Introduction
This cross-sectional study aimed to analyze the precision and trueness of dental models produced using 2 rapid prototyping 3-dimensional printers.
Methods
A digital crowded maxillary arch with a T-shaped base and 2 hemispheres of 2.5 mm radius was printed 10 times with a stereolithography apparatus (SLA) and digital light processing (DLP) in the highest precision and minimum layer thickness (z-resolution) mode. The copies were scanned using the D710 3Shape desktop scanner and assessed for precision and trueness via arch superimpositions and hemisphere measurements. Mann-Whitney U tests were used to compare trueness and precision among printers. Hemisphere radius was compared with the reference measurement and between 3-dimensional printers using 1 sample and independent Student t tests, respectively (α = 0.05).
Results
The root mean square values of arch superimpositions showed statistically significant differences between the 2 techniques, both for precision ( P = 0.011): SLA (46.8 μm ± 13.5); DLP (111.1 μm ± 71.9), and trueness ( P = 0.015): SLA (61.1 μm ± 9.8); DLP (99.8 μm ± 47.2). The color map model analysis indicated greater distortion on premolar and molar surfaces, with a higher range of contraction on the SLA and both contraction and expansion on the DLP. Anterior and posterior hemisphere radius registered increased values with DLP (1.7% and 0.49%) and reduced values with SLA (0.6% and 0.7%); however, only the anterior SLA hemispheres revealed a significant decrease from the reference value ( P = 0.037).
Conclusions
In this study, the SLA printer was significantly different from the DLS printer, with the highest precision and trueness.
Highlights
- •
SLA 3-dimensional printed models showed higher precision and trueness than DLP.
- •
The SLA and DLP printers studied were accurate enough for orthodontic applications.
- •
Models should be digitally trimmed on the teeth or on the gingival margin to prevent distortion.
Over the last years, the rapid development of digital technology has raised the interest in the dentistry community for increasing its use in daily practice. In orthodontics, 3-dimensional (3D) imaging and additive manufacturing (AM) are continuously advancing to enhance treatment efficiency and to provide a customized, comprehensive treatment. This technology is also referred to as rapid prototyping (RP) or 3D printing.
The accuracy of 3D printed replicas is defined by both precision and trueness. Precision is defined as the closeness of agreement on printed models within each printer and trueness as the difference of each 3D replica from the reference model (ISO 15725-1). As an outcome, bringing accuracy to the comprehensive orthodontic treatment involves process optimization, less iatrogenic effects, and the possibility of reducing the overall treatment time. As the 3D printing market is rapidly growing, a variety of printers involving different techniques, brands, and prices are becoming available. This creates a challenge for professionals when deciding which is the most suitable option for the practice needs. One of the most commonly used AM technology in dentistry is vat polymerization-based printing, in which liquid photopolymer in a tray is selectively cured by light-activated polymerization, layer by layer. , In the scientific literature, studies can be found which analyze the accuracy of different RP systems, the effect of print layer height, , the model base design, and the impact of orientation and surface quality. ,
Dietrich et al, evaluated the effect of crowding on the accuracy of 2 maxillary crowded arches printed 10 times using SLA and polyjet printing techniques, one with little crowding and a wide arch, and the other with marked anterior crowding and a shorter arch. Higher trueness was found in polyJet printed models, although SLA revealed better precision. In another study, orthodontic patients with mild, moderate, and severe crowded arches were selected, in which white stone models were compared with color jet printing technique, Z Printer 450. The authors concluded that RP replicas were not acceptable to replace stone models, especially for appliance construction.
The SLA system, the DLP, and 2 other RP techniques were compared by Kim et al, using 5 replicas per arch and straight maxillary and mandibular dentitions. Results showed significant differences in trueness and precision among the 4 printers, with the polyjet technique presenting the highest accuracy.
In the literature, the few studies in which the vat polymerization printers were used present a limited number of printed replicas. Moreover, most of the research was done in aligned models, but crowded arches are frequently seen in the orthodontic practice. Models were also printed without reference structures of well-known dimensions, which can be used for calibration.
There is also a literature gap on the influence of different model cuts on accuracy measurements because of error and bias in the superimposition method. In one study, the base of the models was removed to avoid superimposition distortions. To our knowledge, no studies have examined the influence of different digital model cuts on superimposition, which can introduce error and bias on the results.
The primary aim of this study was to analyze the precision and trueness of dental models using 2 RP techniques (SLA and DLP). For the overall sample, the null hypothesis was that no differences would be found between SLA and DLP printing techniques in trueness and precision when producing 3D dental models. A secondary aim was to evaluate the influence of different cuts (base, gingiva, and teeth) on superimpositions. The null hypothesis was that no differences would be found when superimposing models with different cuts.
