Abstract
Bridging a nerve defect is sometimes necessary to achieve nerve regeneration after injury. Different methods and conduit designs have been considered, but only isograft transplants or prefabricated conduits are available. This study presents a comparison of prefabricated conduits and isograft transplants in rats, with the aim of making suggestions for clinical settings. In rats of inbred strains LEW and DA, a 1.5 cm defect of the sciatic nerve was reconstructed by isograft ( n = 10) or conduit ( n = 10). Untreated rats ( n = 10), sham-operated rats ( n = 10) and nerves of the non-operated contralateral limb served as controls. Regeneration was evaluated by histomorphological examination and with walking track analysis of the ankle stance angle (ASA) and the sciatic functional index (SFI). After 16 weeks, myelinization and ASA in the conduit group were significantly superior to that in the isograft group. There was no significant difference in SFI between the groups. Reconstruction in the isograft group showed a negative impact on the non-operated side. Conduits and isografts did not reach the morphological or functional levels of untreated or sham-operated animals. The results suggest preferential conduits should be used for nerve reconstruction.
Lesions, including complete axonotmesis of peripheral nerves, appear in the head and neck area after accidents and as a result of destructive growth processes; they are within the scope of elective and regulative surgical interventions . The peripheral nerves have a greater potency for nerve regeneration than nerve fibres of the central nervous system, but an entire restitutio ad integrum is rare after axonal lesions . Despite intensive efforts in the field of peripheral nerve injury and regeneration, it remains difficult to achieve full functional recovery following nerve lesions in humans. With regard to nerve injuries of the face and neck, loss of sensory perception in the cheeks, tongue and lips, and the lasting failure of the arbitrary musculature results in facial paralysis, gulp disturbance and deficits in lifting the upper extremities, which interferes with the quality of life . Primary continuity restoration free of tension is currently the best treatment for fresh nerve injuries. Progress in microsurgical technology and materials means that these nerve injuries can be treated at an early phase and continuity of the nerve can be restored . Factors such as substance losses, extensive laceration of axonal structure, delay in surgical care and serious accompanying injuries can prevent timely nerve reconstruction and require bridging of the nerve defects. Before the advent of tissue engineering, autografts were the only therapeutic approach to such injuries. This involves harvesting autologous nerve tissue and its transplantation at the site of primary injury.
Several problems can arise from nerve autografting, including denervation distal to the donor site and neuroma formation. There are a limited number of suitable autologous sites available for nerve harvesting, and the donor graft or grafts may be insufficient to guide and support axonal regeneration . Over the past two decades there have been numerous efforts to find better and more efficient biological or synthetic conduits as alternatives to autografts . Different tubular models have been used for nerve regeneration in experimental animal studies . The ability of regenerating neurites to penetrate through the structurally altered extracellular matrix, surrounding tissues, and infiltrating cells at the injured site to reach synaptic targets has an important role in the regeneration of peripheral nerves. Treatment of peripheral nerve defects is aimed primarily at recreating continuity of the nerve to allow axonal re-growth into the nerve stump distal to the lesion. In nerve conduits within the scope of the originating axonal regeneration, the fortified environment contains all the neurotrophic factors for physiological regeneration. The distance between the axonal stumps is quickly bridged by longitudinal growth along the inner surface of the nerve conduits originating fibrin matrix as a guide for regenerating axons. Aberrations of nerve fibres, and thereby possibly the originating neuroma, can partly be avoided. The literature suggests that for successful axonal regeneration about a nerve conduit, a critical defect length of 1.5–2 cm (the distance that can be bridged within the scope of physiological nerve regeneration in 4–6 weeks) cannot be exceeded in a rat model . There are reports of complications after implantation of nerve conduits including an increased infection risk, disturbances in the healing process, allergic reactions, hypersensitivity and painful scars as a result of foreign body reactions. Implantation of nerve conduits can also lead to dislocation and nerve compression .
