Abstract
Objective
The objective of this study was to synthesize and characterize novel star-shaped poly(acrylic acid-co-itaconic acid)s via chain-transfer radical polymerization technique, use these polyacids to formulate the resin-modified glass–ionomer cements, and evaluate the mechanical strengths of the formed cements
Materials and methods
The star-shaped poly(acrylic acid-co-itaconic acid)s were synthesized via a chain-transfer radical polymerization reaction using a newly synthesized star-shaped chain-transfer agent. The effects of MW, GM-tethering ratio, P/L ratio and aging on the compressive properties of the formed experimental cements were studied. Compressive, diametral tensile as well as flexural strengths were evaluated and compared to those of Fuji II and Fuji II LC cements.
Results
The star-shaped polyacids showed significantly lower viscosities in water as compared to their linear counterparts. The cements formulated with these novel polyacids showed significantly improved mechanical strengths i.e., 49% in yield strength, 41% in modulus, 25% in CS, 20% in DTS and 36% in FS, higher than commercial Fuji II LC. After aging in water for 30 days, the compressive strengths of the experimental cements were significantly changed with an increase of 29% in YS, 19% in modulus as well as 23% in CS and a decrease of 5% in toughness, indicating that aging in water enhances the salt-bridge formation and increases brittleness.
Conclusions
A novel light-cured glass–ionomer cement system composed of the star-shaped poly(carboxylic acid)s has been developed via a cost-effective and time-efficient chain-transfer radical polymerization.
1
Introduction
Contemporary dental filling restoratives are composed of resin composites and glass–ionomer cements (GICs) . Currently GICs are getting more and more attentions due to their unique properties including direct adhesion to tooth structure and base metals , anticariogenic properties due to release of fluoride , thermal compatibility with tooth enamel and dentin due to low coefficients of thermal expansion similar to that of tooth structure , minimized microleakage at the tooth–enamel interface due to low shrinkage , biological compatibility , and low cytotoxicity .
Despite numerous advantages, brittleness, low tensile and flexural strengths have limited the current GICs for use only at certain low stress-bearing sites such as Class III and Class V cavities . Much effort has been made to improve the mechanical strengths of GICs and the focus has been mainly on improvement of polymer backbone or matrix . Briefly two main strategies have been applied. One is to incorporate hydrophobic pendent (meth)acrylate moieties onto the polyacid backbone rendering it to become light- or redox-initiated resin-modified GIC (RMGIC) and the other is to directly increase molecular weight (MW) of the polyacid . As a result, the former has shown significantly improved tensile and flexural strengths as well as handling properties . The strategy of increasing MW of the polyacid by either introducing amino acid derivatives or N-vinylpyrrolidone has also shown enhanced mechanical strengths ; however, the working properties were somehow worsen because strong chain entanglements formed in these high MW linear polyacids resulted in an increased solution viscosity . It is known that viscosity is inversely proportional to MW of a polymer and a polymer with high MW often shows both high mechanical strengths and viscosity . So far, all the polyacids used in commercial GIC formulations have been linear polymers and applying high MW linear polyacids has been limited due to the viscosity issue.
Polymers with star, hyperbranched or dendritic shapes often demonstrate low solution or melt viscosity because these molecular structures behave similar to a solution of hard spheres and exhibit limited chain entanglements, which is beneficial to polymer processing . Since 2005, our laboratory has been developing a light-curable glass–ionomer system composed of either star-shaped or hyperbranched polymers . The polymers were synthesized via an advanced polymerization technique—atom-transfer radical polymerization (ATRP). The formed cement systems have demonstrated substantially higher mechanical strengths as compared to Fuji II LC . The main purpose of using star-shaped and/or hyperbranched polymers was to improve the mechanical strengths of the current GICs by altering the molecular architectures of the polymers without significantly increasing the viscosity of the formed polymer solution. The strategy has been found valid . However, we also realized that the polymer synthesis via ATRP was not as cost-effective and time-efficient as that via conventional free-radical polymerization, although the former is more practical than either anionic or cationic polymerization. In this paper we proposed to synthesize a star-shaped polymer via a cost-effective and time-efficient chain-transfer radical polymerization.