Material and methods
A physically crowded maxillary arch was created using 14 maxillary acrylic teeth from a Typodont PE-ANA001 (Nissin Dental Products Inc, Kyoto, Japan) and pink wax (No. 02-4110, Pink Plattenwachs; Al Dente Dentalprodukte, Goslar, Germany) to involve the dental roots. The model was scanned using a desktop scanner (D710; 3Shape, Copenhagen, Denmark) into a computer-aided design software (3Shape, Copenhagen, Denmark). The scanned file was modified using the computer-aided design software to replace the palatal area with a T-shape base, with a bar connecting the second molars and a bar connecting the posterior structure to the midline to prevent transverse distortions ( Fig 1 , A ). Two hemispheres with a 2.5 mm radius were placed at the base onto the bars, separated by a distance of 20 mm between both geometric centers. These were used as standard measurements. The modified digital model was converted to stereolithography file format (STL) and used as the reference model.

Two vat photopolymerization 3D printers were included in this study, SLA and DLP ( Table I ). The SLA printer (Form 2: Formlabs, Somerville, Mass) was set to produce replicas using its minimum layer thickness (25 μm). Beige dental model resin (Formlabs) was used as the printing material for this technique. The postprocessing method included manual cleaning with 2 consecutive isopropyl alcohol 99.9% baths for 10-minutes each, as recommended by the manufacturer. After cleaning, models were air-dried at room temperature and postcured in a UV-light curing box (Form Cure; Formlabs) at 60°C for 30-minutes for a final polymerization.
Technique | Printer parameters | Selected settings | |||||
---|---|---|---|---|---|---|---|
3D printer | x-y resolution | z-resolution (layer thickness) | Inclination to platform build | Model structure | Print material | Print time | |
Laser-based stereolithography or SLA | Form 2; Formalabs | 140 μm | 25 μm (100, 50, 25) | Parallel to build platform (0°) | Hollow | Dental Model Photoreactive resin, beige | 615 min |
DLP | D40 II; RapidShape | ± 34 μm | 35 μm (35, 50, 100) | Parallel to build platform (0°) | Hollow | PhotoDent model resin, beige opaque | 190 min |
In the DLP printer (D40 II; RapidShape, Heimsheim, Germany), the minimum layer thickness (35 μm) was also selected to create the models. Beige photopolymer resin (NextDent Model 2.0, 3D Systems, Soesterberg, the Netherlands) was used as the printing material. After production, replicas were manually rinsed in 2 consecutive methyl alcohol 99.9% baths, air-dried at room temperature, and postcured in a UV-light curing box (NextDent LC-3D Print Box, 3D Systems, Soesterberg, the Netherlands) at 60°C for 30-minutes for final polymerization.
From the reference digital model, support was added to avoid inaccuracy of the initial layers ( Fig 1 , B ), and then each printer produced 10 replicas (SLA, 10; DLP, 10), with the occlusal plane parallel to the build platform ( Fig 1 , C ). The support was then manually removed, the copies were scanned using a previously calibrated D710 desktop scanner (3Shape, Copenhagen, Denmark) and assessed for precision and trueness via arch superimpositions and hemisphere measurements. Once scanned, the acquired digital models were imported into reverse-engineering software (Geomagic Studio 2018.1.0; 3D Systems Inc, Morrisville, NC). To determine superimposition deviations, 3D models were trimmed using 3 different cutting methods ( Fig 2 ). The cut on the base removed the excess of material scanned during the models’ digitalization process. The gingiva cut was made 2 mm below the gingival margin of all teeth. On the teeth cut, trimming was performed next to the clinical crown, after the contour of the gingival margin. The reliability of the superimposition procedure was tested between the 3 groups, first with a three-point registration software tool to obtain a rough alignment of the surfaces and then with the best-fit alignment tool to check for deviations from the reference model. Finally, a color map was created with the 3D-compare tool to visualize displacement from the reference model surface, setting 0.5 mm as maximum and minimum range and ± 0.05 as specific tolerance. All cuts and superimpositions were repeated (T2) by the same investigator (CRM) within a 15-day interval between them.

Precision was defined as the closeness of agreement over 10 repeated 3D model replicas in each printer, and trueness was assessed by the comparison of each 3D replica with the reference model (ISO 15725-1). ,
Regarding arch superimposition data, trueness was measured by superimposing SLA and DLP replicas with the reference model STL file, and precision was determined by superimposing the replicas within each printer category, as already described.
In Geomagic, the Auto Segment tool was used to find geometric figures on the digital model mesh. Search sensitivity was set at 65% to identify hemispheres, and radius was found using region information.
Statistical analysis
On the basis of previously published results, a minimum sample size of 10 replicas per group was determined, with power set at 85% and alpha at 0.05 (G-Power, version 3.1.9.2; Heinrich-Heine-Universität Düsseldorf, Düsseldorf, Germany) to detect a clinically significant difference of 0.1 mm.
