7
Prosthetic Abutment Characteristics and Implant–Abutment Maintenance
Rafael Delgado‐Ruiz1 and Georgios E. Romanos2
1 Department of Prosthodontics and Digital Technology, Stony Brook University, Stony Brook, NY, USA
2 Department of Periodontics and Endodontics, Stony Brook University, Stony Brook, NY, USA
Introduction
An implant abutment is defined by the glossary of prosthodontic terms as “that portion of a dental implant that serves to support and/or retain a prosthesis” [1]. It is also defined by Giglio [2], as an intermediate component connecting the implant and the restoration [2], its retained to the implant with a screw (abutment screw) [1] and retains the implant crown with another screw (prosthetic screw) [3] in screw‐retained restorations, or with cement in cement‐retained restorations [1–3]. In addition, the prosthetic abutments of certain implant systems are retained by friction to the implant connection without a screw [1–3].
Considering the number of parts involved in the implant–abutment‐restoration, a system of tiers (two and three) was created [2]. Tier‐2 indicates that the abutment and the crown are a single piece retained to the implant or that the implant and the abutment are a single piece and the crown is retained to it. Meanwhile, in tier‐3 three interconnected pieces conform the system: the implant, the abutment, and the crown [2].
In addition to retaining an implant restoration, implant abutments can have different purposes. For example, healing abutments are used during the healing phase on single‐stage implants to assist tissue healing or to modify tissue contours [1]. Implant–angulated abutments are used to correct the implant angulation in case of positional errors, and other abutments are used to support temporary restorations until the complete integration of the implants [1]. Once the osseointegrated implants are restored, the maintenance of the implant restoration is essential to reduce the plaque deposits and their subproducts thus, preventing peri‐implant diseases [4].
Different aspects of the design of implant restorations that compromise cleanability have been presented as well as some methods to reduce plaque adhesion and enhance cleanability [5–10].
For example, Walton et al. [5] described that fixed implant restorations were harder to clean compared to removable implant–supported restorations [5]. O’Mahoney et al. [6] analyzed failed implants and found plaque deposits in all the implant–abutment‐restoration interfaces, the prosthesis surface, and the implant neck [6]. Teughels et al. [7] found that the transmucosal region of various materials developed robust and thicker biofilms when their surfaces were rough [7]. Zaugg et al. [8] demonstrated that hydrophilic and rough surfaces accumulate considerable amounts of biofilm and the topography influenced the cleanability of the surface [8]. Mazjoub et al. [9] found that larger emergence angles of the restoration resulted in higher marginal bone loss [9]. Finally, Chanthasan et al. [10] demonstrated that the interproximal design of single implant–supported posterior crowns influences the amount of food impaction and the health of the peri‐implant gingival tissues [10] (Table 7.1).
Biofilm formation within the oral cavity cannot be eliminated and plaque deposits will evolve and mature over time on all hard dental and prosthetic implant structures if the patients cannot access hidden aspects of the implant restorations [11, 12]. Dab [13] described two aspects that should be considered in implant suprastructure designs: the running room (distance of the most apical portion of the interproximal contacts to the implant platform) and the ridge dimensions. Together, these two parameters dictate the best implant diameter and insertion depth to achieve the ideal emergence angle with the lowest resorption rates of the peri‐implant marginal bone [13].
Table 7.1 Design parameters of implant restorations and their influence on the formation of biofilm and plaque.
Author, year, type of study | Number of patients | Type of prostheses/comparisons | Problem | Solutions |
---|---|---|---|---|
Walton et al. 1994 [5]. Retrospective. |
156 patients | Fixed versus Removable | For both types of restorations patients complained about cleanability. Fixed implant – supported restorations more difficult to clean. |
Inform patients about limited cleanability Education on maintenance methods |
O’Mahony et al. 2000 [6]. Retrospective. |
40 patients | Fixed prostheses (single implant crowns and implant supported bridges). | Micro‐gaps at different levels between implant and abutment and restoration and abutment. Plaque accumulation at the transmucosal surfaces with restricted access produced by over contoured restorations. Plaque accumulation at the surface of the restorations. Plaque accumulation at the implant surface. General increased surface roughness. |
Reduce micro‐gap and enhance parts assembly protocols. Prosthetic designs that minimize plaque retention and improve emergence profiles. All components exposed to oral environment should possess smooth surfaces. |
Teughels et al. 2006 [7]. Systematic review. |
36 Clinical studies were included. The studies were completed within the oro‐pharyngeal region to evaluate the surface properties /biofilm formation on supra and subgingival hard structures. |
Clinical studies evaluating the: biofilm formation on teeth, restorations, abutments, or implants, surface characteristics, and also plaque formation. | Rough surfaces (Ra >2 μm) of abutments, crowns and overdentures present larger plaque deposits with increased spirochetes, rods, and motile organisms. Increased inflammatory infiltrate, crevicular fluid, and bleeding. Material chemistry also influenced the biofilm formation. |
Roughness values Ra ≤ 0.2 μm reduce plaque accumulation. Materials with low surface energies recommended to reduce biofilm formation. |
Zaugg et al. 2017 [8]. Comparative In vivo study. |
16 patients | Studied biofilm formation on disks (made in four materials and different surfaces) bonded to intraoral splints
|
Hydrophilic and rough surfaces increased biofilm formation. The topography of the surface influenced the cleanability. Demonstrated by bigger amounts of biofilm on micro‐grooved surfaces. There are inter and intra‐individual differences in biofilm formation that made difficult other comparisons. |
Machined surfaces facilitate cleaning. |
Mazjoub et al. 2021 [9]. Retrospective study. |
63 patients | Studied radiographically the restoration emergence angle of patients with bone‐level implants and other parameters like age, implant length and diameter, type of restoration (cement or screw‐retained), internal screw length, and maintenance visits. | Increased marginal bone loss (up to 4 mm) in restorations with angles >30° The marginal bone level was found 1 mm apical to the end of the abutment screws in 63% of the patients. |
Not presented |
The restoration angle was evaluated between a line parallel to the coronal third of the implant and the angle of the restoration. The length of the screw measured from the platform level to the apical end of the abutment screw. |
||||
Chanthasan et al. 2022 [10]. Cross‐sectional. |
178 patients | Studied the food impaction at single posterior implant crowns Used clinical parameters (papilla height, open contacts determined by flossing, food remnants) and radiographic parameters (contact length and contact point level). | The presence of interproximal open contacts resulted in 66.9% patients with food impaction between the implant crown and the adjacent teeth. Increased plaque accumulation and deeper pockets present in open contacts areas. |
The following parameters length of the contact area, level of the contact point, and dimensions of the embrasure area should be controlled. |
In addition to the previous parameters described by Dab [13], the transmucosal portion of the prosthetic abutment is critical in preventing peri‐implant diseases [14]. Therefore, the characteristics of prosthetic abutments including composition, surface, coating, design, emergence profile, and their influence on cleanability and maintenance, require a detailed analysis [14].