Recent clinical studies with nerve gaps of less than 2 cm have shown successful nerve regeneration with nerve conduits of different designs and materials. A standard model or method, including morphological and functional parameters to evaluate and to compare results, is not available. The objective of this study was to establish a standardized method including these parameters for use in further studies, and to use the method to evaluate the outcome of nerve reconstruction with nerve conduits and isograft transplants compared with untreated animal controls.
Materials and methods
All experiments were carried out in accordance with the NIH regulations for animal care, and an animal use protocol was approved by the Animal Care and Use Committee of the state. Every effort was made to minimize the number of animals and their suffering.
Animal surgery and transplantation
Two inbred rat strains were used. Adult male Lewis (LEW/HanHsd; n = 20; weight: 140–150 g) and Dark Agouti rats (DA/OlaHsd; n = 20; weight: 140–150 g) were purchased from a commercial dealer (Harlan Winkelmann GmbH, Borchen, Germany) and used at an age of 12 weeks. Animals were assigned randomly to one of four groups (5 rats of each strain per group). Group I rats received no treatment and served as controls for all other groups. Surgical procedures were performed under deep anaesthesia (40 mg of chloral hydrate per 100 g body weight) and aseptic conditions. Rats in groups II–IV underwent a gluteal muscle-splitting incision with exposure and neurolysis of the sciatic nerve on the left side. Rats in group II served as a sham-operated group, after exposure of the sciatic nerve and closure of the incision. In groups III and IV, the nerve was resected over a distance of 15 mm, 3 mm proximal to its trifurcation. In group III, the gap was repaired by interposing a nerve segment grafted from animals of the same strain (isograft). Segments were transplanted between the proximal and distal stumps, and anchored with three or four 9-0 resorbable perineural microsutures at each end. The segment was sutured so that its fascicular and longitudinal orientation was preserved to ensure optimal graft repair.
The rats in group IV received conduits (NeuraGen TM , Integra Life Sciences Corporation, Plainsboro, NJ, USA) made of highly purified type I collagens (<0.2% hexosamine and <0.1% trichloroacetate as insoluble residues) from bovine deep extensor sinews. The complete description of the material processing can be found elsewhere . 1–3 months after implantation, the conduit becomes increasingly porous to guarantee a sufficient blood supply for the axonal bridge newly regenerated from Schwann cells and sprouting axons. After 9–12 months, the conduit loses its mechanical stability and it is absorbed completely after 3 years .
The nerve conduits had an inner diameter of 3 mm and a length of 2 cm. They were shortened to 1.7 cm, moistened with physiological NaCl solution, and inserted into the former nerve bed. To avoid post-surgical dislocation, a 1 mm wide fold was prepared for a seam adaptation on the muscle fascia and the inner diameter of the conduit was adapted to match the diameter of the nerve stumps. Figure 1 gives an example of the preparation of the nerves and the conduit. The procedure was carried out according to the manufacturer’s instructions. The conduit and nerve stumps were joined under surgical microscopy by epineural suture with 9-0 microsutures. Before completion of the distal connection, the conduit was filled with physiological NaCl solution and the fold was fixed onto the fascia of the muscle with 4-0 resorbable sutures. Afterwards, the wound was closed by interrupted 3-0 resorbable sutures of the muscle and skin, and was infiltrated with bupivacaine 0.5% for post-operative local anaesthesia. The contralateral limb and nerve served as an intra-animal control to assess possible functional or histomorphometric impact on the non-operated side. All animals were weighed weekly and examined for any sign of discomfort such as paralysis or self-mutilation. All animals had access to food and water ad libitum.