The objective of this study was to synthesize and characterize novel star-shaped poly(acrylic acid-co-itaconic acid)s via chain-transfer radical polymerization technique, use these polyacids to formulate the resin-modified GICs, and evaluate the mechanical strengths of the formed cements
2
Materials and methods
2.1
Materials
Acrylic acid (AA), itaconic acid (IA), pentaerythritol, mercaptoacetic acid (MEA), 2-mercaptoethanol, p-toluenesulfonic acid, 2,2′-azobisisobutyronitrile (AIBN), dl-camphoroquinone (CQ), 2-(dimethylamino)ethyl methacrylate (DMAEMA), pyridine, glycidyl methacrylate (GM), hydrochloric acid (HCl, 37%), toluene, diethyl ether, N , N -dimethylformamide (DMF), ethyl acetate, sodium bicarbonate (NaHCO 3 ), and anhydrous magnesium sulfate (MgSO 4 ) were used as received from VWR International Inc (Bristol, CT) without further purifications. Commercial glass–ionomer cements Fuji II, Fuji II LC and their corresponding glass powders were used as received from GC America Inc (Alsip, IL).
2.2
Synthesis and characterization
2.2.1
Synthesis of a star-shaped chain transfer agent
A star-shaped chain-transfer agent (CTA) was prepared as described elsewhere . Briefly, to a flask containing pentaerythritol (0.0815 mol), p-toluenesulfonic acid (7.88 mmol) and toluene (50 ml), MEA (0.4885 mol) was added. After refluxing in toluene for 3.5 h, the mixture was poured into saturated NaHCO 3 solution followed by extracting with ethyl acetate. The extract was then washed with 10% HCl, saturated NaCl solution and dried with anhydrous MgSO 4 . The final product (yield > 95%) was obtained by completely removing ethyl acetate. The synthesis scheme is shown in Fig. 1 .

2.2.2
Synthesis of the star-shaped polymer
The star-shaped poly(AA-co-IA) copolymer was synthesized via free-radical polymerization. Briefly, to a flask containing AA (0.072 mol), IA (0.018 mol), 4-arm CTA (0.503 mmol) and distilled water (75 ml), AIBN (0.09 mmol) was added. After degassing and nitrogen-purging, the solution was heated to 70 °C and kept at that temperature for 17 h. The molar feed ratio (AA:IA = 8:2 by mole) was used as suggested elsewhere . The polymers with different molecular weights (MW) were prepared by changing the amount of CTA used. The final products were freeze-dried and stored prior to use. The yields were greater than 96% for all the polymers synthesized in the study. The liner poly(AA-co-IA) copolymers were synthesized likewise except that 2-mercaptoethanol was used as CTA instead. The scheme for the 4-arm star-shaped poly(AA-co-IA) copolymer synthesis is also shown in Fig. 1 .
2.2.3
Synthesis of the star-shaped polymer with pendent methacrylate groups
The star-shaped poly(AA-co-IA) with pendent methacrylate groups was synthesized similarly as described elsewhere . Briefly, the 4-arm star-shaped polymer was tethered with GM in DMF at 50 °C overnight in the presence of pyridine (1% by weight). The GM-tethered polymer was then recovered by precipitation from diethyl ether, followed by drying in vacuo at room temperature. The synthesis scheme is also shown in Fig. 1 .
2.2.4
Characterization
The synthesized CTA and polymers were characterized by Fourier transform-infrared (FT-IR) and nuclear magnetic resonance (NMR) spectroscopy. The polymers were also characterized by gel permeation chromatography (GPC). FT-IR spectra were obtained on a FT-IR spectrometer (Mattson Research Series FT/IR 1000, Madison, WI). The proton NMR ( 1 H NMR) spectra were obtained on a 500 MHz Bruker NMR spectrometer (Bruker Avance II, Bruker BioSpin Corporation, Billerica, MA) using deuterated dimethyl sulfoxide as solvent. For determination of molecular weight, the polymers were treated with diazomethane, which was generated from diazald reacted with potassium hydroxide (KOH) in water/ethanol solution at 65 °C, to obtain partially esterified products , having solubility in THF for molecular weight estimation. Molecular weights were estimated on a Waters GPC unit (Model 410 differential refractometer, Waters Inc., Milford, MA) with THF as a solvent, using standard GPC techniques and polystyrene standards.
2.3
Evaluation
2.3.1
Viscosity determination
The viscosity of the liquid formulated with the polymer and distilled water was determined at 23 °C using a cone/plate viscometer (RVDV-II + CP, Brookfield Eng. Lab. Inc., Middleboro, MA).
