantage of forming well-controlled physically interpenetrated and covalently interconnected polymer networks. During the secondary polymerization between nanogel and matrix monomers, both the chemical and physical crosslinking between nanogel and resin are important for the mechanical performance of the final materials. In order to enhance crosslinks between nanogel and matrix for reinforcing the mechanical properties, either further modification of nanogel with reactive groups or increasing nanogel crosslinking density is required. Further modification requires additional reaction to introduce reactive groups that may complicate the nanogel synthesis procedure. Special attention is usually required for the synthesis of nanogels with very high branching densities due to the tendency for macrogelation.
As a part of this study, reactive nanogels were synthesized with a disulfide-containing crosslinker and monomethacrylate in the presence of a styrene dimer-based RAFT (reversible addition-fragmentation chain-transfer) agent. The internal disulfide connections allow subsequent intentional deconstruction of the nanogels as a means to probe their basic structure; however, these same linkages introduce difficulties and instabilities during the secondary photopolymerization of nanogel-modified resin formulations. As such, the disulfide dimethacrylate was replaced with a non-degradable urethane dimethacrylate crosslinker in the RAFT-based nanogel synthetic approach to provide a separate series of branched nanogels for study as potential shrinkage and stress-limiting resin additives.
2
Materials and methods
2.1
Materials
Isobornyl methacrylate (IBMA), benzyl methacrylate (BzMA), 2,4-diphenyl-4-methyl-1-pentene (also known as α-methylstyrene dimer, AMSD), 2-hydroxyethyl disulfide, methacryloyl chloride, triethylamine (TEA), 4-dimethylaminopyridine (DMAP), dithiothreitol (DTT), azobisisobutyronitrile (AIBN) and triethylene glycol dimethacrylate (TEGDMA) were obtained from Sigma–Aldrich. 2,2-Dimethoxy-2-phenylacetophenone (DMPA) was obtained from BASF. The structures of the monomers and AMSD are shown as Fig. 1 . Urethane dimethacrylate (UDMA) was obtained from Esstech. (Essington, PA). The inhibitor was removed from IBMA by treatment with activated basic alumina (Brockman I, Sigma-Aldrich). AIBN was recrystallized from methanol. All other materials were used as received.

2.2
Degradable disulfide dimethacrylate synthesis
Disulfide dimethacrylate (DSDMA) was synthesized according to a prior literature report . Methacryloyl chloride (5.64 g, 36.5 mmol), TEA (11.09 g, 109.6 mmol) and DMAP (0.45 g, 3.65 mmol) was dissolved in 150 ml anhydrous dichloromethane (DCM). The solution was purged with nitrogen gas and cooled by ice bath for 30 min. 2-Hydroxyethyl disulfide (5.64 g, 36.5 mmol) was added to the solution in a dropwise fashion. The reaction mixture was allowed to warm to room temperature under nitrogen and held for 24 h. Afterwards, the reaction was quenched by addition of deionized water and subsequently washed twice with a DI H 2 O, Na 2 CO 3 (10%), brine solution. The organic phase was dried over Na 2 SO 4 and the solvent removed under vacuum. Yellow oil was obtained in 80.2% yield. The monomer structure was verified by 1 H NMR (400 MHz, chloroform- d ): δ (ppm) 6.15–6.14 (m, 1H), 5.69–5.54 (m, 1H), 4.43 (t, J = 6.6 Hz, 2H), 2.99 (t, J = 6.6 Hz, 2H), 1.96 (dd, J = 1.6, 1.0 Hz, 3H).
2.3
Polymer and nanogel preparation
A linear polymer of isobornyl methacrylate (PIBMA) was synthesized in the presence of 15 mol% of AMSD (relative to IBMA content) with 1 mol% AIBN in ethyl acetate (1:1 by weight) as solvent. Polymerization was carried out at 80 °C for 24 h in a nitrogen environment with 82.3% vinyl group conversion as determined by FT-IR. The homopolymer sample was isolated by precipitation from an excess of methanol with the residual solvent removed under vacuum to obtain the final oligomeric material as a powdery solid.
