19
Prosthetic Rehabilitations to Optimize Maintenance and Implant Long‐term Success
Silvia Brandt
Department of Prosthodontics, Center for Dentistry and Oral Medicine (Carolinum), Goethe University Frankfurt, Frankfurt am Main, Germany
Introduction
Decisions between fixed and removable implant–supported restorations are usually governed by clinical considerations (anatomy and esthetics). Financial constraints and patient requests may also enter the equation, and there is a growing tendency for sociodemographic aspects to become relevant in selecting treatment modalities [1, 2]. Due to a rapidly changing population, dental clinicians in the United States will be treating an ever‐increasing proportion of older patients, whose percentage in the age range of 65 and over is expected to increase from currently 16.5% to almost 20% by the late 2020s and to 25% by 2060 [1, 3]. Dental clinicians will have to develop treatment concepts in line with the requirements and limitations of this continuously growing population. This particularly applies to implant dentistry, where the goal is to achieve high success rates combined with low complication rates. Advanced fabrication methods – such as computer‐aided design and manufacturing (CAD/CAM) technology – have increased the precision and time efficiency of achieving treatment goals. At the same time, there is a mandatory need to ensure that the resultant prostheses can be readily handled by patients whose motor abilities will foreseeably decrease with age. There is also a need, notably, with a view to the longevity of dental implants, that good cleansability of the superstructures by routine self‐performed hygiene is ensured. With a view to these manipulation and maintenance requirements, implant–supported removable prostheses are considered beneficial, but attention should also be devoted to their attachment systems [4, 5].
Attachment Systems for Removable Superstructures
Different attachment systems are the main distinguishing feature between implant–supported removable superstructures. Two major subtypes exist: freestanding (unsplinted) and splinted systems. All of these have in common that they comprise a patrix (male) and a matrix (female) component, the latter forming an integral part of the superstructure and acting as a receptacle, the former being connected to the implant and engaging them [6]. Freestanding systems notably include ball, locators, magnetic, and telescopic attachments. Splinted systems may take the form of either flexible (cast) or rigid (milled) bar attachments (Figure 19.1).
Long‐term Evaluation
For all these attachment systems, adequate outcomes are documented with regard to function, esthetics, and “oral health‐related quality of life” (OHRQoL) [7, 8]. They have been shown to offer high rates of five‐year overall survival ranging from 95 to 100% [5, 9]. While a complete assessment of long‐term prognoses will also, of course, have to consider the risk of complications, a uniform approach to classifying complications of implant–supported removable prostheses does not currently exist. Back in 2001, Payne et al. [10] proposed that a distinction should be made between routine maintenance performed during periodic recall visits on the one hand, and repair or corrective maintenance requirements on the other. Reports in the literature actually do tend to differentiate between technical complications (often used interchangeably with mechanical complications) and routine maintenance requirement.

