Abstract
Objective
To study the effect of silver diamine fluoride (SDF) treatment and incorporating casein phosphopeptide-amorphous calcium phosphate (CPP-ACP) into a glass-ionomer cement (GIC) to prevent secondary caries.
Method
A cervical cavity was prepared on 32 premolars for the following restoration groups: group 1, conventional GIC restoration; group 2, SDF (38%) treatment and conventional GIC restoration; group 3, CPP-ACP (3%) modified GIC; and group 4, SDF treatment and CPP-ACP modified GIC. The restored teeth were thermal-cycled before undergoing a multi-species cariogenic biofilm challenge. The restored teeth were examined by micro-computed tomography (micro-CT), scanning electron microscopy with energy dispersive X-ray spectroscopy (SEM/EDX) and Fourier transform infrared (FTIR) spectroscopy. Data were analyzed by two-way ANOVA.
Results
Micro-CT determined outer lesion depths for groups 1–4 were: 123 ± 6 μm, 87 ± 7 μm, 79 ± 3 μm and 68 ± 5 μm respectively. An interaction effect on the outer lesion depth was found between the restorative materials and SDF treatment ( p < 0.001). Both SDF treatment and modification with CPP-ACP had a significant effect on outer lesion depth ( p < 0.001). SEM/EDX showed an increase of calcium and phosphorus at the root dentine adjacent to the restoration in groups 3 and 4 (CPP-ACP modified GIC). FTIR revealed that SDF treatment and CPP-ACP modified GIC had a significant effect on amide I-to-hydrogen phosphate ratio on the material-root interface ( p = 0.001).
Conclusion
SDF treatment and incorporation of CPP-ACP into GIC restorative material can prevent secondary root caries development.
Clinical significance
The results provide useful information to dentists in formulating clinical management protocols and material when treating root caries.
1
Introduction
Secondary (recurrent) caries has been considered to be the most common factor for failure of direct restorations . A study reported that more than 25% of replacements of silver amalgam and resin composite restorations were attributed to secondary caries . The use of alternative restorative materials possessing potential anti-cariogenic capabilities such as glass-ionomer cements (GICs) has often been suggested . GIC has the ability to enhance remineralisation through its release of fluoride ions; and inhibit secondary caries in vivo and in vitro . However, some researchers found that fluoride leached from GIC was limited and insufficient to prevent the development of secondary caries . Therefore, new remineralising agents have been introduced to supplement and enhance fluoride to restore tooth minerals when demineralization has occurred .
Casein phosphopeptide-amorphous calcium phosphate (CPP-ACP) is a bioactive agent derived from bovine milk protein, casein, calcium and phosphate . Casein is the principal phosphoprotein in bovine milk, predominantly as calcium phosphate stabilised micellular complexes . Studies have demonstrated CPP-ACP can prevent enamel demineralisation and promote enamel subsurface lesion remineralisation in animal and human in situ studies . CPP-ACP has been regarded as a calcium phosphate reservoir, buffering the activities of free calcium and phosphate ions . The anticariogenic activity of CPP-ACP has been ascribed to its ability to concentrate amorphous calcium phosphate at the tooth surface and within the biofilm, thus consequently helping to maintain a state of supersaturation with regard to tooth mineral . In addition, it was demonstrated that CPP-ACP was stable in the presence of fluoride and interacted with fluoride ions to yield amorphous calcium fluoride phosphate (ACFP) . The ACFP complex, which was stabilised by CPP at the tooth surface, can provide the necessary elements to promote remineralisation via fluorapatite formation . This makes CPP-ACP a promising addition to restorative materials and dental products. CPP-ACP could be incorporated into GIC, and a study showed it became more resistant to acid challenge . It also has been suggested that CPP-ACP modified GIC is able to enhance calcium and phosphate ion release , while there were no significant adverse effects on the mechanical properties of the restorative material .
The cariogenic biofilm of secondary caries is similar or identical to that of primary caries, and comprised mainly of Streptococci , Lactobacilli and Actinomyces naselundii . Silver diamine fluoride (SDF) can inhibit the formation of multi-species cariogenic biofilms on dentine surfaces . SDF is an inexpensive topical fluoride medicament commonly used for preventing and arresting caries, and has been approved for clinical use by the United States Food and Drug Administration . Clinical trials showed that SDF was effective in preventing and arresting caries . Laboratory studies have also demonstrated that SDF has a strong antibacterial effect on cariogenic biofilms and hampered caries progression . Another study concluded that SDF did not adversely affect the bond strength of restorations to dentine using resin-based adhesives . SDF can be used to treat carious lesions without excavation of the superficial infected layers and thus it minimizes the risk of pulp exposure . A laboratory study reported that prior treatment with SDF can increase resistance of GIC and composite restorations to secondary caries . Another laboratory study found that CPP-ACP modified GIC restoration had an improved anticariogenic potential compared to a control GIC . However, it is unknown whether the combination of SDF treatment in conjunction with the placement of a CPP-ACP modified GIC restorative material can enhance the preventive effects on secondary caries development. Therefore, the aim of this study was to investigate the effects of SDF treatment on the prevention of secondary root dentine caries development around conventional GIC and CPP-ACP modified GIC restorations. The null hypotheses tested were that: (1) SDF treatment before GIC restoration has no effect on secondary caries prevention; (2) incorporating CPP-ACP into GIC has no effect in prevention of secondary caries; and (3) there is no interaction effect on secondary caries prevention between SDF treatment and incorporating CPP-ACP into GIC restorative material.