A linear block copolymer was then synthesized from the reaction of the isolated PIBMA with benzyl methacrylate (BzMA) using AIBN (0.5 mol% based on IBMA repeat units). The molar ratio of BzMA monomer to IBMA units in PIBMA was 2:1. A four-fold excess of ethyl acetate was used and the reaction was conducted for 12 h at 80 °C under nitrogen. With the BzMA vinyl group conversion at 80.4%, the copolymer was isolated by precipitation from methanol to yield a powdery solid material.
A degradable nanogel was prepared by the copolymerization of DSDMA and IBMA (30:70 mole ratio) with 15 mol% AMSD and 1 mol% AIBN (related to total monomers) in a four-fold excess of ethyl acetate. Polymerization was carried out at 80 °C for 24 h in a nitrogen environment with 81.4% final vinyl group conversion. Samples were taken at various intermediate time points with the nanogels being isolated by precipitation from an excess of methanol and then dried under vacuum to obtain a pale yellow powder.
2.4
Disulfide nanogel degradation
Nanogel prepared with disulfide dimethacrylate (0.10 g, about 0.15 mmol disulfide functionality) was dissolved in inhibitor-free tetrahydrofuran (THF; 6 ml). DTT (0.15 g, 1.0 mmol) was added under a nitrogen environment. The reaction was allowed to proceed at room temperature and aliquots of samples were withdrawn periodically and immediately diluted in THF for GPC analysis.
2.5
Non-degradable nanogel synthesis
Two additional nanogels were synthesized without the cleavable disulfide crosslinks. UDMA and IBMA were mixed at 10:90 or 30:70 molar ratios and 15 mol% of AMSD and 1 mol% of AIBN (relative to monomers) was added with a four-fold excess of ethyl acetate. Polymerization was continued for 4 h at 80 °C under a nitrogen environment. Samples were precipitated from an excess of methanol and dried under vacuum to obtain white powdery materials. The conversion of vinyl groups was 48.4% and 38.5%, respectively, for RN19 and RN37 (RN refers to reactive nanogel, and the numbers indicate the crosslinker to monomer molar ratios).
RN19 and RN37 were added to TEGDMA in mass ratios of 10–40% in 10% increments. DMPA as photo initiator (0.5 wt%, relative to TEGDMA) was added to each sample. Unfilled TEGDMA with DMPA was used as control system. Nanogels were fully dispersed into TEGDMA and formed stable, transparent mixtures following mechanical agitation.
2.6
Polymer structural analysis
GPC (Waters 515) was employed for the analysis of linear polymer and nanogel molecular weight and polydispersity index (PDI) based on polystyrene calibration standards. Tetrahydrofuran was used as eluent at a flow rate of 1 ml/min at 35 °C. A 400 MHz NMR (Bruker Avance-III 400) was used for collection of 1 H spectra of the monomers as well as polymeric structures in either CDCl 3 or CD 2 Cl 2 .
2.7
Photopolymerization kinetics
Real-time near-infrared (NIR) spectroscopy was applied to monitor the reaction kinetics of the TEGDMA-dispersed nanogel formulations during the photopolymerization process. The methacrylate group ( CH 2 ) has an overtone absorbance peak at 6165 cm −1 , which was monitored throughout the reaction. All specimens ( n = 3) were irradiated under 365 (±10) nm UV light with a 10 mW/cm 2 irradiation intensity as determined by radiometer at the sample surface position.
2.8
Volumetric polymerization shrinkage
A non-contact linear variable differential transducer (LVDT)-based linometer (Academic Center for Dentistry Amsterdam, The Netherlands) was used for real-time volumetric shrinkage testing. Samples (compressed cylindrical shape of 3–4 mm diameter and 1 mm thickness) were placed on an aluminum disk with a glass slide covering the top. A thin film of applied grease on the aluminum disk and glass slide substrates allowed the sample to shrink freely in the horizontal as well as vertical dimensions. The transducer underneath the aluminum disk monitored the z -axis linear displacement of the sample during polymerization that was then used to calculate the real-time volumetric shrinkage. All specimens ( n = 3) were irradiated under 365 (±10) nm UV light with 10 mW/cm 2 irradiant intensity with limiting shrinkage data reported at the end of the 10 min illumination interval.
