Abstract
Objectives
The objectives of this study were to develop a chitosan bilayer membrane containing microspheres with sustained TGF-β 1 release to enhance odontoblast-like cell function in vitro, and to investigate pulp-capping in a dog model to promote reparative dentin formation in vivo for the first time.
Methods
A chitosan bilayer membrane was synthesized consisting of a dense film on one side and a macroporous sponge on the other side. The rationale was to use the dense film to block the perforated pulp from bacterial invasion, and the porous sponge to be loaded with microspheres containing TGF-β 1 (MS-TGF) for sustained release. Pulp capping in 48 teeth of six beagle dogs was performed to test four groups: Control with no pulp capping material, commercial Dycal, chitosan membrane without MS-TGF, and chitosan membrane with MS-TGF. The dog teeth were harvested for histological analysis at two time points (10 and 60 d).
Results
The spongy side of the membrane had macropores with a mean size of 151 μm. The porosity of the membrane was 83%. Chitosan microspheres containing TGF-β 1 showed sustained release, gradually releasing 42% of the TGF-β 1 in 7 d. The proliferation of odontoblast-like cells on membrane with MS-TGF was much greater than that without TGF ( p < 0.05). At 10 d, H&E staining revealed mild to moderate pulp inflammation in all four groups, with no dentin bridge formation. At 60 d, pulp inflammation disappeared, but there was no reparative dentin bridge in the group with no pulp-capping material. Chitosan membranes with MS-TGF generated reparative dentin with a thickness of (142 ± 29) μm, 3–6 times thicker than that with Dycal or chitosan bilayer membrane without TGF ( p < 0.05).
Significance
A novel chitosan bilayer-microsphere construct containing TGF-β 1 for pulp-capping generated 3–6 times more reparative dentin than the controls in a dog model for the first time. The chitosan bilayer-microsphere construct with growth factor delivery may be useful for a wide range of dental and regenerative medicine applications.
1
Introduction
The repair of the dentin–pulp complex after injury depends on tertiary dentinogenesis to reconstitute normal tissue continuum at the pulp–dentin border . Current vital pulp therapy aims to treat reversible pulpal injury, to maintain pulp vitality and function, and to regulate tissue-specific processes of tertiary dentinogenesis . Previous studies suggest that a network of extracellular matrix molecules and growth factors influence tertiary dentinogenesis, and the application of dentin matrix components and growth factors can promote the biosynthetic activity of odontoblasts or odontoblast-like cells, and stimulate the formation of reparative dentin . Among these molecules and factors, transforming growth factor-beta1 (TGF-β 1 ) is considered to be deeply involved in this process and its therapeutic usage in vital pulp therapy has been investigated . Tissue engineering via the design of new biomaterials in combination with the controlled release of relevant growth factors are highly promising for dental and craniofacial repair and regeneration . Therefore, the development of novel dental materials that incorporate TGF-β 1 may be a promising strategy for successful regenerative pulp treatment.
Chitosan, a partially deacetylated derivative of chitin composed of N -acetylglucosamine, is structurally similar to glycosaminoglycan (GAG) and has been reported to be nontoxic and to promote wound healing . In addition, chitosan has also been shown to facilitate cell adhesion and proliferation and promote the activity of growth factors . The unique physical and chemical characteristics of chitosan, such as its cationic nature from primary amine groups and its pH-dependent solubility, enable chitosan to be fabricated into microspheres, membranes and scaffolds for pharmaceutical and tissue engineering uses . Porous chitosan-based scaffolds have been widely used and exhibited desirable results in tissue regeneration . Chitosan has provided good strength and toughness to scaffold materials , and supported mesenchymal stem cell function and osteogenic differentiation .