2
Methods
2.1
Specimen preparation
This study was approved by the local Institutional Review Board: IRB number UW 12-221. Extracted mature (closed apex) human premolars, without visible defects, were collected with the patients’ consent. The teeth were stored in a 0.1% thymol solution. After thorough cleaning, a box-shaped cavity (4 × 2 × 2 mm) located across the cemento-enamel junction was prepared on each premolar. The cavities were prepared by means of a high-speed handpiece with a tungsten carbide bur (FG 330; SS White, Lakewood, NJ, USA) under copious water-cooling. The teeth were then sterilised by autoclaving . After conditioning with 10% polyacrylic acid , the teeth were randomly allocated to the following treatment groups: Group 1 (conventional GIC), the cavities were filled with a conventional GIC, Group 2 (conventional GIC + SDF), the cavities were treated with SDF for 3 min, and then were filled with conventional GIC, Group 3 (CPP-ACP modified GIC): the cavities were filled with GIC containing 3% w/w CPP-ACP, and Group 4 (CPP-ACP modified GIC + SDF), the cavities were treated with SDF for 3 min, and then were filled with GIC containing 3% w/w CPP-ACP. The operator wore sterile gloves and used sterile hand instruments. All restored teeth were then stored at 37 °C and 100% humidity for 24 h. The conventional GIC used in this study was a conventional self-curing glass-ionomer cement (Fuji VII, GC Corp, Tokyo, Japan). The CPP-ACP modified GIC used was Fuji VII EP (GC Corp, Tokyo, Japan). The 38% SDF solution was from the silver capsule of Riva Star (SDI, Bayswater, Australia). It was topically applied for 3 min to the cavities using a micro-brush provided by the manufacturer. Before restoration, the cavities were blown dry gently by a 3-in-1 syringe.
Results of a previous study suggested an outer lesion depth of approximately 120 μm in the dentine at the material-root junction would develop after cariogenic challenge . The common standard deviation (sigma) was set at 20 μm, the type I error (α) was 0.05 and the power was 0.90. It required at least 6 samples to detect a difference of 40 μm between groups. This study used 32 teeth with 8 samples in each group. The flowchart of this study was shown in Fig. 1 .

2.2
Thermocycling
The restoration surfaces were polished with sterile sand-paper discs to ensure there was no excess material over the cavity margins. All teeth were coated with an acid-resistant nail varnish (Clarins, Paris, France), leaving approximately a 1-mm window around the cavity margins. To mimic the aging process, all groups were thermocycled for 1500 cycles between 55 ± 5 °C and 10 ± 5 °C deionized water baths with a 32-s dwell time in each bath and a 14-s interval between baths . The teeth were then immersed in 70% ethanol for 1 min, followed by drying in air for 20 s before cariogenic biofilm challenge .
2.3
Cariogenic biofilm challenge
Four common species of cariogenic bacteria- Streptococcus mutans ATCC (American Type Culture Collection) 35668, Streptococcus sobrinus ATCC 33478 , Lactobacillus rhamnosus ATCC 10863 and Actinomyces naselundii ATCC 12014 were used in this study . The bacteria were cultured in blood agar plates at 37 °C anaerobically for 2 days. Then, isolated colonies were transferred to tubes containing brain-heart infusion (BHI) broth with 5% sucrose and incubated for another 24 h (37 °C, anaerobically). Subsequently, the bacterial cell pellets were harvested and re-suspended in BHI broth with 5% sucrose to a cell density of McFarland 2 (6 × 10 8 cells/mL) . A 500 μL aliquot of each bacteria culture was mixed and inoculated on each tooth sitting in a well of a 12-well plate. The teeth were stored in an anaerobic chamber at 37 °C for 7 days. The medium was refreshed daily and contaminants were checked by performing Gram stain tests of the used media.
2.4
Assessment of outer lesion depth
The extent of demineralisation of dentine at the material-root junction which represents secondary caries was assessed by measuring outer lesion depth [3]. To assess the outer lesion depth, the teeth (n = 8 per group) were scanned non-destructively under water using a SkyScan 1076 micro-CT (SkyScan, Antwerp, Belgium). A 1 mm thick aluminium filter was used to remove low-energy radiation. Scanning was performed with a spatial resolution of 8 μm at a voltage of 80 kV and a current of 100 μA. NRecon reconstruction software (SkyScan, Antwerp, Belgium) was used to reconstruct the scan results of each tooth. After reconstruction, images of teeth were viewed by the software CTAn (SkyScan, Antwerp, Belgium). Cross-sectional images displaying lesion area of each tooth were identified from the reconstructed 3-D images. Ten images were selected by random sampling from those lesion images and the outer lesion depth was measured using image analysis software (Image J; National Institutes of Health, USA).