2.9
Shrinkage stress measurement
A tensometer (Volpe Research Center, American Dental Association, Gaithersburg, MD) was utilized for measurement of real-time polymerization shrinkage stress . All cylindrical samples (6 mm diameter and 1 mm thickness) were placed between two polished, silane-methacrylate-treated quartz rod surfaces (6 mm diameter) where the top rod was attached by a collet connected to the stainless steel beam of the cantilever tensometer. The bottom glass rod, which was fixed to the tensometer base with a second collet, was also used as a light guide extension for photocuring with an adapter connecting the tip of the UV light guide with the rod. Specimens ( n = 3) were irradiated under 365 (±10) nm UV light at intensity of 10 mW/cm 2 (measured by radiometer at the exit of the glass rod) for 15 min. The deflection of the calibrated cantilever beam was monitored by a LVDT detector with displacement converted to the shrinkage stress output.
2.10
Mechanical property testing
Flexural mechanical properties were collected by a universal testing machine (Mini-Bionix II, MTS, Eden Prairie, MN). Specimens with dimensions of 25 mm × 2 mm × 2 mm were cured under 365 (±10) nm light irradiation for 25 min with 5 mW/cm 2 intensity. Flexural modulus and strength were determined in three-point bending tests on a 20 mm span at a crosshead speed of 1 mm/min after approximately 48 h storage in the dark at room temperature. Vinyl group conversion for the mechanical testing samples ( n = 3) was measured with NIR shortly after the photopolymerization.
A dynamic mechanical analyzer (DMA, Perkin Elmer 8000, Waltham, MA, USA) was employed to measure the bulk nanogel glass transition temperature ( T g values by sandwiching 10 mg of nanogel powder in aluminum pockets. A single cantilever cyclic displacement at 1 Hz was applied while the sample ( n = 3) was heated to 180 °C and then cooled back to room temperature at 2 °C/min with tan δ data collected during the cooling process. The T g was assigned as the maximum in the tan δ output. This technique has been validated using film and powdered polymer standards of known T g .
A TA Q800 DMA was applied for measuring the storage moduli ( G ′) and tan δ behavior of fully-cured nanogel-modified compositions and the TEGDMA homopolymer control. Samples were prepared under the same curing conditions as employed with the flexural strength testing; however with different specimen dimensions of 25 mm × 3 mm × 1 mm. The photocured specimens ( n = 3) were post-cured at 160 °C overnight to reach essentially full conversion (>98%) to avoid any post-cure effects during the thermal analysis testing, which utilized a tensile test mode with a strain rate and frequency of 0.02% and 1 Hz, respectively during the temperature scan from 0 °C to 220 °C at 2 °C/min.
2
Materials and methods
2.1
Materials
Isobornyl methacrylate (IBMA), benzyl methacrylate (BzMA), 2,4-diphenyl-4-methyl-1-pentene (also known as α-methylstyrene dimer, AMSD), 2-hydroxyethyl disulfide, methacryloyl chloride, triethylamine (TEA), 4-dimethylaminopyridine (DMAP), dithiothreitol (DTT), azobisisobutyronitrile (AIBN) and triethylene glycol dimethacrylate (TEGDMA) were obtained from Sigma–Aldrich. 2,2-Dimethoxy-2-phenylacetophenone (DMPA) was obtained from BASF. The structures of the monomers and AMSD are shown as Fig. 1 . Urethane dimethacrylate (UDMA) was obtained from Esstech. (Essington, PA). The inhibitor was removed from IBMA by treatment with activated basic alumina (Brockman I, Sigma-Aldrich). AIBN was recrystallized from methanol. All other materials were used as received.
2.2
Degradable disulfide dimethacrylate synthesis
Disulfide dimethacrylate (DSDMA) was synthesized according to a prior literature report . Methacryloyl chloride (5.64 g, 36.5 mmol), TEA (11.09 g, 109.6 mmol) and DMAP (0.45 g, 3.65 mmol) was dissolved in 150 ml anhydrous dichloromethane (DCM). The solution was purged with nitrogen gas and cooled by ice bath for 30 min. 2-Hydroxyethyl disulfide (5.64 g, 36.5 mmol) was added to the solution in a dropwise fashion. The reaction mixture was allowed to warm to room temperature under nitrogen and held for 24 h. Afterwards, the reaction was quenched by addition of deionized water and subsequently washed twice with a DI H 2 O, Na 2 CO 3 (10%), brine solution. The organic phase was dried over Na 2 SO 4 and the solvent removed under vacuum. Yellow oil was obtained in 80.2% yield. The monomer structure was verified by 1 H NMR (400 MHz, chloroform- d ): δ (ppm) 6.15–6.14 (m, 1H), 5.69–5.54 (m, 1H), 4.43 (t, J = 6.6 Hz, 2H), 2.99 (t, J = 6.6 Hz, 2H), 1.96 (dd, J = 1.6, 1.0 Hz, 3H).