Figure 19.1 Unsplinted Attachment systems for implant–supported removable superstructures.
Complications and Maintenance
Technical complications in the sense that patrix or matrix components need to be activated, replaced, or repositioned and are the most common type of intervention with implant–supported removable prostheses [11–14]. While ball attachments seem particularly susceptible to requiring such interventions notably within the first year of delivery, these inventions do not translate into a significant difference in overall comparisons between freestanding ball or Locator attachment systems [14, 15]. Within splinted systems, a difference has been reported in favor of rigid (milled) bars, where significantly fewer interventions were required for the retentive part [14].
Inconclusive rates are documented for screw (including abutment screw) loosening or fracture. On one hand, all attachment systems appear to require interventions for these events similarly often [13]. On the other, the incidence of this complication with maxillary prostheses has been reported to be especially high for bar attachments [12].
The risk of prosthetic fractures – including failure of a resin tooth or the denture framework – is influenced by how the restoration has been fabricated in the dental laboratory. Current evidence in the literature indicates a higher rate of technical complications associated with the denture itself for maxillary restorations that do not feature a metal reinforcement or coverage of the palate [12, 16]. Another modifier of intervention rates is for how long a denture has been worn. Systematic reviews have unanimously disclosed that complications increase after a five‐year period [12, 13].
How to Select an Attachment System?
A variety of parameters come into play in deciding which attachment system should be selected in each patient. What follows is a short discussion of three diagnostic levels from which the decision should eventually be derived.
Decision Level 1: People‐related Factors
At this level, the clinician and the patient are the main two factors contributing to the decision. Other modifiers include the clinical and technical expertise of the entire team, the patient’s desires and expectations, and how much he or she can afford to pay for the treatment. Selectable attachment systems may also be limited by health‐related issues, most notably regarding the patient’s motor abilities.
Decision Level 2: Tissue‐related Factors
This level, too, combines various aspects to be considered in the decision‐making. Most importantly, these include the existing prosthetic space, number of implants, inter‐implant distance, implant angulation, and implant position. Of particular note in this context, removable prostheses are generally considered to involve greater horizontal and vertical space requirements than fixed prostheses. It is mandatory to make sure in the diagnostic phase that adequate space will be present for both the attachment and its housing, as well as for the denture itself. In the literature, vertical dimension requirements of 7 or 11 mm have been reported for freestanding or splinted (i.e. bar) attachments, respectively [17]. These values should be regarded as the minimum height capable of avoiding undersized components (Figure 19.2).

Figure 19.2 (a) and (b): Intraoral view of a case where inadequate space resulted in undersized prosthetic components, thereby causing fracture of the denture and loss of the matrix component.

Figure 19.3 (a) and (b): Intraoral views of differently designed full‐mouth restorations with double crown attachments. Those on the left are designed as removable dentures, while those on the right are similar to fixed prostheses.
Decision Level 3: Simulation‐related Factors
Factors related to the quality of simulation need to enter the decision process as well. They are not only concerned about the question if palatal coverage is required, but also about the dimensions of the future prosthesis. Whenever coverage of the palate is not tolerated by a patient, inserting an adequate number of implants will eliminate this need. Four or more implants are required to this end [18]. The use of double crowns for attachments is considered beneficial by allowing for substantially reduced dimensions of the prosthesis. Experience has shown that implant–supported removable prostheses of this type, similar in design to fixed bridgework, meet with high patient acceptance (Figure 19.3).
This author believes that an ideal attachment system, which will reliably meet a given patient’s individual requirements, can only be selected by taking a synoptic view (Figure 19.4).
Prostheses Retained by Double‐crowns
Of the aforementioned retention systems for implant–supported removable prostheses, the variant of double‐crowns will be discussed in depth for the remainder of this chapter. Compared to other freestanding systems, this attachment mechanism has several distinguishing features and is extremely versatile.

Figure 19.4 Overview of modifiers to be considered in selecting an ideal attachment system.
History of Telescopic Crowns
Telescopic crowns were invented for fixed dental prostheses. Their use was first described by a Philadelphian dentist named R. W. Starr in 1886 [19]. Initially used for bridgework segmentation, they were later harnessed for removable prostheses.
The conventional use for double‐crown telescopes is to retain dentures on residual teeth. A telescopic double crown is made up of a primary (internal) crown fixed to a natural tooth or implant abutment, and a secondary (external) crown, which, being a rigid component of the denture framework, forms an integral part of the prosthesis.
Friction‐type (Cylindrical Crown) Telescopes
These telescopes are based on a primary crown milled to 0−2° of parallelism, which slides into the secondary crown much like a plunger into a cylindrical receptacle, having contact with its wall circumferentially. Retention is thus achieved by friction, i.e. by the frictional resistance developing between the two contacting surfaces. The original design of these friction‐type telescopes, and their further development into parallel attachments and bars, dates back to Karl Häupl und Hermann Böttger [20].
Resilience‐type (Clearance Crown) Telescopes
This non‐friction variant of telescopic double crowns was introduced by Manfred Hofmann in 1966. Here retention is achieved mainly by mucosal support. Given around 0.5 mm of occlusal clearance between the primary and secondary crowns, retention develops not immediately but after mucosal resilience is established [20]. In European countries, these prostheses are known as “cover dentures.” Good results with this system have been obtained mainly in the presence of up to three natural tooth abutments. Resilience‐type telescopes are not used in implant dentistry.
Cone‐type (Conical Crown) Telescopes
In 1968, Karl‐Heinz Körber worked on refining the friction‐type telescopes by developing a primary crown no longer cylindrical in shape, but featuring a taper of 4°–8°, which reduced frictional resistance to telescope closure [20]. Depending on the convergence angle of the primary crown, either a retentive or a supportive function is accomplished. Smaller angles translate into more retention, the strength of which is also influenced by different surface characteristics and materials. A high‐gold alloy has proven to be the material of choice for the components of this system (Figure 19.5).