The goal of this chapter was to review the characteristics of the tissue interfaces around prosthetic abutments, describe the influence of prosthetic abutment material, surface characteristics, presence of coatings, design and emergence profile, and other variables in implant maintenance. This chapter is supplemented with illustrations, clinical photos, and recommendations for improving prosthetic abutments that can reduce peri‐implant diseases by enhancing their maintenance.
Tissue Interfaces Around Prosthetic Abutments
The body of the prosthetic abutment consists of three parts: the connection, the transmucosal area, and the retentive walls. Its vertical dimensions, from the top to the base, encompass the length of the walls, the transmucosal area, and the connection length (Figure 7.1).
The abutment connection stabilizes the prosthetic abutment within the implant, prevents its rotation, seals it against bacteria colonization, and transfers loads to the implant [15, 16]. The transmucosal portion of the prosthetic abutment pierces the mucosa. It elevates the abutment/restoration micro‐gap away from the bone, provides support to the soft tissue contours and the establishment of a soft tissue cuff (primary barrier to bacteria penetration), and participates in forming the emergence profile of the restoration [17, 18]. The prosthetic abutment walls support the implant restoration, and incorporating different designs, grooves, wall angles, and cement, can increase the retention of cement‐retained implant crowns [19–21].
The amount and hard or soft tissues in contact with prosthetic abutments depends on the implant insertion depth (subcrestal level, bone level, or tissue level), the prosthetic abutment height, the initial soft tissue thickness and previous soft/hard tissue grafts, and tissue recession [22, 23].
For example, in implants deeply inserted in bone (subcrestal implants), the prosthetic abutment may contact the cortical bone and all the soft tissue thickness. In addition, the restoration base will be in contact with soft tissues. Meanwhile, in supracrestal implants, the prosthetic abutments will have only contact with the soft tissues, and the crown will have some soft tissue contact. In bone‐level implants, the prosthetic abutment will be in contact mainly with the soft tissues, although some contact with bone can exist in platform‐switched abutments [24]. The prosthetic abutments’ length will require adjusting with long transmucosal portions in subcrestal implants. Meanwhile, supracrestal implants will require prosthetic abutments with shorter transmucosal portions (Figure 7.2).

Figure 7.1 Illustration of a conventional prosthetic abutment for a single implant for a cement‐retained restoration. Three areas can be identified: light blue area represents the walls, the dark blue area represents the transmucosal portion (collar), and the grey area, the connection. The sum of all areas constitutes the abutment length.

Figure 7.2 Effect of the implant insertion depth on the dimensions of the transmucosal portion of prosthetic abutments.
Characteristics of the Soft Tissue in Contact with the Prosthetic Abutments
Donley et al. (1992), reported that the epithelia of the soft tissue are attached to the titanium oxide layer of the abutment surface, by a layer of glycoproteins (laminin and fibronectin) of 20 nm thickness, and described that the connective tissue fibers run parallel to the abutment surface. However, the abutment’s surface properties and geometry can change the fibers’ orientation [25]. In addition, Berglundh et al. [26] found that the marginal implant mucosa around the titanium surface contains a collagen/fibroblast ratio of 109 versus a collagen/fibroblast ratio of 4 in gingival tissues of teeth in canines [26].
Iglhaut et al. [27], completed an exhaustive review of the parameters and differences that can affect the attachment and apical migration of the gingival epithelia on implant abutments [27]. Their findings can be summarized as follows:
- The peri‐implant soft tissues are larger (3–3.5 mm) [26] than the periodontal soft tissues (2.73 mm) [28]. The soft tissue dimensions around implants account for 2 mm epithelium + 1–1.5 mm connective tissue, and in teeth, account for 1.6 mm epithelium + 1.06 mm connective tissue [26–28].
