Abstract
This feasibility study evaluated whether contrast enhanced ultrasound (CEU) was able to assess free flap perfusion following free tissue transfer in the head and neck region. Thirty-six patients underwent standard clinical monitoring (SCM) and CEU postoperatively. The time taken for each technique to detect flap failure was recorded. Qualitative CEU analysis by visual assessment predicted survival in 30/30 (100%) and failure in 5/6 (83%) flaps with sensitivity, specificity, positive (PPV) and negative (NPV) predictive values of 100, 86, 97 and 100%, respectively. Quantitative CEU measurement of blood volume ( α ) values within healthy perfused flaps was over 60 times higher than in failing flaps (8.25 ± 2.82 dB vs. 0.12 ± 0.17 dB, respectively, P < 0.0001). If a cut-off α value of <1.5 dB was used to predict future flap failure, the accuracy of the test was 100% (sensitivity, specificity, PPV, NPV). If a cut-off α value of >1.9 dB indicated flap success, the PPV and NPV are 100%. Following surgery, SCM took 76 (±15) h to detect flap failure compared with 18 (±38) h with CEU ( P < 0.05). CEU is highly accurate in its ability to distinguish between perfused and failing flaps. The technique is quick (<10 min) and capable of imaging all flap types.
Surgical resection for cancer often results in complex defects and microvascular free tissue transfer (FTT) is a common method of reconstructive surgery. The absolute failure rate for FTT in head and neck reconstruction is approximately 5%, and the proportion that has to return to theatre for salvage surgery is even higher at 16% . The salvage rates for non-buried flaps are modest at 77% but that for buried flaps is zero . Free flap failure is a devastating complication and is predominantly due to free flap ischaemia. Early detection of ischaemia is vitally important and has been shown to be directly related to salvage rates . There are several methods of free flap monitoring, most of which provide indirect estimates of perfusion. These vary from the simple to the highly complex. The most heavily relied upon is clinical observation combined with hand-held continuous wave Doppler (CWD) . Clinical observation involves regular assessment of flap colour, capillary refill time, temperature and bleeding on pinprick. This is subject to inter-observer variability, tends to detect flap ischaemia late and buried flaps cannot be assessed . Both hand-held and implantable Doppler probes (spectral) can assess flow in the arterial pedicle. Locating the pedicle vessels can be difficult, they do not assess flow within the microcirculation of the flap itself and may miss other non-arterial causes of flap compromise, resulting in low sensitivities . Other sophisticated methods in limited use include laser flowmetry, spectrophotometry, oxygen tension assessment, microdialysis, photoplethysmography, laser fluorescence and hydrogen clearance. Published reports suggest that they have met with some success but can be technically difficult to use, susceptible to artefact and are unable to assess buried flaps .
Contrast enhanced ultrasound (CEU) provides direct visual information on tissue perfusion at a microvascular level. This involves the continuous infusion of gas-filled microbubbles through a peripheral vein. The microbubbles oscillate when subjected to ultrasound and resonate with great amplitude at multiples of their resonant frequency (harmonics). The reflected signal field can be frequency-filtered to preferentially receive the harmonic signals and simultaneously suppress those of the surrounding tissue . The reflected signal therefore represents the total blood volume within the microcirculation of tissue being studied. Ultrasound destruction of the microbubbles is possible and the rate at which the microbubbles return into the tissue of interest (the rise in signal intensity) represents the tissue blood velocity. The product of tissue blood volume and velocity corresponds to tissue blood flow .
The most common application of CEU has been myocardial imaging, where it has been able to detect ischaemia and discriminate between viable myocardium and scar tissue. The adverse event rates are lower than contrast agents used in CT and MRI . No one has assessed whether CEU can be used to assess perfusion in free flaps following surgery and relate this to outcome. This feasibility study assessed whether CEU was able to assess free flap perfusion and therefore its accuracy in determining flap outcome. The authors also assessed the flexibility of CEU in imaging a wide range of buried and non-buried free flaps.