Histological procedure and morphometric analysis
After 16 weeks, animals were anaesthetized by application of a lethal dose of chloral hydrate. The interposed nerve segments on the transplanted side and the implanted conduits including 3 mm distal and 3 mm proximal to the nerve graft or conduit, nerve segments of the control limb and those of untreated animals were removed. Nerves were fixed in phosphate buffer (PB; sodium phosphate pH 7.2) containing glutaraldehyde and paraformaldehyde (each 2%). After fixation and rinsing in PB, the nerves of the transplanted side (group III) and conduits (group IV) were cut into three portions corresponding to the sides proximal and distal to the nerve graft or conduit, and the nerve graft or conduit itself. Untreated nerves (groups I and II) and control nerves of the non-operated limb were treated in the same way. Nerves were cryo-protected in a PB/sucrose solution, embedded in compound and cut on a microtome into 40 μm transverse sections. After blocking with bovine serum, sections were incubated with a primary polyclonal antibody (rabbit anti-myelin basic protein, Chemicon, Burlingam, CA, USA; 1:200) overnight, and were further developed using an ABC Kit (Vector Laboratory, Inc., Billerica, MA, USA). 3.3 Diaminobenzidine (DAB) was used as a chromogen, and was intensified by the addition of NiSO 4 and CoCl 2 (each 2%). Sections were dehydrated and then a coverslip with Entellan was placed on top for examination under a light microscopic. Images of the sections were digitized at a magnification of 400× and blob analysis was performed on two randomly selected areas of 100 μm × 100 μm using image analysis software (Matrox Inspector Version 8.0, Matrix Electronic Systems Ltd., Quebec, Canada). The 100 μm sampling grid was based on statistical analysis using an optical dissector as a non-biased stereological technique. Thresholds to distinguish non-specific background myelin staining from true myelin basic protein were given by constant software setups referring to analysis of myelin basic protein (MBP)-myelinization rates in sections of untreated animals.
Functional assessment
Walking track analysis was used to measure global functional recovery following injury to the sciatic nerve. Functional nerve regeneration was evaluated by the sciatic functional index (SFI) for function of the lower limb, mainly of foot muscles , and by the ankle stance angle (ASA) recording the ankle joint angle and the function of the ankle joint muscles . To obtain data for SFI, the hind feet of the rats were dipped in dark ink and the rats walked across a wooden corridor while footprints were recorded on paper laid on the bottom of the corridor. The distance between the third toe and heel, the first and fifth toe, the second and fourth toe, and the distance from the rostral tip of the foot to that of the opposite foot were measured on the experimental side (EPL, ETS, EIT, and ETOF, respectively) and the contralateral normal side (NPL, NTS, NIT, and ETOF, respectively). The SFI was calculated as follows:
SFI = ETOF − NTOF NTOF + NPL − EPL EPL + ETS − NTS NTS + EIT − NIT NIT × 220 4
In general, an SFI of around 0% corresponds to normal function, and an index of −100% represents total dysfunction. The ASA was measured during the midstance phase of locomotion. Recordings were taken with a digital video camera (DCR-TRV900E Sony Corp., Tokyo, Japan) positioned at a distance 1 m lateral to the walking track corridor. The entire walk was recorded and representative sequences of locomotion were read into a computer, and the angle was determined from the means of two or three video frames of typical midstance phases. At 4, 8, 12 and 16 weeks post-operation, values were measured by two independent investigators who were blinded to treatment allocation. The inter-individual correlation (Pearson’s correlation) of data determined by the investigators was 0.91 for ASA and 0.94 for SFI, which demonstrated the reliability of the procedure.
Statistical analysis
Differences in data from the walking track analysis and the morphometric analysis were examined with the Mann–Whitney U -test. The level of statistically significant difference was set as p < 0.05. The normal distribution of data was tested by means of the Kolmogoroff–Smirnov test; and Pearson’s correlation coefficients were determined. Calculations were done with the statistical software SPSS version 14.0 (SPSS Inc., Chicago, IL, USA).
Results
The animals did not display distress or any secondary complications resulting from the sciatic nerve lesion, such as automutilation, pressure ulcers, loss of weight, and significant inflammatory reactions or neuroma. All animals tolerated anaesthesia without complications. There were no post-operative drop-outs in any group.
Untreated animals (group I) showed a median density of MBP per section field of 17 (±4)%, without significant difference among proximal, median or distal sections, an ASA of 45.02(±2.23) and an SFI of −3.22(±9.95). All functional and morphometric data for the experimental groups II–IV were set in relation to data from the untreated animals. There was no statistical difference among sections of the contralateral non-operated sides of each experimental group or between strains.