- Fewer blood vessels are found in peri‐implant soft tissues than around teeth because the blood supply is only supraperiosteal; meanwhile, blood supply around teeth comes from the vascular plexus of the periodontal ligament and the supraperiosteal blood vessels [28]. This can result in down migration of the connective tissue [29, 30]
- Material chemistry influences the apical displacement of the epithelia. Pure titanium, alumina oxide ceramics (Al2O3), and zirconia oxide (ZrO2) abutments will develop a gingival cuff of 3.5 mm [31]. Meanwhile, gold and platinum alloys can produce apical displacement of the epithelia and potential enlarged gingival cuffs and bone loss [32].
- The presence of contaminants on the abutment surface produces an apical displacement of the epithelia and increased inflammatory reactions [33].
- Butt‐joint implant–abutment connections result in apical epithelial migration, but platform‐switched implant–abutment connections result in medial migration of the epithelia [34–36].
- Epithelial attachment on implant abutments is weaker (few hemidesmosomes) and repeated probing can increase probing depths compared to probing around natural teeth [37].
- Multiple abutment connection‐disconnection can increase the apical migration of the epithelia and bone loss [38, 39].
- The presence of horizontal microgrooves at the abutment surface can prevent the epithelial down growth by inducing transversal attachment of connective tissue fibers into the abutment [40].
Mature soft tissues around the prosthetic abutments are observed between six and eight weeks after their installation [41], and by the resolution of inflammatory cells observed at eight weeks of healing in samples obtained from the adjacent gingival tissue [42]. Maintaining a strong epithelial and connective tissue attachment to the abutment surface is necessary to resist mechanical injury and prevent the penetration of pathogens into the transmucosal area of the prosthetic abutment [43].
The following sections of this chapter will evaluate prosthetic abutment materials, surfaces, coatings, designs, and emergence profiles that can improve implant maintenance by limiting biofilm formation and establishing a robust gingival attachment.
Prosthetic Abutment Material
In general, four main materials are used for prosthetic abutments: metals and metal alloys, ceramics, hybrid abutments (composed of two or more materials), and polymer‐based abutments (Figure 7.3) [44–48].
From esthetic and mechanical aspects, each abutment material possesses specific characteristics that indicate its use. For example, ceramic or coated metallic abutments will provide higher esthetics [49], metallic and zirconia abutments will provide high mechanical strength [50], hybrid abutments permit angle corrections with esthetic advantages [51], and abutments based on polymers will allow long‐term temporization [52].
However, from the perspective of maintaining the stability of the soft tissues, the prosthetic abutment material may have other effects [53–55].
The effect of different abutment materials on the soft tissues around dental implants was evaluated by Ferrari et al. [53] in a randomized clinical trial [53]. Forty‐seven patients received ninety‐seven dental implants. The implants were restored with prosthetic abutments made in titanium (15), titanium with a titanium nitride coating (18), and zirconia (14). After two years of maintenance with a supportive periodontal care program (recalls every four or six months), the keratinized tissue, gingival biotype, peri‐implant markers, and recession were similar for the three used materials without differences between groups. Thus, suggesting that plaque control is key to maintaining peri‐implant health independent of the abutment composition [53].
Linkevicius et al. [54] evaluated the stability of the peri‐implant hard and soft tissues when abutments of different materials (titanium, gold alloy, zirconium oxide, and aluminum oxide) were used [54]. The authors completed a systematic review, including clinical studies, human histology, and animal studies. After the search and selection process, nine articles were included: three animal studies, two human histological studies, and four randomized clinical trials. The authors concluded that soft and hard tissues presented similar stability when titanium abutments were used compared to gold alloy, zirconium oxide, and aluminum oxide [54].
The abutment must also resist degradation under function in the oral environment [55]. De Avila et al. [55] reviewed different parameters of the surface and bulk materials used for implant abutments.
The authors concluded that the oxidation of the surface, contamination during handling, and the corrosion processes, can affect the integrity of metallic abutments, increasing the release of metallic ions, thus, increasing the release of inflammatory cytokines in the adjacent tissues [55].

Figure 7.3 Materials used for the fabrication of prosthetic abutments. Although titanium‐based abutments are predominant, new hybrid materials and polymers are available thanks to new fabrication methods.
Biofilm Formation on Different Abutment Materials
Biofilms around prosthetic abutments are formed in supragingival and subgingival locations, the subgingival biofilm is difficult to remove, and their characteristics are dependent on the surface energy, roughness, and partially on the chemical composition of the abutments [56].
To demonstrate the biofilm formation on modern implant abutments, Hahnel et al. [57] completed an experimental study using disks made in zirconia, polyetheretherketone (PEEK), and titanium and compared these with control disks made in polymethylmethacrylate (PMMA). The disks were highly polished and the surface roughness and energy were measured. Afterward, salivary biofilm was seeded with S. mutans, S. gordonii, A. naeslundii, and C. albicans and the adhered and dead microorganisms were measured.
Their results showed lower roughness for PEEK (Ra = 0.04 μm) and PMMA (Ra = 0.05 μm) compared to zirconia (Ra = 0.16 μm) and titanium (Ra = 0.17 μm). The surface energy (drop angle) was higher for PEEK (42.19), followed by PMMA (39.14), titanium (35.62), and zirconia (31.72). After 20 hours, the lowest cell availability was found in PEEK, followed by zirconia, titanium, and PMMA. After 44 hours, the biomass increased in all the materials. In regards to dead microorganisms, after 44 hours zirconia (74.87%) showed higher percentages of dead microorganisms, followed by titanium (68.82%), PEEK (63.72%), and PMMA (60.17%) [57].