Figure 19.5 Different primary crowns used in double crown attachment systems.

Figure 19.6 (a) and (b): Principle of the “tribological system” according to Weigl et al. [21].
Gold‐on‐zirconia Double Crowns
This system also uses a conical primary crown with a defined cone angle of 2° but with a high‐strength oxide ceramic as the primary crown material and a secondary crown made of electroformed gold. This principle was developed and introduced by Paul Weigl at the turn of the millennium, has entered the daily clinical practice with the introduction of advanced CAD/CAM technology, and is today widely accepted. This system, also known as the “Frankfurt principle,” does not rely on friction between the patrix and matrix components for retention (which therefore remains unaffected by intraoral service). Rather, the retention is accomplished tribologically, with the surfaces interacting in relative motion via a gap of 5 μm that is created between the primary and secondary crowns. In contact with saliva, this feature serves as a capillary gap allowing cohesive forces to develop that will ensure continuous retention of the prosthesis. The design and material selected for the primary crowns are also beneficial, as zirconia minimizes the “demasking” effect and given a predefined vertical height of at least 7 mm, acts as a rest seat allowing for a delicate removable prosthesis similar to what would normally be the design of a fixed prosthesis. In addition, primary crowns of zirconia offer more stable retention than crowns made of gold alloy (Figure 19.6) [5,21–23].
To summarize, a variety of double‐crown telescopic systems are available, all of them having their own strengths and weaknesses. The characteristics of the most widely used systems are listed in Table 19.1.
The “Frankfurt Principle”
Indications and Limitations
A challenge routinely posed by severely reduced dentitions is for the clinical team and the patient to decide whether the proposed rehabilitation should incorporate any compromised residual teeth or should be supported and retained exclusively by implants. While there is never going to be a universally applicable solution to this dilemma, the concept of telescopic retention by double crowns, notably in accordance with the “Frankfurt principle,” does offer several advantages in prostheses combining both existing teeth and strategically inserted implants.
Combinations of tooth and implant abutments are known to offer mutual stabilization. While the tactile sensitivity of natural abutments, given intact periodontal receptors, will counteract any excessive masticatory forces, the much higher stability of the implants will protect the compromised abutment teeth from excessive loading [25]. The goal is to achieve total integration of abutments, i.e. of all residual natural teeth with all implants placed. As an additional consideration, the prosthetic usefulness of each potential abutment tooth should be evaluated in advance based on periodontal, endodontic, and reconstructive criteria [26, 27]. Its suitability for a hybrid prosthesis of this type can be derived from the result of this evaluation outlined in Table 19.2.
Table 19.1 Double‐crown telescopic systems, overview modified and expanded.
Source: From Lehmann and Gente [24].
Cone‐type (conical crown) telescopes | Gold‐on‐zirconia telescopes (Frankfurt concept) | Friction‐type (cylindrical crown) telescopes | Resilience‐type (clearance crown) telescopes | |
---|---|---|---|---|
Retention mechanism | Wedging | Adhesion | Friction | No inherent retention |
Function for prosthesis | Retention, support, guidance, indirect‐retainer function | Retention, support, guidance, indirect‐retainer function | Retention, support, guidance, indirect‐retainer function | (Support), guidance Indirect‐retainer function |
Shape of primary crown (patrix component) | Conical (tapered) | Conical (tapered) | Cylindrical (parallel‐walled) | Parallel along marginal third |
Convergence angle α | 4° < α < 8° | 2° | 0°–2° | 0°–2° |
Fit | Defined transition fit | Precision fit (<5 μm) | Transition fit | Clearance fit |
Prosthetic design | Various | Bridework‐style or with a denture base | 1–3 tooth abutments, functional margin (usually) interrupted in the abutment area | *Cover denture*, continuous functional margin |
Prosthetic retention | Via the matrix component (secondary crown) | Denture base with functional margin design | ||
Double crown support | Yes Matrix makes positive (or near‐positive) contact with patrix | No. Distance between matrix and patrix equals mucosal resilience | ||