2.3
Polymer and nanogel preparation
A linear polymer of isobornyl methacrylate (PIBMA) was synthesized in the presence of 15 mol% of AMSD (relative to IBMA content) with 1 mol% AIBN in ethyl acetate (1:1 by weight) as solvent. Polymerization was carried out at 80 °C for 24 h in a nitrogen environment with 82.3% vinyl group conversion as determined by FT-IR. The homopolymer sample was isolated by precipitation from an excess of methanol with the residual solvent removed under vacuum to obtain the final oligomeric material as a powdery solid.
A linear block copolymer was then synthesized from the reaction of the isolated PIBMA with benzyl methacrylate (BzMA) using AIBN (0.5 mol% based on IBMA repeat units). The molar ratio of BzMA monomer to IBMA units in PIBMA was 2:1. A four-fold excess of ethyl acetate was used and the reaction was conducted for 12 h at 80 °C under nitrogen. With the BzMA vinyl group conversion at 80.4%, the copolymer was isolated by precipitation from methanol to yield a powdery solid material.
A degradable nanogel was prepared by the copolymerization of DSDMA and IBMA (30:70 mole ratio) with 15 mol% AMSD and 1 mol% AIBN (related to total monomers) in a four-fold excess of ethyl acetate. Polymerization was carried out at 80 °C for 24 h in a nitrogen environment with 81.4% final vinyl group conversion. Samples were taken at various intermediate time points with the nanogels being isolated by precipitation from an excess of methanol and then dried under vacuum to obtain a pale yellow powder.
2.4
Disulfide nanogel degradation
Nanogel prepared with disulfide dimethacrylate (0.10 g, about 0.15 mmol disulfide functionality) was dissolved in inhibitor-free tetrahydrofuran (THF; 6 ml). DTT (0.15 g, 1.0 mmol) was added under a nitrogen environment. The reaction was allowed to proceed at room temperature and aliquots of samples were withdrawn periodically and immediately diluted in THF for GPC analysis.
2.5
Non-degradable nanogel synthesis
Two additional nanogels were synthesized without the cleavable disulfide crosslinks. UDMA and IBMA were mixed at 10:90 or 30:70 molar ratios and 15 mol% of AMSD and 1 mol% of AIBN (relative to monomers) was added with a four-fold excess of ethyl acetate. Polymerization was continued for 4 h at 80 °C under a nitrogen environment. Samples were precipitated from an excess of methanol and dried under vacuum to obtain white powdery materials. The conversion of vinyl groups was 48.4% and 38.5%, respectively, for RN19 and RN37 (RN refers to reactive nanogel, and the numbers indicate the crosslinker to monomer molar ratios).
RN19 and RN37 were added to TEGDMA in mass ratios of 10–40% in 10% increments. DMPA as photo initiator (0.5 wt%, relative to TEGDMA) was added to each sample. Unfilled TEGDMA with DMPA was used as control system. Nanogels were fully dispersed into TEGDMA and formed stable, transparent mixtures following mechanical agitation.
2.6
Polymer structural analysis
GPC (Waters 515) was employed for the analysis of linear polymer and nanogel molecular weight and polydispersity index (PDI) based on polystyrene calibration standards. Tetrahydrofuran was used as eluent at a flow rate of 1 ml/min at 35 °C. A 400 MHz NMR (Bruker Avance-III 400) was used for collection of 1 H spectra of the monomers as well as polymeric structures in either CDCl 3 or CD 2 Cl 2 .