Therefore, chitosan scaffold might be useful in pulp capping to provide a matrix for the adhesion of endogenous odontoblast-like cells. Since chitosan itself is not sufficient to induce rapid dentinogenesis, it might be beneficial to combine TGF-β 1 with chitosan scaffold. Previous studies dipped the scaffolds into a growth factor-containing solution, or lyophilized the mixture of carrier material and growth factor to obtain a drug-loaded scaffold . However, the resulting materials could only provide a short-term and unsustained growth factor release. Since chitosan microspheres were used to provide controlled release of drugs and to improve the bioavailability of factors such as TGF-β 1 , the present study fabricated TGF-β 1 -containing chitosan microspheres and incorporated them into a bilayer chitosan membrane. One layer of the membrane consisted of a dense chitosan film, to cover the pulp from bacterial invasion. The other layer of chitosan was highly macroporous for TGF-β 1 -containing microspheres loading in order to obtain sustained release of TGF-β 1 .
Accordingly, the objectives of this study were to develop a chitosan membrane-microsphere construct with TGF-β 1 release for tissue engineering applications, to evaluate the effect of this construct on the proliferation of odontoblasts, and to investigate pulp-capping via this construct in a dog model to enhance dentinogenesis and reparative dentin formation for the first time. It was hypothesized that: (1) The chitosan membrane-microsphere construct will achieve a sustained TGF-β 1 release; (2) The membrane-microsphere with TGF-β 1 will enhance odontoblast proliferation in vitro; (3) The membrane-microsphere construct with TGF-β 1 will yield much more reparative dentin in vivo than chitosan membrane without TGF-β 1 , and than a commercial pulp-capping control.
2
Materials and methods
A chitosan bilayer membrane was synthesized that consisted of a dense film on one side and a macroporous sponge on the other side. The porous sponge layer was loaded with microspheres containing TGF-β 1 (MS-TGF) for sustained release. Odontoblast-like cells (MDPC-23) were cultured with MS-TGF-loaded membrane to evaluate its effect on the odontoblast proliferation in vitro. Pulp capping in teeth of beagle dogs was performed to test the effect of chitosan membrane with MS-TGF on reparative dentin formation.
2.1
Synthesis and characterization of chitosan microspheres
Chitosan (75–85% deacetylated) and surfactant Span-80 were purchased from the Sigma–Aldrich (St. Louis, MO). TGF-β 1 was obtained from Peprotech Inc. (Rocky Hill, NJ). Fetal bovine serum (FBS) and Dulbecco’s minimum Eagle’s medium (DMEM) were obtained from Gibco (Grand Island, NY). All solvents used were of analytical grade.
Chitosan microspheres were prepared using an emulsion-crosslinking method . Briefly, 100 mg of chitosan was dissolved in 4 mL of 2% acetic acid solution and centrifuged to remove any undissolved residue. The chitosan solution was dropped into 30 mL of liquid paraffin containing surfactant Span-80, and was emulsified at 1000 rpm for 5 min in a homogenizer (Mixer, Omni, Kennesaw, GA), resulting in the formation of water-in-oil emulsion. Next, 0.6 mL of glutaraldehyde was added into this emulsion as a crosslinking agent and agitated at 1000 rpm for 20 min in the homogenizer at 37 °C. After incubating for 30 min to complete the polymerization reaction, the precipitated microspheres were collected and washed with acetone and water, and then lyophilized by freeze-drying (Genesis Virtis, SP Scientific, Stone Ridge, NY).
For scanning electron microscopy (SEM), the dried chitosan microspheres were placed onto an electric-adhesive film and sputter-coated with gold. The size and morphology of the microspheres were examined with SEM (JSM-6460, JEOL, Japan).
Chitosan could absorb water and swell, and this property was used to load TGF-β 1 into the microspheres. The swelling ratio of chitosan microspheres was assessed. Two types of solutions were used to immerse the chitosan microspheres: A 0.01 M phosphate buffered saline (PBS) at pH 7.4 to simulate physiological fluid, and an acetic acid buffer at pH 5.2 to mimic an acidic environment due to inflammation and bioresorption by cells such as osteoclasts. Twenty milligrams of dry chitosan microspheres were placed into each solution at 37 °C. The suspensions were placed in a shaking bath at 135 rpm. At time intervals of 0.5, 1, 1.5, 2, 4, 6, 8, and 12 h, the suspensions were centrifuged and the weight of each batch of the swelled microspheres was measured for each time period. The swelling ratio = ( W t − W 0 )/ W 0 , where W 0 denotes the initial weight of the dry microspheres, and W t designates the weight of microspheres after water uptake at time t .