Prosthetic support principle | Immobile seating | Mobile seating | ||
Indications | Tooth‐ and/or implant–supported dentures, tooth‐ and mucosa‐supported dentures | Dentures supported mainly by mucosa (secondary support function possible) |
* Cover Denture = resilience‐type telescopes
Another important advantage of these restorations is that they can potentially survive any loss of one or several tooth abutments. In a scenario like this, the prosthesis will usually continue to serve its purpose. Later, an additional implant can be inserted to re‐establish stability at the site of the lost abutment tooth.
A distinctive feature of the Frankfurt treatment protocol is to fabricate and provide these patients with a “travel denture” (described in detail later in this chapter). As a result, the patient can resort to an acceptable copy of the definitive denture whenever the need arises, so that any maintenance and/or repair jobs can be conducted in the laboratory as required without creating an unpleasant waiting period for the patient.
Compared to fixed restorations, it is easier to maintain high levels of plaque control with a prosthesis that can be removed by the patient at all times. Compared to dentures retained by splinted bars, it is also much easier for the patient to maintain self‐performed hygiene of the freestanding implant–supported crowns serving as primary telescopes. One potential limitation to keep in mind, however, is the long‐term prognosis of the patient’s general health, including his or her motor abilities (Figure 19.7 and 19.8).
Correct implant positioning is essential to the long‐term success of the prosthesis and, therefore, should be driven by the design of the future prosthesis, to avoid any malpositioning that might cause deviations from the uniform orientation that is needed for all abutments to properly insert into the secondary crowns. These requirements may necessitate a more extensive surgical approach, which, in turn, might exceed the patient’s willingness to accept risks or be contraindicated in the presence of health problems. While few compromises are acceptable with regard to the position, length, and angulation of implants for fixed prostheses, there is nothing wrong with selecting between several options of implant positioning for removable prostheses to strike an appropriate balance between the extensiveness, cost, and benefit of treatment. For instance, major surgical procedures like bone augmentation can often be avoided or their invasiveness reduced without repercussions on the function or esthetics attainable by prosthetic treatment. An effort should be made, however, to achieve an abutment distribution as quadrangular as possible for optimal support of the prosthesis. Pertinent follow‐up examinations have demonstrated a statistically significant difference between the success rates offered by quadrangular versus both triangular and linear transverse distributions, in the absence of a difference between these latter two support distributions (Figure 19.9) [5].
Table 19.2 Suitability of natural teeth for use as abutments, modified and expanded.
Source: From Zitzmann [26] and Naumann [27].
![]() |
![]() |
![]() |
|
---|---|---|---|
Suitability of natural teeth for use as abutments | |||
Treatment goal | Tooth should be preserved | Tooth should be pretreated for a safe prognosis | Tooth should be extracted |
PERIODONTAL criteria | Safe | Uncertain | Hopeless |
|
|
|
|
|
|
|
|
ENDODONTIC criteria | Safe | Uncertain | Hopeless |
|
|
|
|
|
|
|
|
RECONSTRUCTIVE criteria | Safe | Uncertain | Hopeless |
|
|
|
|
|
|
|
|
|
|
|
|
|
|
|
Also, removable restorations supported by implants allow for better flexibility in optimizing the prosthetic design for aesthetics and function. The denture‐mucosa interface can be positioned more freely, and the tooth axes more readily corrected. Occlusal relationships can be transformed or adjusted in the vertical and horizontal planes with modest effort. Limitations of these prostheses, notably when natural tooth abutments are to be incorporated, arise from the total space requirements for a primary crown, secondary crown, tertiary structure, and veneer layer. Also, preparing natural teeth with abrasive instruments for use as abutments is somewhat invasive. Esthetic limitations may arise from a need for over‐contoured prosthetic restorations notably in the anterior segment of the maxilla or mandible. Giving due consideration to all these limitations in the planning stage is absolutely required.