All statistical analysis and graphic representations were computed using SPSS software (version 25.0; IBM, Armonk, NY). Descriptive analysis included the mean, standard deviation, and range values for trueness and precision root mean square (RMS) values, hemisphere area, and hemisphere radius by printer category. Using the same coordinate system, RMS compared the reference data with the measurement data and calculated the mean value of deviations. A higher RMS value revealed a higher deviation from the reference data. The RMS values were calculated using the following formula:
RMS=1n∑i=1n(xref−xi)2
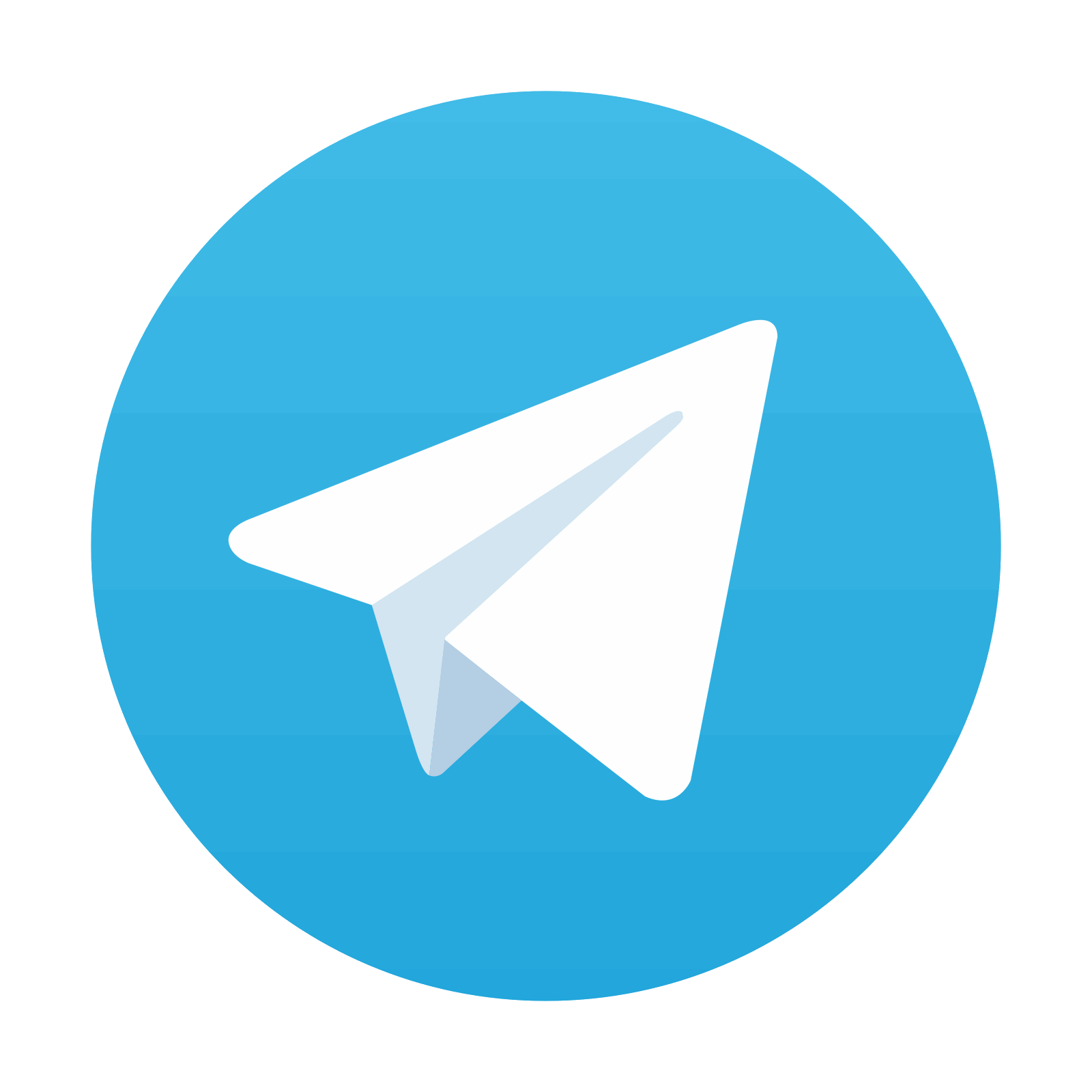
Stay updated, free dental videos. Join our Telegram channel

VIDEdental - Online dental courses
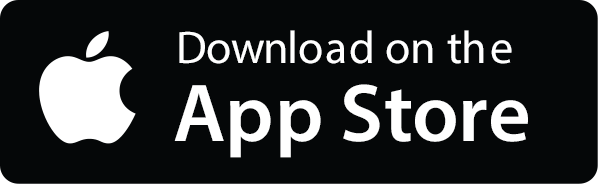
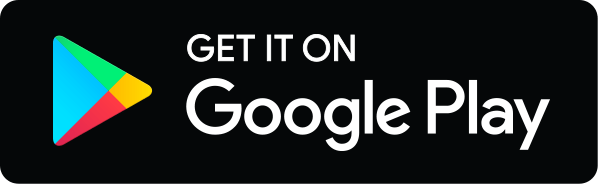
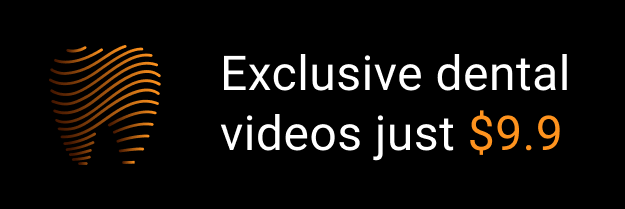