Methods
Thirty-six patients undergoing microvascular FTT for head and neck cancer were recruited into this prospective open non-randomised study between June 2005 and June 2007. Each patient underwent standard clinical monitoring (SCM) and CEU of the free flap several times following surgery. The techniques were assessed for their ability to predict the primary outcome measure – a successful or failed/failing free tissue flap. Successful was defined as a healthy tissue flap on clinical inspection 14 days postoperatively that did not require any prior intervention. A failed or failing flap was one that demonstrated evidence of ischaemia or necrosis at re-exploration surgery and would not have survived unless operated upon. The time taken by SCM and CEU to detect free flap failure was also recorded.
As this was a feasibility study designed to assess the accuracy of CEU in monitoring for healthy or ischaemic flap perfusion and its relation to outcome, the results of the CEU were not made known to the chief surgeon and not allowed to influence the decision to return to theatre, which was based on SCM alone. Ethical approval was granted by the NHS Research Ethics Committee and patient consent obtained.
For SCM, the non-buried free flaps were assessed for flap colour, temperature, capillary refill time, and bleeding on pinprick (if indicated). Both buried and non-buried flaps underwent hand-held CWD of the arterial vascular pedicle. SCM took place hourly for the first 2 days and then 4-hourly for 11 days. The flap was recorded as either healthy or not-healthy.
All patients underwent CEU 12, 24 and 48 h postoperatively. Contrast (Sonovue ® , Bracco Int SPA, Milan) was given as a continuous intravenous infusion in a 5-ml solution. Images were obtained with a Sonos 5500 ultrasound platform (Philips Medical Systems, UK) with a 5-MHz transducer. Tests were executed by one of two clinicians involved in the surgery together and a cardiologist operating the ultrasound platform; although the complete imaging protocol could easily be achieved by a single operator. Flap identification, orientation and margin delineation were made using two-dimensional (2D) imaging with a surgical instrument placed on one surface of the flap (which is clearly seen on ultrasound). Buried flaps were located using anatomical landmarks appropriate to the location of the flap. The flap was reviewed in three planes to obtain the optimum scanning plane. Following this, CEU took place in real time using power modulation mode with a mechanical index of 0.1. Microbubbles were continuously infused at a rate of 1.5 ml/min and, once a steady state was reached (60 s), the ultrasound gain, image depth, focus and sector angle settings were adjusted optimally. Following flash imaging (microbubble destruction), 15 cardiac cycles were digitally recorded in DICOM format to demonstrate tissue replenishment with microbubbles.
Three sequences were recorded at each stage. Using this technique the flap was assessed qualitatively and quantitatively. Qualitative analysis required the observer to decide visually whether microbubbles were flowing into the whole flap following microbubble destruction and decide whether the flap was perfused (yes or no). Quantitative analysis was performed offline in which free flap blood velocity and volume was calculated using QLAB Advanced Quantification software (Philips Medical Systems, Nederlands BV). A region of interest was selected in the centre of flap but away from the arterial pedicle. The software generated a replenishment curve following bubble destruction according to the replenishment curve-fit-model: y ( t ) = α (1 − exp(− βt )) + C (where α is peak intensity, β is refilling rate, t is time and C is baseline intensity). The frame immediately following bubble destruction was selected to represent background signal intensity and this was subtracted to zero the software. All readings were carried out with results blinded to the surgeon in charge.
For statistical analysis, CEU and SCM were compared in their abilities to predict the primary outcome measure as defined above. Sensitivities, specificities, positive and negative predictive values were calculated for each technique. A paired t -test was used to compare the times taken by each method to detect flap failure. A P value of <0.05 was taken as statistically significant. Logistic regression was used to model the relationship between flap outcome and the α value. Odds ratios were obtained for the chances of flap success per dB change in the α value.
Results
Thirty-six patients undergoing FTT for maxillofacial cancer were recruited between June 2005 and May 2007. The mean (range) age of the patients was 57 (29–82) years; 22 (61%) were male. Eight (22%) flaps were buried and 28 (78%) were non-buried. Table 1 shows that the majority of procedures performed were glossectomies ( N = 14, 39%) and the main FTT method used was the radial forearm fasciocutaneous flap ( N = 21, 58%). Six (17%) flaps failed (one buried) and 30 (83%) were successful (five buried). Overall success rate (including non-recruited patients and following salvage) was 94%. There were no adverse incidents associated with the administration of contrast agent. 2D imaging, used to define the flap boundaries, was quick and simple to perform but localisation with a surgical instrument was not possible with buried flaps. Identification of the pedicle was straightforward but occasionally maintaining an ideal image for Doppler readings was difficult due to the small size of the vessels and movement (voluntary or related to normal respiratory movements or swallowing). The flap boundaries were clearly visible due to the different characteristics of the flap tissue adjacent to native tissue (e.g. radial fascia adjacent to native tongue muscle). Differences in intra-flap tissue vascularity could also be seen, for example skin, muscle and fascia could be differentiated on the basis of their reflectivity and contrast intensities. Bony flaps cast acoustic shadows in the far field but the tissue in this area was visible when imaged in a different plane.