2.3.2
Formulation and preparation of specimens for strength tests
A two-component system (liquid and powder) was used to formulate both conventional GIC (CGIC) and light-cured GIC (LCGIC) . For CGIC, the liquid was prepared by simply dissolving the polymer in distilled water where polymer/water (P/W) ratio = 50/50 (by weight) and the powder was Fuji II glass. The mixing ratio of glass powder verse polymer liquid or powder/liquid (P/L) ratio = 2.7/1 (by weight). For LCGIC, the liquid was prepared by dissolving the light-curable polymer, CQ (photo-initiator) and DMAEMA (activator) in distilled water where CQ = 0.9% (by weight), DMAEMA = 1.8% and P/W ratio = 65:35. The powder was Fuji II LC glass where P/L ratio = 2.7/1. Two-component GICs Fuji II where P/L ratio = 2.7/1 and Fuji II LC where P/L ratio = 3.2/1 were applied and prepared per manufacturers’ instructions.
Specimens were fabricated at room temperature according to the published protocol . Briefly, the cylindrical specimens were prepared in glass tubing with dimensions of 4 mm in diameter × 8 mm in length for compressive strength (CS) and 4 mm in diameter × 2 mm in thickness for diametral tensile strength (DTS) tests. The rectangular specimens were prepared in a split Teflon mold with dimensions of 2 mm in width (or breadth) × 2 mm in thickness (or depth) × 25 mm in length for flexural strength (FS) test. For CGIC, the specimens were removed from the mold after conditioning in 100% humidity for 15 min and then conditioned in distilled water at 37 °C for 24 h prior to testing. For LCGIC, the specimens were exposed to blue light (EXAKT 520 Blue Light Polymerization Unit, EXAKT Technologies, Inc., Oklahoma City, OK) for 2 min, conditioned in 100% humidity for 15 min, and removed from the mold, followed by conditioning in distilled water at 37 °C for 24 h prior to testing, unless specified.
2.3.3
Strength measurements
CS, DTS and FS tests were performed on a screw-driven mechanical tester (QTest QT/10, MTS Systems Corp., Eden Prairie, MN), with a crosshead speed of 1 mm/min, where the FS test was conducted in three-point bending with a span of 20 mm between supports. Six to eight specimens were tested to obtain a mean value for each material or formulation in each test. CS was calculated using an equation of CS = P / πr 2 , where P is the load at fracture and r is the radius of the cylinder. DTS was determined using an equation of DTS = 2 P / πdt , where P is the load at fracture, d the diameter of the cylinder, and t is the thickness of the cylinder. FS was obtained using an equation of FS = 3 Pl /2 bd 2 , where P is the load at fracture, l the distance between the two supports, b the breadth of the specimen, and d is the depth of the specimen.
2.3.4
Statistical analysis
One-way analysis of variance (ANOVA) with the post hoc Tukey-Kramer multiple range test was used to determine significant differences of strengths among the materials in each group. A level of α = 0.05 was used for statistical significance.
2
Materials and methods
2.1
Materials
Acrylic acid (AA), itaconic acid (IA), pentaerythritol, mercaptoacetic acid (MEA), 2-mercaptoethanol, p-toluenesulfonic acid, 2,2′-azobisisobutyronitrile (AIBN), dl-camphoroquinone (CQ), 2-(dimethylamino)ethyl methacrylate (DMAEMA), pyridine, glycidyl methacrylate (GM), hydrochloric acid (HCl, 37%), toluene, diethyl ether, N , N -dimethylformamide (DMF), ethyl acetate, sodium bicarbonate (NaHCO 3 ), and anhydrous magnesium sulfate (MgSO 4 ) were used as received from VWR International Inc (Bristol, CT) without further purifications. Commercial glass–ionomer cements Fuji II, Fuji II LC and their corresponding glass powders were used as received from GC America Inc (Alsip, IL).
2.2
Synthesis and characterization
2.2.1
Synthesis of a star-shaped chain transfer agent
A star-shaped chain-transfer agent (CTA) was prepared as described elsewhere . Briefly, to a flask containing pentaerythritol (0.0815 mol), p-toluenesulfonic acid (7.88 mmol) and toluene (50 ml), MEA (0.4885 mol) was added. After refluxing in toluene for 3.5 h, the mixture was poured into saturated NaHCO 3 solution followed by extracting with ethyl acetate. The extract was then washed with 10% HCl, saturated NaCl solution and dried with anhydrous MgSO 4 . The final product (yield > 95%) was obtained by completely removing ethyl acetate. The synthesis scheme is shown in Fig. 1 .