Figure 19.7 Reinforcement of oral‐hygiene training for cleansing of freestanding primary crowns required by dental providers. These ceramic primary telescopes had been in situ for eight years.

Figure 19.8 Abutment distributions as classified by Steffel [28]. (a) punctiform support. (b) linear sagittal (unilateral anteroposterior) support. (c) linear transverse support. (d) linear diagonal support. (e) triangular support. (f) quadrangular support.
The treatment and fabrication process are more complex for hybrid prostheses than for other restorations. A team approach to planning is required, followed by close coordination of the successive clinical and laboratory steps. Meticulous care should be devoted to “passivation,” which is required to compensate for the physiological mobility of natural tooth abutments as compared to the relative rigidity of osseointegrated implants. The process of laboratory fabrication, too, is technique‐sensitive and should be performed by a considerably experienced and skilled technician.
Clinical and Technical Process
The rest of this chapter will be dedicated to case reports illustrating the chairside and laboratory workflows for prostheses retained by gold‐on‐zirconia double crowns.

Specific Workflow Considerations
Primary and Secondary Crowns
Using CAD/CAM technology, the primary crowns are milled from zirconia, an oxide‐ceramic material known for its high strength. Based on an esthetic try‐in (mock‐up) of a digitized denture setup, a backward‐planned design process is carried out first for ideal abutment shapes and then for the primary crowns. Once milled, these primary crowns are then processed using diamond finishing instruments without polishing.


Workflow: figures 1–6.
A milling device is used to create the contact surfaces of the conical crowns. Care should be taken to ensure smooth surfaces, as any tiny “dent” will be replicated in the process of electroforming the matrix component, which then, in the process of being dislodged from the conical crown, is bound to expand by the amount of this dent, thus compromising the high precision of fit. Any polishing will indent these smooth surfaces in ways not always visible to the naked eye. Therefore, following processing in the milling unit, the conical crowns should never be polished.

Workflow: figures 7 and 8
The secondary crowns, or matrix components, are created directly on the surface of the conical primary crowns using a fully automated process of electroforming by deposition of fine‐gold particles. Thus, rather than duplicating the primary crown for indirect forming of the matrix component, the internal surface of the fine‐gold coping is directly defined by the external surface of the conical crown. To render the surface conductive for electroforming, the conical crown is covered with a very thin layer of silver varnish by air‐spraying, which also creates a separating layer allowing the electroformed coping, once finished, to be dislodged from the conical crown. The layer thickness (and especially evenness) of conductive silver varnish is critical for an accurate fit of the electroformed matrix component. Given a correct procedure, a 5 μm fit is created between the primary and secondary crown.

Workflow: figures 9−12: Fabrication of the secondary crown by deposition of fine‐gold particles.

VIDEdental - Online dental courses
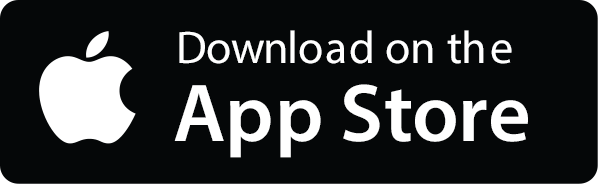
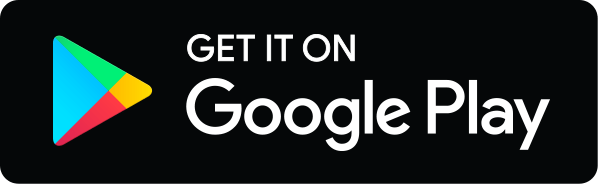