Free tissue flap | N (%) | Type of ablative surgery | N (%) |
---|---|---|---|
Radial forearm fasciocutaneous | 21 (58) | Glossectomy (all types) | 14 (39) |
Osteomyocutaneous fibula | 2 (6) | Floor of mouth (FOM) | 4 (11) |
Jejeunal | 3 (8) | Maxillectomy | 4 (11) |
Osteomyocutaneous scapula | 2 (6) | Mandibulectomy | 6 (17) |
Anterolateral thigh | 1 (3) | Laryngopharyngectomy | 5 (14) |
Osteomyocutaneous deep circumflex iliac artery | 2 (6) | Scalpectomy | 1 (3) |
Serratus anterior | 3 (8) | Oropharyngeal | 1 (3) |
Latissisimus dorsi | 2 (6) | Buccal mucosa | 1 (3) |
SCM
SCM was not possible in any of the buried flaps and predicted all successes and failures accurately, but the time taken to detect flap failure was 76 (±15) h. Hand-held CWD was possible in all 36 flaps, but this technique suggested that all flaps were healthy and did not detect any flap failures (sensitivity 100%, specificity 50%).
Qualitative CEU analysis
CEU was possible in all patients. Qualitative CEU correctly predicted survival in 30/30 (100%) flaps and failure in 5/6 (83%). This represented a sensitivity, specificity, positive and negative predictive value of 100, 86, 97 and 100%, respectively. Qualitative CEU was incorrect in one case, in which it predicted survival in one flap that went on to fail. In the five flaps that CEU correctly predicted to fail, the mean time to failure detection was 12 h, which was significantly earlier than SCM ( P < 0.05). Review of the quantitative analysis results in the incorrectly predicted case suggested this flap was functioning at the 12- and 24-h scans but had failed by the last scan. Flaps that went on to fail showed a complete lack of signal and were straightforward to identify with an ‘all-or-nothing’ appearance. Figures 1 and 2 show two different flap types failing. Figure 3 shows the same patient as in Fig. 2 following successful revision surgery 3 months later (contralateral deep circumflex iliac artery flap).



Quantitative CEU analysis
Table 2 shows the mean (range) signal intensities ( α ) in successful and failed free tissue flaps at different times. Overall, the mean values per time point in the successful flaps (8.25 ± 2.82 dB) were more than 60-fold higher than in the failed group (0.12 ± 0.17 dB) ( P < 0.0001), the latter were close to zero. Figure 4 A shows a time replenishment curve for a well-perfused flap with the curve gradient related to the refill kinetics. The plateau signal value ( α ) is high indicating a successful flap. Figure 4 B shows the replenishment curve for a similar radial flap but one that is not perfused. The plateau value is very low and even the raw graphical data appears variable due to the expanded dB scale. Table 3 shows that five of the failed flaps had poor vascular flow on CEU at the initial 12-h scan. Failed flap number 5 had normal flow on quantitative CEU at 12 and 24 h but no flow subsequently. SCM suggested this flap to be clinically failing at 60 h and this was confirmed at exploratory surgery. The results show that flap ischaemia is likely to have occurred between the 24- and the 48-h scan as suggested by quantitative analysis at 48 h. The analysis included flap number 5 as a correct prediction for flap status at the 12- and 24-h time points and as a correct prediction for eventual outcome at the 48-h time point.