Desch et al. [58], evaluated the biofilm formation on 192 zirconia and titanium disks (n = 96) bonded to intraoral splints in twelve patients. Samples were collected at different time points (6 hours, 24 hours, three and five days), and volume of biofilm, and number of live and dead bacteria were measured with confocal laser scanning microscope. In addition, the microbial composition was analyzed using DNA sequencing analysis [58].
The results showed that the volume of biofilm increased with the time on both materials, reaching a plateau at three days. The microbial composition differed over time but was not influenced by material composition. Some microorganisms were detected in higher percentages at the first hours (Abiotrophia defectiva, Lautropia mirabilis, Ralstonia solanaceraum, Rothia, Prevotella, and Streptococcus) but decreased at three and five days. The remaining microorganisms started with low percentages and increased over time (Actinomyces, Bergeyella, Capnocytophaga, Eikenella, Fusobacterium, Neisseria, Porphyromonas, Veillonella). The authors concluded that there are no differences between zirconia and titanium in the quantity of adherent bacterial species after three days [58].
Zeller et al. [59] studied the biofilm formation on metallic alloys, zirconia, and PEEK [59]. A removable intraoral appliance was designed to retain 15 disks distributed equally at the palatal area and inserted in sixteen patients. The disks were designed with 5 mm diameter and 1 mm thickness. They were manufactured in five materials: gold‐based andsilver‐based noble metal alloy, zirconia, PEEK, or titanium zirconium alloy. After the appliances were delivered, samples were collected after 24 and 48 hours. The results showed that the gold and silver‐based alloys presented the lowest number of colonies forming units, and titanium‐zirconium alloy, zirconia, and PEEK showed similar values. Thus, indicating that silver and gold‐based alloys can be used in the transmucosal portion of implants to limit the biofilm formation [59].
Herrman et al. [60] compared early and mature biofilm (formed after 3 days and after 31 days, respectively) growing in simulating implant surface, implant collar, and abutments [60]. Disks with 5 mm diameter and 2 mm thickness were fabricated in four materials: sandblasted and acid‐etched titanium (Ra = 1.87 μm, simulating the implant surface), machined titanium (Ra = 0.18 μm, simulating implant collar), titanium‐aluminum‐vanadium alloy (Ra = 0.16 μm), and zirconia (Ra = 0.74 μm) simulating the abutment roughness. The disks were not polished to maintain their original roughness and topography. Mandibular acrylic appliances were developed and the disks were attached facing the lingual side. The appliances were inserted in 14 patients.
The devices were removed only during eating and for routine oral hygiene. Total bacteria were detected using polymerase chain reaction. Their results showed that bacteria colonized all the materials after exposure to the oral cavity at 3 and 31 days. Curiously in the early biofilm, bacteria colonies at three days were lowest for machined titanium (3.83 × 108 ± 3.13 × 108) and highest for zirconia (5.63 × 108 ± 4.83 × 108). Mature biofilm at 31 days showed bacteria counts higher for zirconia (6.66 × 108 ± 4.19 × 108) and lower for titanium alloys (4.67 × 108 ± 2.81 × 108). The comparison of machined versus sandblasted‐acid etched titanium showed higher bacterial counts for sandblasted‐acid etched titanium compared to machined titanium. Most of the detected bacterial species increased cell numbers in mature biofilms compared to the early biofilms. The authors concluded that the higher roughness observed in zirconia abutments explains the higher bacterial cell counts compared to machined titanium and titanium alloy [60].
More recently, Del Rey et al. [61] completed a systematic review on the biofilm formation of alternative abutment materials compared to titanium. Studies evaluating biofilm formation on metallic, ceramic, or polymeric materials were included.
The following variables were analyzed: microbial counts, biofilm thickness and area, and cell viability. In total, 19 studies were included (10 clinical and 9 in situ). The alternative materials used in these studies were alumina, cobalt chromium, polytetrafluoroethylene (PTFE), gold and gold‐platinum alloys, and zirconia. The authors found that titanium versus zirconia abutments showed comparable bacteria and inflammation levels and health conditions, but bacteria levels increased over time. Gold alloys showed thick biofilms and more extensive bacteria surface coverage than titanium and zirconia, and all the surfaces accumulated supragingival biofilm [61].
Despite difficult comparisons between materials, and the short‐term of the included studies, the authors suggest studies with extended follow‐up periods.
Prosthetic Abutment Surface
The transmucosal portion of the abutment surface is confined at the basal area by the implant platform and the cortical bone, laterally by the connective tissue and the gingival epithelial tissue, and coronal by the interface abutment‐restoration and the restoration. Therefore, different modifications have been completed on the transmucosal portion of the abutments to improve the behavior of the adjacent connective and epithelial tissues. These surface modifications can be divided into machined, micro‐grooved, coated, and functionalized surfaces.
Machined Surfaces
In in vivo studies, machined surfaces have shown that the fibroblasts of the connective tissue are oriented with their long axis parallel to the abutment [62, 63], there is a lack of connective tissue attachment to the abutment surface, and the junction epithelia is long [40]. In addition, the original machined surface changes after two months of exposure to the oral environment from smooth (surface roughness Sa = 0.259 μm) toward a rougher surface (surface roughness Sa = 0.430 μm) [64].