2.7
Photopolymerization kinetics
Real-time near-infrared (NIR) spectroscopy was applied to monitor the reaction kinetics of the TEGDMA-dispersed nanogel formulations during the photopolymerization process. The methacrylate group ( CH 2 ) has an overtone absorbance peak at 6165 cm −1 , which was monitored throughout the reaction. All specimens ( n = 3) were irradiated under 365 (±10) nm UV light with a 10 mW/cm 2 irradiation intensity as determined by radiometer at the sample surface position.
2.8
Volumetric polymerization shrinkage
A non-contact linear variable differential transducer (LVDT)-based linometer (Academic Center for Dentistry Amsterdam, The Netherlands) was used for real-time volumetric shrinkage testing. Samples (compressed cylindrical shape of 3–4 mm diameter and 1 mm thickness) were placed on an aluminum disk with a glass slide covering the top. A thin film of applied grease on the aluminum disk and glass slide substrates allowed the sample to shrink freely in the horizontal as well as vertical dimensions. The transducer underneath the aluminum disk monitored the z -axis linear displacement of the sample during polymerization that was then used to calculate the real-time volumetric shrinkage. All specimens ( n = 3) were irradiated under 365 (±10) nm UV light with 10 mW/cm 2 irradiant intensity with limiting shrinkage data reported at the end of the 10 min illumination interval.
2.9
Shrinkage stress measurement
A tensometer (Volpe Research Center, American Dental Association, Gaithersburg, MD) was utilized for measurement of real-time polymerization shrinkage stress . All cylindrical samples (6 mm diameter and 1 mm thickness) were placed between two polished, silane-methacrylate-treated quartz rod surfaces (6 mm diameter) where the top rod was attached by a collet connected to the stainless steel beam of the cantilever tensometer. The bottom glass rod, which was fixed to the tensometer base with a second collet, was also used as a light guide extension for photocuring with an adapter connecting the tip of the UV light guide with the rod. Specimens ( n = 3) were irradiated under 365 (±10) nm UV light at intensity of 10 mW/cm 2 (measured by radiometer at the exit of the glass rod) for 15 min. The deflection of the calibrated cantilever beam was monitored by a LVDT detector with displacement converted to the shrinkage stress output.
2.10
Mechanical property testing
Flexural mechanical properties were collected by a universal testing machine (Mini-Bionix II, MTS, Eden Prairie, MN). Specimens with dimensions of 25 mm × 2 mm × 2 mm were cured under 365 (±10) nm light irradiation for 25 min with 5 mW/cm 2 intensity. Flexural modulus and strength were determined in three-point bending tests on a 20 mm span at a crosshead speed of 1 mm/min after approximately 48 h storage in the dark at room temperature. Vinyl group conversion for the mechanical testing samples ( n = 3) was measured with NIR shortly after the photopolymerization.
A dynamic mechanical analyzer (DMA, Perkin Elmer 8000, Waltham, MA, USA) was employed to measure the bulk nanogel glass transition temperature ( T g values by sandwiching 10 mg of nanogel powder in aluminum pockets. A single cantilever cyclic displacement at 1 Hz was applied while the sample ( n = 3) was heated to 180 °C and then cooled back to room temperature at 2 °C/min with tan δ data collected during the cooling process. The T g was assigned as the maximum in the tan δ output. This technique has been validated using film and powdered polymer standards of known T g .
A TA Q800 DMA was applied for measuring the storage moduli ( G ′) and tan δ behavior of fully-cured nanogel-modified compositions and the TEGDMA homopolymer control. Samples were prepared under the same curing conditions as employed with the flexural strength testing; however with different specimen dimensions of 25 mm × 3 mm × 1 mm. The photocured specimens ( n = 3) were post-cured at 160 °C overnight to reach essentially full conversion (>98%) to avoid any post-cure effects during the thermal analysis testing, which utilized a tensile test mode with a strain rate and frequency of 0.02% and 1 Hz, respectively during the temperature scan from 0 °C to 220 °C at 2 °C/min.