12 h | 24 h | 48 h | |
---|---|---|---|
Successful FTT | 8.93 ± 2.69 (3.44–14.85) | 8.21 ± 2.28 (4.64–13.99) | 8.38 ± 2.44 (4.64–13.86) |
Failed FTT | 0.16 ± 0.11 (0–0.3) | 0.04 ± 0.04 (0.01–0.13) | 0.18 ± 0.22 (0–0.59) |

No. | Flap type | Buried (B)/not buried (NB) | CHI signal intensities (mean dB) | Flap success (S)/failure (F) | ||
---|---|---|---|---|---|---|
12 h | 24 h | 48 h | ||||
1 | SA | B | 0.10 | 0.02 | 0.09 | F |
2 | ODCIA | NB | 0.12 | 0.03 | 0.27 | F |
3 | RFF | NB | 0.30 | 0.01 | 0.59 | F |
4 | LD | NB | 0.00 | 0.03 | 0.04 | F |
5 | RFF | NB | 7.90 | 9.70 | 0.00 | F |
6 | RFF | NB | 0.20 | 0.13 | 0.12 | F |
7 | SCAPULA | NB | 11.27 | 6.34 | 12.16 | S |
8 | SA | B | 5.80 | 4.91 | 4.64 | S |
9 | LD | B | 5.20 | 11.29 | 5.93 | S |
10 | JEJUNAL | B | 7.20 | 6.70 | 7.00 | S |
11 | JEJUNAL | B | 10.44 | 10.25 | 13.50 | S |
12 | SCAPULA | B | 8.48 | 9.47 | 9.38 | S |
13 | FIBULA | B | 7.10 | 5.20 | 4.83 | S |
14 | RFFF | B | 9.38 | 7.18 | 9.36 | S |
15 | SA | NB | 3.44 | 7.29 | 7.17 | S |
16 | RFFF | NB | 8.48 | 7.13 | 7.05 | S |
17 | RFFF | NB | 7.85 | 7.85 | 6.49 | S |