In in vitro studies, fibroblasts were attached firmly to the surface of machined titanium disks. Their bodies were flat, rounded, with short and thick lamellipodia, without clear orientation on the surface [65]. In in vivo studies, immunofluorescent analyses of oral epithelial‐like cells showed well‐developed actin filaments and expression of adhesion proteins (laminin‐5) [66]. Finally, the soft tissues adjacent to machined surfaces showed expression of Intercellular Adhesion Molecule (ICAM‐1), Matrix Metalloproteinases (MMP’s), and MMP‐9 (which regulates neutrophils migration) [67].
The epithelium facing the machined surface was analyzed by Atsuta et al. [68]. The authors described three zones: upper, middle, and lower portion. At the lower portion, adhesion structures (hemidesmosomes) were present in a zone of 100 nm covering about 80% of the lower area. At the middle portion, adhesion structures were present in 40% of the area [68].
Micro‐Grooved Surfaces
Early studies completed by Brunette et al. (1991) demonstrated in vitro and in vivo that organized topography (repeated patterns like grooves) on the material surface can guide cell orientation and cell spreading [69].
Weiner et al. [70] evaluated the connective tissue, epithelia, and crestal bone around micro‐grooved and machined implant collars [70]. In their study, 36 implants (3.75 mm diameter and 8 mm length) were inserted in six dogs. Each dog received six implants (three implants with machined collar and three implants with micro‐grooved collar) in the bilateral healed premolar and molar areas of the mandible. Both collars had a length of 2 mm. The micro‐grooved collar was divided into three zones an upper machined zone of 0.5 mm, a middle zone of 0.7 mm was laser micro‐grooved with micro‐grooves of 8 μm wide, and the apical zone of 0.8 mm laser micro‐grooved with micro‐grooves of 12 μm wide. Samples were obtained at three and six months after implant insertion. Histologic samples were obtained and evaluated for soft tissue recession, bone growth, bone loss, and the number of osteoclasts. The authors found that the micro‐grooved surfaces presented minimal soft tissue recession, lower bone loss, and osteoclast activity compared to machined surfaces for all the evaluated periods. Collagen fibers were attached to the middle zone of the micro‐grooved surface, and some microgrooves of the apical zone of the micro‐grooved surface showed bone formation. Meanwhile, the machined collars did not show attachment of collagen fibers or bone formation [70].
Nevins et al. [40] completed an animal study in dogs to evaluate the influence of two implant surfaces with two types of healing abutments [40]. The implants were tapered, with or without a 0.5 mm machined collar, and their surfaces were treated by resorbable blast texturing. The implants were inserted at the bone level in healed mandibular ridges and received either a micro‐grooved healing abutment or a machined healing abutment. Healing abutments, soft tissues, implants, and bone were evaluated with micro‐computed tomography (micro‐CT), transmitted light, and scanning electron microscopy (SEM).
The histological analysis showed bone and connective tissue attached to the micro‐grooved zone, and the junctional epithelium ending at the highest coronal portion of the micro‐grooved zone, without the characteristics of a long junction epithelium given that the connective tissue attachment impeded the apical migration of the epithelium. The SEM analysis showed dense connective tissue attached to the micro‐grooved abutment surfaces. Meanwhile, the junctional epithelium migrated apically on machined abutment surfaces, and no connective fiber attachment was observed [40].
Shin et al. [71], evaluated micro‐grooves influence on the healing of peri‐implant tissues in immediate implants in dogs [71]. Implants with external hexagon connection, with the same geometry (cylindric‐apical taper) and dimensions but different collars. The control group possessed a machined collar, and the test group had a micro‐grooved collar. Four dogs received sixteen implants (eight implants of each group) inserted immediately after extracting the third and fourth mandibular premolars at a bone level or slightly apical to the lingual bone crest. After three months, the samples were processed for histological analysis. The results showed that the first bone‐to‐implant contact was higher in micro‐grooved implant collars than in machined collars and demonstrated that the connective tissue fibers of the peri‐implant mucosa were attached perpendicular to the collar. Meanwhile, the first bone‐to‐implant contact was more apical in machined collars, the connective tissue fibers were parallel, and the mucosa penetrated below the machined collar. Thus, the authors conclude that the micro‐grooved collars improve the attachment of soft and hard tissues and reduce the marginal bone loss in immediate implants [71].
Micro‐grooved abutments also can reach reattachment of the soft tissues to their original levels. Therefore, using micro‐grooved components like healing abutments and micro‐grooved prosthetic abutments are recommended when multiple abutment replacements are required [72]. Furthermore, enhanced cell adhesion and soft tissue sealing to the micro‐grooved surface benefit against bacterial intrusion [73].
In a literature review, Ketabi et al. (2015) concluded that micro‐grooves at the implant collar inhibit apical migration of the soft tissues, thus reducing crestal peri‐implant bone loss. Characteristics of the micro‐grooved surface, including the dimensions of the micro‐grooves, their pitch, valleys distribution, and their nano topographical features, allow basal tissue attachment and stop epithelia from migration. In addition, the connective tissue surrounding micro‐grooved collars resembles that located around natural teeth [74].
Geurs et al. [75] completed a clinical study to evaluate the healing and formation of the connective tissue attachment around micro‐grooved and machined abutments. It was observed that connective tissue attachment occurs on micro‐grooved surfaces during healing and even after replacement by new micro‐grooved abutments. However, machined abutments resulted in epithelial attachment without connective tissue presence. After substitution by micro‐grooved abutments, this situation did not change. However, if a wound was created after the removal of a machined abutment and a micro‐grooved abutment replaced this, then connective tissue attachment was observed. The authors recommended removing the epithelium to allow connective tissue attachment when replacing machined by micro‐grooved abutments [75].