The trauma of the surgical exposure of the sciatic nerve of group II rats (sham) did not influence the functional outcome and the myelinization after 16 weeks. The data did not differ significantly from untreated animals. In group I and II rats, differences were not significant between the control side and the experimental side along the entire nerve segment investigated. The fascicular structure of the nerves was intact.
The myelinization of nerves showed a significant difference between group I and II animals compared with group III (isograft) and group IV (conduit) rats ( p < 0.05; Figs 2 and 3 ). In LEW and DA rats in group III, a negative implication was found on the myelinization of the non-operated control sides. Here, myelinization was decreased significantly compared with group I rats ( p < 0.05). The myelinization of the control and the experimental side was significantly higher in group IV rats compared with group III rats. In both groups the myelinization was significantly reduced in all sections of the experimental side compared with the control sides and with group I and II rats ( p < 0.05).


The ASA of the control sides of group IV rats did not differ significantly from that of group I rats ( Table 1 ). During the course of 16 weeks, the ASA values of the experimental side did not reach those of group I and II rats. In LEW rats of group IV a significant increase in the ASA of the experimental side could be observed between 4 and 8 weeks ( p = 0.0079, regression-gradient G Regr = 1.6155) reaching the ASA level of DA rats of this group. In comparison, the ASA of DA rats did not change significantly over the weeks ( p > 0.05, G Regr = 0.6612). In DA and LEW rats of group III, the experimental side did not show a significant increase of ASA compared with group I and II rats. The ASA was significant lower than in group IV at 8, 12 and 16 weeks ( p < 0.05). The control side in group III was negatively affected and differed significantly over 16 weeks from that of group I and II rats ( p < 0.05).
4 Weeks | 8 Weeks | |||||
---|---|---|---|---|---|---|
II a | III a | IV a | II a | III a | IV a | |
LEW co b | ||||||
Mean | 100.33 | 36.35 † | 94.62 | 101.99 | 32.99 † | 101.88 |
SD c | 5.11 | 7.82 | 7.58 | 3.74 | 9.01 | 9.56 |
LEW ex d | ||||||
Mean | 93.05 | 24.05 * | 31.31 * | 99.31 | 18.45 * | 42.05 * |
SD c | 2.55 | 14.31 | 3.54 | 5.61 | 6.15 | 6.78 |
DA co b | ||||||
Mean | 99.45 | 41.06 † | 97.04 | 95.72 | 34.7 † | 101.9 |
SD c | 5.51 | 9.94 | 10.23 | 4.31 | 7.85 | 7.37 |
DA ex d | ||||||
Mean | 98.6 | 27.28 * | 50.29 * | 102.34 | 22.31 * | 41.58 * |
SD c | 5.91 | 8.38 | 8.73 | 5.31 | 9.53 | 9.87 |
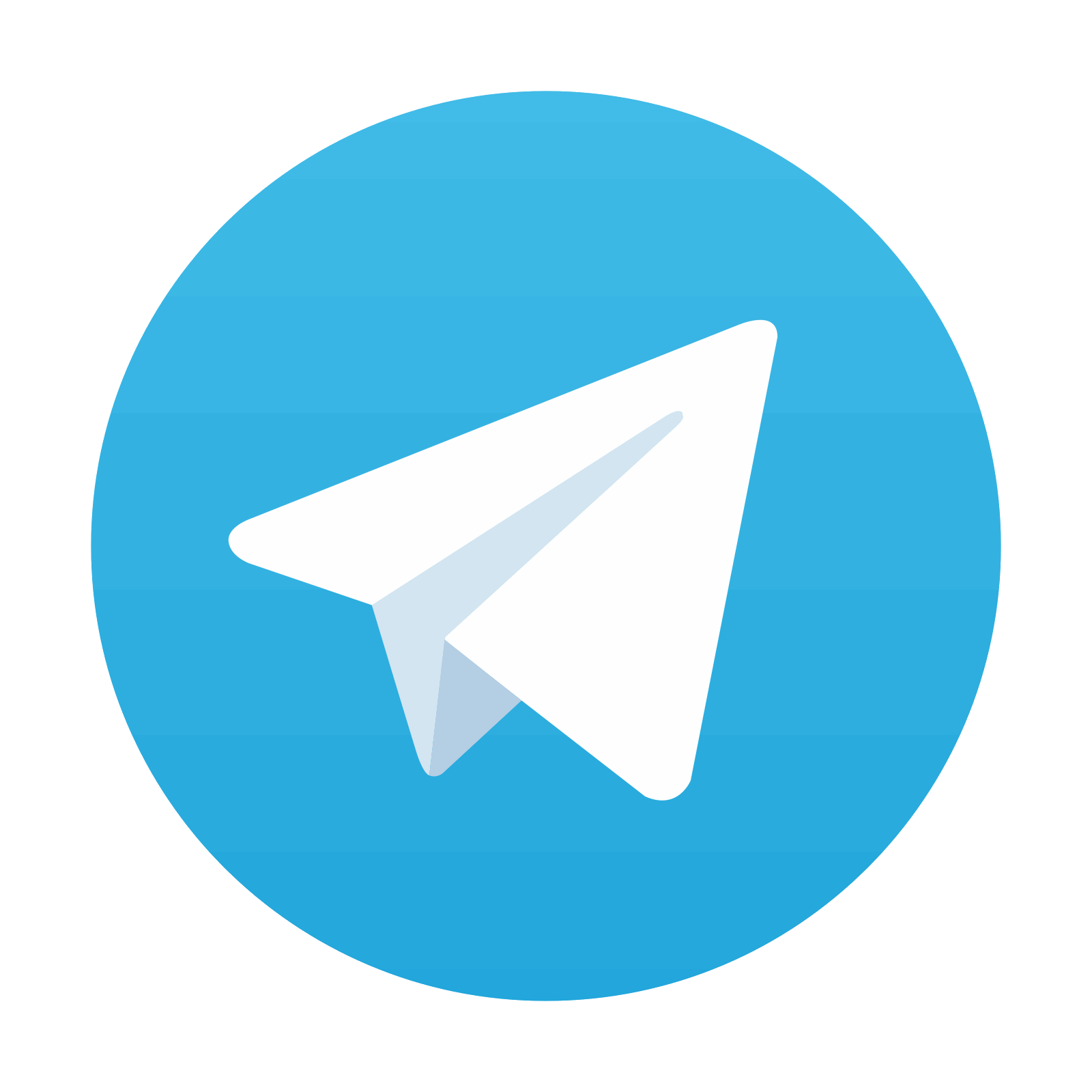
Stay updated, free dental videos. Join our Telegram channel

VIDEdental - Online dental courses
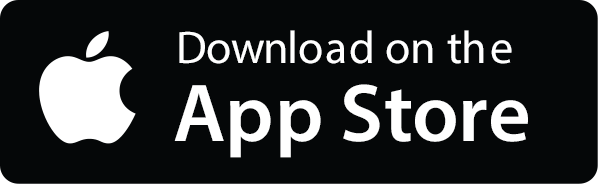
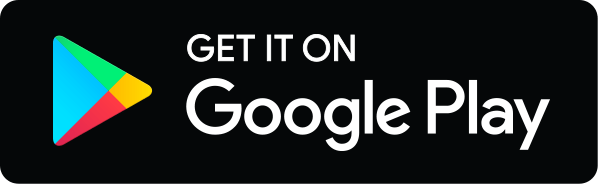
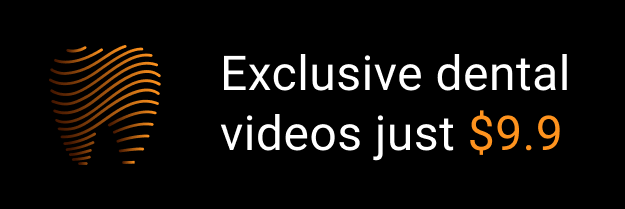