2.2.2
Synthesis of the star-shaped polymer
The star-shaped poly(AA-co-IA) copolymer was synthesized via free-radical polymerization. Briefly, to a flask containing AA (0.072 mol), IA (0.018 mol), 4-arm CTA (0.503 mmol) and distilled water (75 ml), AIBN (0.09 mmol) was added. After degassing and nitrogen-purging, the solution was heated to 70 °C and kept at that temperature for 17 h. The molar feed ratio (AA:IA = 8:2 by mole) was used as suggested elsewhere . The polymers with different molecular weights (MW) were prepared by changing the amount of CTA used. The final products were freeze-dried and stored prior to use. The yields were greater than 96% for all the polymers synthesized in the study. The liner poly(AA-co-IA) copolymers were synthesized likewise except that 2-mercaptoethanol was used as CTA instead. The scheme for the 4-arm star-shaped poly(AA-co-IA) copolymer synthesis is also shown in Fig. 1 .
2.2.3
Synthesis of the star-shaped polymer with pendent methacrylate groups
The star-shaped poly(AA-co-IA) with pendent methacrylate groups was synthesized similarly as described elsewhere . Briefly, the 4-arm star-shaped polymer was tethered with GM in DMF at 50 °C overnight in the presence of pyridine (1% by weight). The GM-tethered polymer was then recovered by precipitation from diethyl ether, followed by drying in vacuo at room temperature. The synthesis scheme is also shown in Fig. 1 .
2.2.4
Characterization
The synthesized CTA and polymers were characterized by Fourier transform-infrared (FT-IR) and nuclear magnetic resonance (NMR) spectroscopy. The polymers were also characterized by gel permeation chromatography (GPC). FT-IR spectra were obtained on a FT-IR spectrometer (Mattson Research Series FT/IR 1000, Madison, WI). The proton NMR ( 1 H NMR) spectra were obtained on a 500 MHz Bruker NMR spectrometer (Bruker Avance II, Bruker BioSpin Corporation, Billerica, MA) using deuterated dimethyl sulfoxide as solvent. For determination of molecular weight, the polymers were treated with diazomethane, which was generated from diazald reacted with potassium hydroxide (KOH) in water/ethanol solution at 65 °C, to obtain partially esterified products , having solubility in THF for molecular weight estimation. Molecular weights were estimated on a Waters GPC unit (Model 410 differential refractometer, Waters Inc., Milford, MA) with THF as a solvent, using standard GPC techniques and polystyrene standards.
2.3
Evaluation
2.3.1
Viscosity determination
The viscosity of the liquid formulated with the polymer and distilled water was determined at 23 °C using a cone/plate viscometer (RVDV-II + CP, Brookfield Eng. Lab. Inc., Middleboro, MA).
2.3.2
Formulation and preparation of specimens for strength tests
A two-component system (liquid and powder) was used to formulate both conventional GIC (CGIC) and light-cured GIC (LCGIC) . For CGIC, the liquid was prepared by simply dissolving the polymer in distilled water where polymer/water (P/W) ratio = 50/50 (by weight) and the powder was Fuji II glass. The mixing ratio of glass powder verse polymer liquid or powder/liquid (P/L) ratio = 2.7/1 (by weight). For LCGIC, the liquid was prepared by dissolving the light-curable polymer, CQ (photo-initiator) and DMAEMA (activator) in distilled water where CQ = 0.9% (by weight), DMAEMA = 1.8% and P/W ratio = 65:35. The powder was Fuji II LC glass where P/L ratio = 2.7/1. Two-component GICs Fuji II where P/L ratio = 2.7/1 and Fuji II LC where P/L ratio = 3.2/1 were applied and prepared per manufacturers’ instructions.