Finally, a longitudinal randomized controlled clinical trial showed that micro‐grooved abutments supporting cemented single implant restorations reduced the sulcus depth and crestal bone loss compared to machined prosthetic abutments supporting cemented single implant restorations. The authors concluded that using micro‐grooved implant collars and micro‐grooved abutments is a dual strategy that should be used to promote a more robust and healthier soft and hard tissue integration [76].
Coated Surfaces
Coatings have been applied to the surface of prosthetic abutments with two main goals: to improve soft tissue attachment and reduce bacteria colonization [77, 78]. The improvement of the soft tissue attachment to the abutment surface should be evidenced by the attachment of collagen fibers in a perpendicular direction, reduction of the apical migration of the gingival epithelium, improved tissue healing, and therefore, limited crestal bone resorption [79]. The reduction of bacterial colonization should be reflected in delayed biofilm formation, reduced plaque, reduced infection, reduced inflammation, and prevention of bone loss [80, 81].
The following antibacterial coatings have been tested on titanium abutment materials: anodic spark deposition, nanoporous titanium oxide (TiO2), titanium‐bismuth‐gallium (Ti‐Bi‐Ga), doxycycline, diamond‐like carbon (DLC), silver and silver nanoparticles, titanium nitride, zirconia nitride [82–90] (Table 7.2).
The following coatings for the improvement of the soft tissue behavior around titanium abutment materials have been evaluated: collagen, titanium nitride, zirconium nitride, and Vitamins D and E [77] (Table 7.3).
Although coatings have the potential for development, only Doxycycline, Silver nanoparticles, Titanium, and Zirconia nitrides, proved antibacterial activity against a limited number of microorganisms (Staphylococcus epidermidis, S. aureus, Streptococcus mutans, S. salivarius, S. sanguinis, S. sobrinus, and Pseudomonas aeruginosa). Furthermore, collagen was the only coating that demonstrated human fibroblast adhesion and proliferation [77, 82–90]. Finally, the effects of mechanical or chemical cleaning methods on the integrity of the coatings after long‐term exposure to use and cleaning are unknown.
Functionalized Surfaces
A biofunctionalized surface uses a coating that confers properties different from the base materials by modifying their composition, structure, and morphology without altering their mechanical properties. Such modifications can improve biocompatibility (increase cell attachment and interactions), reduce bacterial adhesion, and reduce corrosion and wear [91, 92].
The methods for surface functionalization include the addition of ceramic, polymer, and polymeric gel coatings, and ceramic coatings are preferred given their high stability and osteoconductivity [93, 94]. The stability of a biofunctionalized surface in the intraoral environment can be affected by variable conditions of temperature, pH, lack of homogeneous coating and mismatch with the surface, residual stresses, and continued degradation. These mechanisms are poorly understood and can affect their efficacy [91, 95].
The Transmucosal Area of Prosthetic Abutments
The transmucosal portion of the prosthetic abutment passes through the gingival tissues and in different emergence angles continues toward the cervical contour of the implant restoration, these angles vary from 0° to 90°, and provide physical support for the peri‐implant soft tissues [96].
For example, the profile of the prosthetic abutment, as per Steigmann et al. [97] can be concave, slightly concave, or convex. When the implants are too buccal, concave abutments are indicated. When the implants are centered in the ridges, slightly concave abutments could be used, and for too‐palatal implants, convex abutments should be preferred. Thus, convex abutments can help in displacing the buccal tissues facially; meanwhile, slightly concave abutments maintain the position of the soft tissues, and concave abutments could be used in case of limited buccal space or thick gingival tissues [97].
Chu et al. [98] described the implant restorative emergence profile (IREP) as “the contour of the implant/abutment crown complex as it arises from the restorative platform of the implant and emerges from the peri‐implant soft tissues” and also called it the “implant restorative contour” [98].
Table 7.2 Antibacterial coatings tested on titanium abutment materials. Only Doxycycline, silver nanoparticles, and titanium and zirconia nitride coatings showed antibacterial activity.
Antibacterial coatings for titanium surfaces | |||
---|---|---|---|
Technique characteristics | Antibacterial efficacy | Bacteria tested | |
Anodic spark deposition | Incorporation of calcium and phosphate ions within the titanium oxide layer. Thicker titanium oxide layers. | Negligible Bacteria was present in treated and non‐treated surfaces. | Streptococcus. Mutans, Salivaris and Sanguis |
Nanoporous Titanium Oxide | Used sol‐gel treatment to produce a nanoporous titanium oxide coating. | Negligible. No differences in biofilm volume between test and controls. | Streptococcus. Sanguis and Actinomyces Naeslundii |
Titanium‐Bismut‐Gallium (Ti‐Bi‐Ga) | Used vapor deposition to incorporate Ti, Bi, and Ga within the titanium oxide layer. | Negligible. Biofilm formation was not impeded by the coatings. | Multiple bacteria |
Doxycycline | Cathodic polarization was used to incorporate doxycycline on the surface. | Antibacterial activity achieved for 24 hours and one week. | Staphylococcus epidermidis |
Diamond‐Like‐Carbon | Plasma‐enhanced vapor deposition to coat the surface with DLC films. | Negligible. Bacterial adhesion was not inhibited. Bacterial leakage persisted. No antibacterial properties. | Enterococcus faecalis, Escherichia coli. |
Silver nanoparticles | Plasma sputtering method to coat the titanium disks with silver. | Antibacterial activity against Streptococcus mutans, and Pseudomona aeuroginosa. | Staphylococcus aureus. Streptococcus mutans, Pseudomonas aeuroginosa |
Titanium nitride | Anodization | Antibacterial activity demonstrated by higher counts of dead bacteria. | Streptococcus salivarius, S. sanguinis, S. mutans, S. sobrinus, S. oralis |
Zirconia nitride | Anodization | Antibacterial activity demonstrated by higher counts of dead bacteria. | Streptococcus salivarius, S. sanguinis, S. mutans, S sobrinus, S. oralis |
Table 7.3 Coatings for the enhancement of the soft tissues for titanium surfaces. Only collagen coatings produced increased cell adhesion, cell viability, and proliferation.