2.5
Elemental analysis
Each sample was sectioned along the long axis of the tooth, midway across the restoration using a water-cooled copper disc . One half of the sample was used for elemental analysis with scanning electron microscopy with energy-dispersive X-ray spectroscopy (SEM/EDX: Hitachi S-4800 FEG Scanning Electron Microscope, Hitachi Ltd., Tokyo, Japan), and the other half was used for Fourier transform infrared (FTIR) spectroscopy (UMA 500, Bio-Rad Laboratories, Hercules, CA, USA). The objective of SEM/EDX analysis was to evaluate changes in mineral content along the material-root junction. The cross-section surfaces of the restored teeth were treated with 1% acetic acid for 5 s and washed ultrasonically using deionized water to remove the smear layer . The prepared teeth were examined under a scanning electron microscope under operating conditions of 5 kV. Lines with 500 μm length and 50 μm away from the root surfaces with analysis commencing at the restoration-root junction. The lines were analyzed by means of line-scan in terms of phosphorus, calcium, fluoride and silver ion levels.
2.6
Structural evaluation of dentine
The potential changes in the chemical structure of dentine on the restored root surface adjacent to the restoration were analyzed by FTIR spectroscopy with the infrared radiation ranging from 650 to 4000 cm −1 in wavelength number . Spectra of the demineralised dentine along the material-root junction (n = 8 per group) were obtained by the average acquisition of data at the spatial resolution achieved with a 50 × 50 μm aperture. The organic matrix of dentine is mainly composed of type I collagen, and the mineral fraction is composed of hydroxyapatite. The amide I band in the FTIR spectrum is representative of the secondary structure of collagen, and the HPO 4 2− band is representative of mineral . The ratio (amide I: HPO 4 2− ) of the areas of absorbance of collagen amide I peak between 1585 and 1720 cm −1 to the phosphate HPO 4 2− peak between 900 and 1200 cm −1 was an indicator of the extent of demineralisation of dentine due to the activity of cariogenic biofilm .
2.7
Statistical analysis
The experiment was a 2 × 2factorial (GIC with or without CPP-ACP and presence or absence of SDF treatment) combination design. Therefore, data from the outer lesion depth and amide I: HPO 4 2− were subjected to two-way ANOVA test. All of the analyses were conducted using IBM SPSS Version 20.0 software (IBM Corporation, Armonk, New York, USA), and the level of significance was set at 5%.
2
Methods
2.1
Specimen preparation
This study was approved by the local Institutional Review Board: IRB number UW 12-221. Extracted mature (closed apex) human premolars, without visible defects, were collected with the patients’ consent. The teeth were stored in a 0.1% thymol solution. After thorough cleaning, a box-shaped cavity (4 × 2 × 2 mm) located across the cemento-enamel junction was prepared on each premolar. The cavities were prepared by means of a high-speed handpiece with a tungsten carbide bur (FG 330; SS White, Lakewood, NJ, USA) under copious water-cooling. The teeth were then sterilised by autoclaving . After conditioning with 10% polyacrylic acid , the teeth were randomly allocated to the following treatment groups: Group 1 (conventional GIC), the cavities were filled with a conventional GIC, Group 2 (conventional GIC + SDF), the cavities were treated with SDF for 3 min, and then were filled with conventional GIC, Group 3 (CPP-ACP modified GIC): the cavities were filled with GIC containing 3% w/w CPP-ACP, and Group 4 (CPP-ACP modified GIC + SDF), the cavities were treated with SDF for 3 min, and then were filled with GIC containing 3% w/w CPP-ACP. The operator wore sterile gloves and used sterile hand instruments. All restored teeth were then stored at 37 °C and 100% humidity for 24 h. The conventional GIC used in this study was a conventional self-curing glass-ionomer cement (Fuji VII, GC Corp, Tokyo, Japan). The CPP-ACP modified GIC used was Fuji VII EP (GC Corp, Tokyo, Japan). The 38% SDF solution was from the silver capsule of Riva Star (SDI, Bayswater, Australia). It was topically applied for 3 min to the cavities using a micro-brush provided by the manufacturer. Before restoration, the cavities were blown dry gently by a 3-in-1 syringe.
Results of a previous study suggested an outer lesion depth of approximately 120 μm in the dentine at the material-root junction would develop after cariogenic challenge . The common standard deviation (sigma) was set at 20 μm, the type I error (α) was 0.05 and the power was 0.90. It required at least 6 samples to detect a difference of 40 μm between groups. This study used 32 teeth with 8 samples in each group. The flowchart of this study was shown in Fig. 1 .
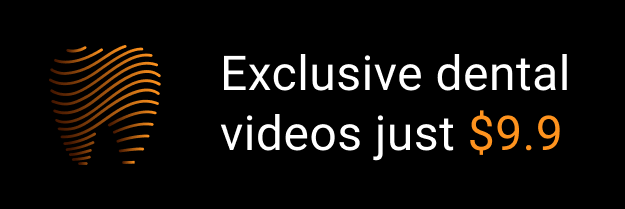