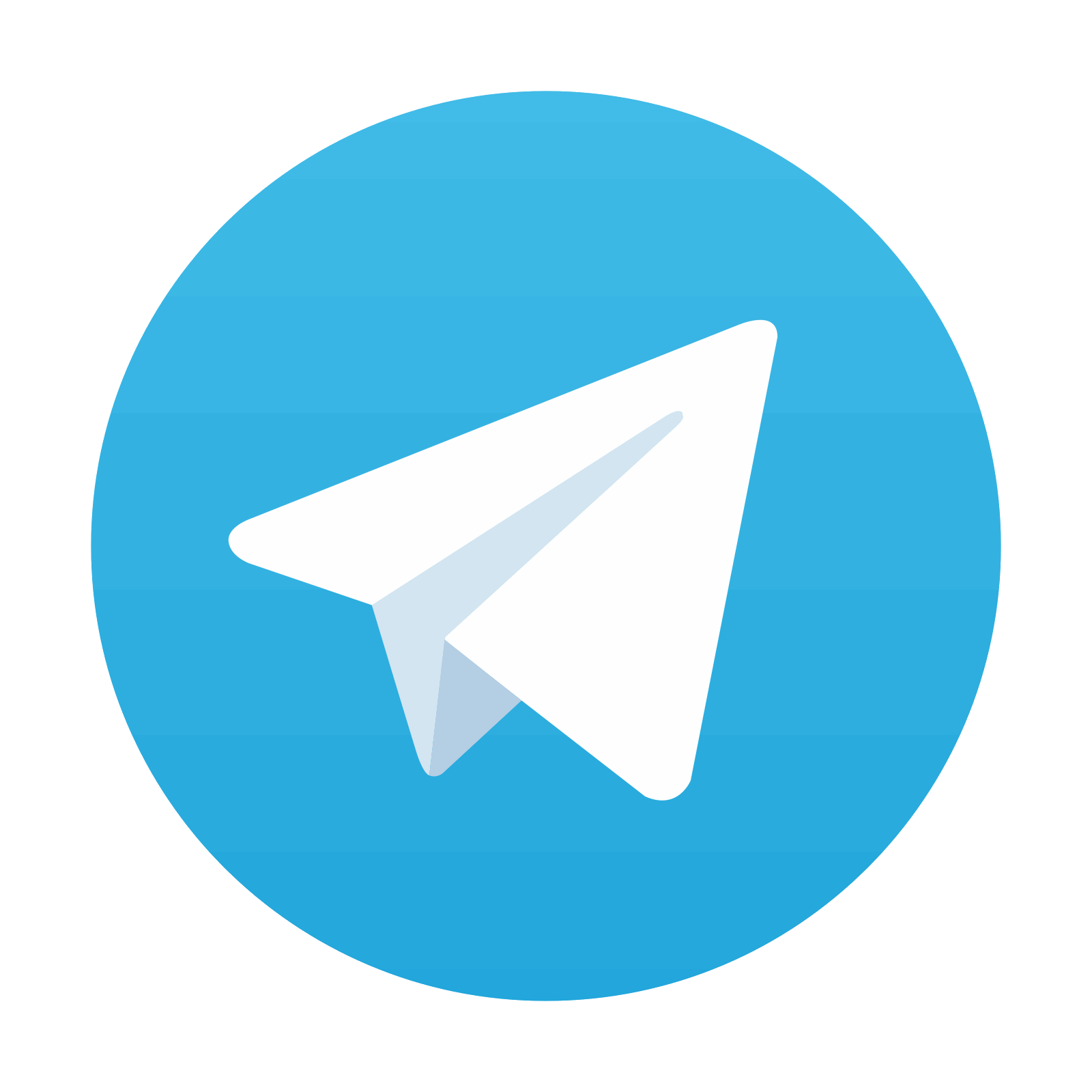
Stay updated, free dental videos. Join our Telegram channel

VIDEdental - Online dental courses
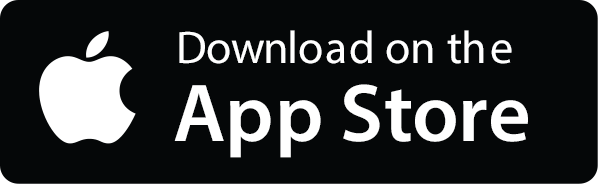
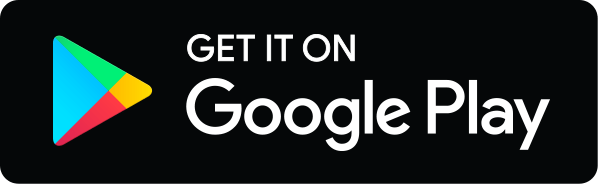