Coatings for enhancement of the soft tissues in contact with titanium surfaces | |||
---|---|---|---|
Technique characteristics | Type of cells or tissue | Improvement observed | |
Collagen | Dip coating and anodic immobilization to incorporate a collagen coating. | Human fibroblasts | Increased cell adhesion at two and four hours |
Increased cell viability and proliferation at one, three, and seven days | |||
Itanium nitride | Anodization | Human fibroblasts from biopsies | No differences in cell adhesion or proliferation compared to non‐coated surfaces |
Zirconium nitride | Anodization | Human fibroblasts from biopsies | No differences in cell adhesion or proliferation compared to non coated surfaces |
Vitamin D | Ultraviolet irradiation | Human fibroblasts from biopsies | No differences in cytotoxicity, cell morphology, expression of adhesion markers, or wound healing |
Vitamin E | Ultraviolet irradiation | Human fibroblasts from biopsies | No differences in cytotoxicity, cell morphology, expression of adhesion markers or wound healing |
In single implants, the IREP should imitate the emergence profile of the extracted tooth on all sides (buccal, mesial, distal, and lingual); however, this is not always possible without complementary procedures like bone and soft tissue grafts and temporization [98]. Two areas of the soft tissues that surround the whole circumference of the transmucosal portion of the prosthetic abutments were described: the critical and the subcritical contours [99]. The critical contour is an area of around 1 mm (coronal‐apical length) immediately apical to the gingival margin. It can be facing the prosthetic abutment, the implant crown, or both, depending on the position of the interface abutment/crown. On the buccal side, the critical contour determines the level of the gingival margin; meanwhile, in the interproximal areas, it determines the shape of the implant crown (square or triangular) [99]. The subcritical contour is the area below the critical contour that extends to the implant platform. Its dimensions are variable (short in shallow implant placement and long in deep implants) and help in establishing the IREP [99].
Correlations between the peri‐implant soft and hard tissues/implant prosthetic abutment/implant restorations are complicated given variable patient and implant factors (gingival biotype, location, healed versus post‐extraction implants, microbiome, implant insertion depth, type of implant connection, abutment contour characteristics, interface abutment‐crown, materials, and others) [100].
To overcome this issue, Mattheos et al. [101] coined the term “implant supracrestal complex” (ISC) to describe and correlate the gingival tissue, mechanical components, and bacteria in the transmucosal portion of the implant prosthesis [101]. In addition, in a recent systematic review of clinical studies analyzing the elements of the design of the ISC, Mattheos et al. [102], concluded that bone‐level implants with abutments with wide emergence angles and convex abutment profiles increase the risk for peri‐implantitis. Also, prosthesis with accessible and cleansable designs reduces peri‐implant mucositis. If mucositis exists, its treatment is more difficult with deep peri‐implant sulcus [102].
Gomez‐Meda et al. [103] described a concept for the vertical distribution of the subgingival design of emergence profiles around implant restorations. In their approach, the subgingival emergence profile contains three zones: the esthetic zone (E), the bounded zone (B), and the crestal zone (C).
The esthetic zone (E) influences the position of the free gingival margin, is around 1 mm thick, and its contour should be convex and establish the morphology of the clinical portion of the implant crown. In addition, it contains the free gingiva.
The bounded zone (B) possesses between 1 and 2 mm thickness. It is located below the E zone. It is determined by the thickness of the surrounding soft tissues, the implant position, and the implant neck design and should be preferably concave. It contains the gingival epithelial tissue.
The crestal zone (C) is 1–1.5 mm in thickness and is located below the B zone. The abutment contour should be straight or concave in this area to avoid pressuring the adjacent hard tissues. Its dimensions also depend on the implant insertion depth. The supracrestal connective tissue is present in this area [103].
Transmucosal Designs of Prosthetic Abutments
Different transmucosal designs have been used in prosthetic abutments, including parallel, concave, convex, convergent, divergent, and customized configurations [97103–107].
A parallel abutment typically possesses walls without angular, geometrical, or diameter changes. Concave abutments possess walls that converge toward a narrower central portion and then diverge. In convex abutments, the walls diverge from the center to an area of maximum curvature, then converge to the coronal. In a convergent abutment, the walls progressively converge toward the coronal. A divergent abutment possesses walls that gradually diverge from the center. Finally, a custom abutment imitates the contour of the contralateral tooth or adopts multiple unique geometries required for the restoration design [103–107].