Specimens were fabricated at room temperature according to the published protocol . Briefly, the cylindrical specimens were prepared in glass tubing with dimensions of 4 mm in diameter × 8 mm in length for compressive strength (CS) and 4 mm in diameter × 2 mm in thickness for diametral tensile strength (DTS) tests. The rectangular specimens were prepared in a split Teflon mold with dimensions of 2 mm in width (or breadth) × 2 mm in thickness (or depth) × 25 mm in length for flexural strength (FS) test. For CGIC, the specimens were removed from the mold after conditioning in 100% humidity for 15 min and then conditioned in distilled water at 37 °C for 24 h prior to testing. For LCGIC, the specimens were exposed to blue light (EXAKT 520 Blue Light Polymerization Unit, EXAKT Technologies, Inc., Oklahoma City, OK) for 2 min, conditioned in 100% humidity for 15 min, and removed from the mold, followed by conditioning in distilled water at 37 °C for 24 h prior to testing, unless specified.
2.3.3
Strength measurements
CS, DTS and FS tests were performed on a screw-driven mechanical tester (QTest QT/10, MTS Systems Corp., Eden Prairie, MN), with a crosshead speed of 1 mm/min, where the FS test was conducted in three-point bending with a span of 20 mm between supports. Six to eight specimens were tested to obtain a mean value for each material or formulation in each test. CS was calculated using an equation of CS = P / πr 2 , where P is the load at fracture and r is the radius of the cylinder. DTS was determined using an equation of DTS = 2 P / πdt , where P is the load at fracture, d the diameter of the cylinder, and t is the thickness of the cylinder. FS was obtained using an equation of FS = 3 Pl /2 bd 2 , where P is the load at fracture, l the distance between the two supports, b the breadth of the specimen, and d is the depth of the specimen.
2.3.4
Statistical analysis
One-way analysis of variance (ANOVA) with the post hoc Tukey-Kramer multiple range test was used to determine significant differences of strengths among the materials in each group. A level of α = 0.05 was used for statistical significance.
3
Results and discussion
3.1
Characterization
Fig. 2 shows the FT-IR spectra for the monomers and polymers used and synthesized in the study. Fig. 2 a shows the FT-IR spectra for MEA, pentaerythritol (4-arm OH), 4-arm CTA (4-arm SH), star-shaped poly(AA-co-IA) and GM-tethered star-shaped poly(AA-co-IA). The characteristic peaks are listed below: (a) MEA: 3600–2400 (O–H stretching) for carboxyl group, 3093, 2680, 1294 and 755 (stretching and deformation) for thiol group; 1713 (C O stretching) and 1209, 1078 as well as 829 (O–H and C–O deformation and stretching) for carboxyl group; and 2929, 2569, 1418, 1153, 1078 and 996 (C–H stretching and deformation) for methylene group. (b) Pentaerythritol: 3306, 1454, 1380, 1278 and 874 (stretching and deformation) for hydroxyl group; and 2949, 2880, 1408, 1129 and 1016 (C–H stretching and deformation) for methylene group. (c) 4-Arm CTA: 2937, 2566, 1469, 1273 and 755 (stretching and deformation) for thiol group; 1736 (C O stretching) and 1469 (C–O deformation) for carbonyl group; and 2962, 1407, 1145 and 1022 (C–H stretching and deformation) for methylene group. (d) Star-shaped poly(AA-co-IA): 3600-2400 (O–H stretching), 1719 (C O stretching) and 1402, 1254, 1018 as well as 797 (O–H and C–O deformation and stretching) for carboxyl group; and 2963, 2572, 1451, 1184, 1094 and 902 (C–H stretching and deformation) for methylene group. (e) GM-tethered star-shaped poly(AA-co-IA): 3600–2400 (O–H stretching), 1717 (C O stretching), and 1402 as well as 1254 (O–H and C–O deformation and stretching) for carboxyl group; 1638 (C C bending) and 813 (C C deformation) for C C group; 3445, 1298 and 951 (O–H stretching and deformation on GM); and 2954, 2573, 1451, 1175, 1094, 1018 and 797 (C–H stretching and deformation) for methylene and methyl groups. Formation of the peaks at 3600–2400 for associated carboxyl group, 1717 for carbonyl group, 1638 and 813 for C C group and 1298 and 951 for OH group confirmed the formation of the GM-tethered star-shaped poly(AA-co-IA) copolymer.
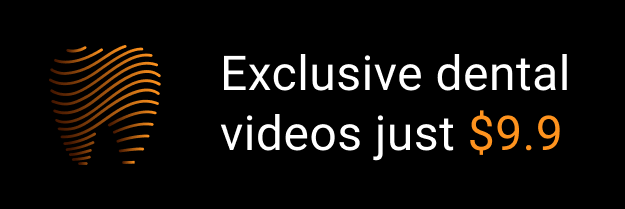