18 | RFFF | NB | 11.87 | 13.10 | 13.86 | S |
19 | RFFF | NB | 10.74 | 6.38 | 7.60 | S |
20 | RFFF | NB | 5.47 | 8.85 | 8.75 | S |
21 | RFFF | NB | 13.72 | 10.50 | 13.04 | S |
22 | RFFF | NB | 9.05 | 6.89 | 9.48 | S |
23 | RFFF | NB | 7.79 | 8.38 | 9.81 | S |
24 | ODCIA | NB | 8.90 | 6.92 | 6.87 | S |
25 | RFFF | NB | 9.30 | 7.93 | 8.05 | S |
26 | ALT | NB | 12.70 | 8.63 | 11.00 | S |
27 | RFFF | NB | 6.24 | 9.90 | 6.10 | S |
28 | RFFF | NB | 9.20 | 9.83 | 9.90 | S |
29 | RFFF | NB | 5.99 | 4.87 | 6.96 | S |
30 | RFFF | NB | 5.28 | 7.80 | 6.90 | S |
31 | RFFF | NB | 14.85 | 4.64 | 7.52 | S |
32 | RFFF | NB | 9.77 | 8.97 | 8.67 | S |
33 | RFFF | NB | 10.17 | 7.90 | 7.33 | S |
34 | FIBULA | NB | 10.96 | 6.79 | 7.20 | S |
35 | RFFF | NB | 10.55 | 13.99 | 9.33 | S |
36 | JEJUNAL | NB | 10.81 | 10.53 | 5.57 | S |
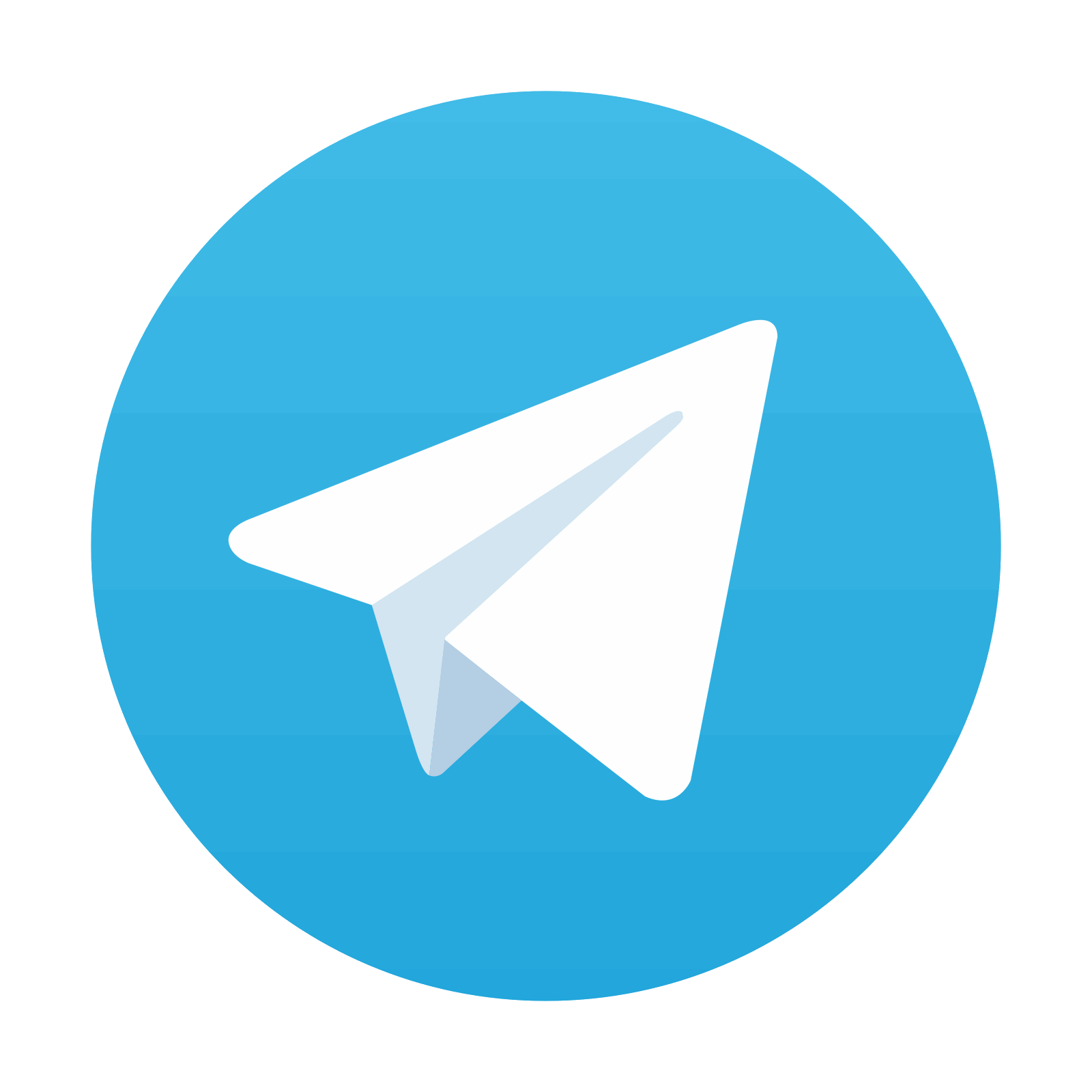
Stay updated, free dental videos. Join our Telegram channel

VIDEdental - Online dental courses
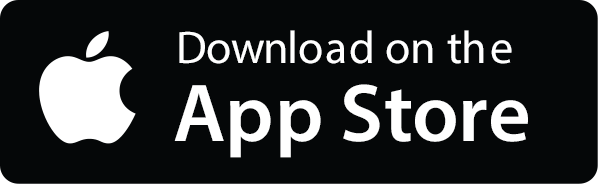
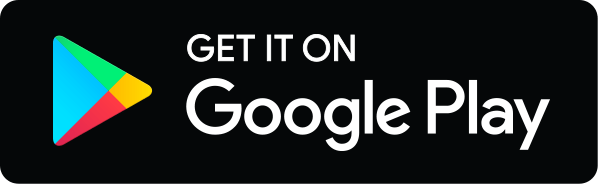
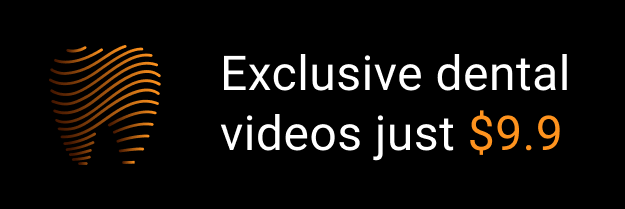