Valente et al. completed a systematic review to evaluate the effect of different abutment geometries (convergent or concave versus divergent and parallel) on marginal bone loss and esthetics [104]. Five studies fulfilled the inclusion criteria. Their results showed that concave and convergent abutments were beneficial in maintaining soft tissue levels and reducing bone loss [104].
Siegenthaler et al. [108] completed a one‐year clinical trial on anterior implant restorations over convex or concave abutments to evaluate the stability of the gingival margins. Their results showed that concave abutments presented a frequency of buccal gingival recessions of 14.3%; meanwhile, abutments with convex profiles presented a frequency of buccal gingival recessions of 64.3% [108], The authors concluded that after a year, concave abutments stabilized the gingival margin better than convex abutments.
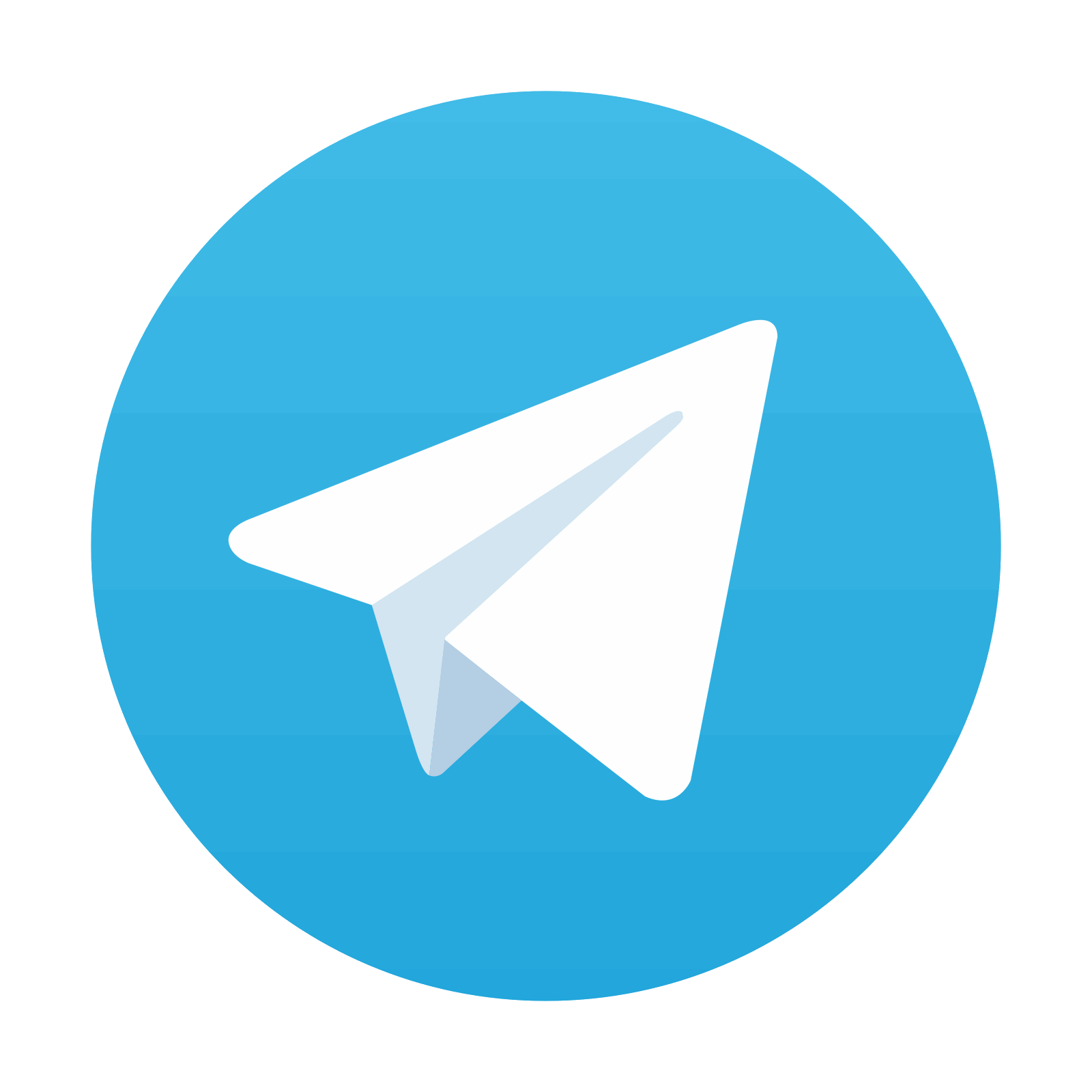
Stay updated, free dental videos. Join our Telegram channel

VIDEdental - Online dental courses
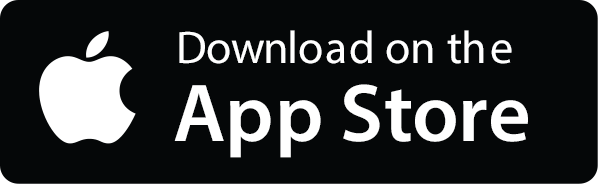
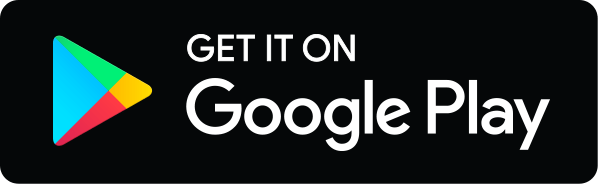