2.2
Incorporation of TGF-β 1 into chitosan microspheres
To incorporate TGF-β 1 into chitosan microspheres, 80 mg of dry microspheres were placed into 0.8 mL of acetic acid buffer solution containing 2 μg of TGF-β 1 . The suspension was placed in a shaking bath with a speed of 135 rpm at 4 °C for 6 h. The microspheres suspension were collected by centrifugation and then lyophilized. The supernatant from the suspension without microspheres was collected for TGF-β 1 concentration analysis using an enzyme-linked immunosorbent assay (ELISA). The total amount of TGF-β 1 placed into the suspension was W T . The amount of TGF-β 1 left in the supernatant was measured to be W S . Hence, the amount of TGF-β 1 incorporated into the microspheres was W T − W S . The TGF-β 1 entrapment efficiency (EE) in the microspheres was EE = ( W T − W S )/ W T . The TGF-β 1 drug loading capacity DL = ( W T − W S )/ W M , where W M is the weight of the microspheres. The TGF-β 1 loaded microspheres were referred to as MS-TGF.
2.3
TGF-β 1 release from chitosan microspheres
TGF-β 1 release was measured via a dynamic monitor method . Briefly, 20 mg of the TGF-β 1 loaded microspheres (MS-TGF) were placed in sterile tube containing 1 mL of PBS, and incubated at 37 °C in a shaking bath at 135 rpm for pre-determined time periods up to 7 d. At each time period, the microsphere suspension was centrifuged, and the supernatant without microspheres was collected for TGF-β 1 concentration analysis. The microspheres thus obtained were then resuspended in fresh PBS to measure further TGF-β 1 release for the next time period. The TGF-β 1 concentrations in the solutions were measured via the ELISA assay, and the cumulative TGF-β 1 released out of the microspheres was calculated.
2.4
Fabrication and characterization of chitosan bilayer membrane
A porous chitosan membrane scaffold was fabricated following previous studies . The membrane was a bilayer, consisting of a dense chitosan thin film bonded with a macroporous chitosan sponge layer. To make the membrane, a 2% chitosan solution was prepared in 2% aqueous acetic acid, and 1.5 mL of this solution was poured into a round mold with a flat bottom having a diameter of 9 cm. The solvent was evaporated in air at 37 °C, resulting in a chitosan film. The film was washed with 1% NaOH solution to remove residual acetic acid, followed by rinsing with water. Next, 3 mL of the chitosan solution was poured into the mold with the dry chitosan film at its bottom. The mold was immediately frozen at −20 °C for 2 h and lyophilized for 24 h in the freeze dryer. This resulted in the chitosan membrane, which consisted of a macroporous spongy layer on the top of a dense chitosan film. The purpose of the dense chitosan film was to cover the perforated pulp and block bacterial invasion. The purpose of the macroporous layer was to be subsequently impregnated with microspheres carrying TGF-β 1 . Both the dense film and the macroporous side of the chitosan membrane were examined with SEM. The cross-section of the membrane was also examined with SEM.
2.5
Incorporation of MS-TGF into chitosan membrane
The MS-TGF were incorporated into the macroporous layer of the chitosan membrane. The membrane was cut into disks with a diameter of 10 mm. The membrane thickness was 0.21 mm. The MS-TGF were dispersed in 100 μL of 90% aqueous ethanol, and then pipetted into the macroporous side of the membrane. Then, the membrane was immediately lyophilized. Although there was no specific study on the use of TGF-β 1 microsphere-incorporated scaffold for reparative dentin regeneration; previous studies reported that the TGF-β 1 microspheres were encapsulated into the scaffold at a concentration of 10 ng per 10-mm diameter scaffold for cartilage tissue engineering, and this controlled TGF-β 1 releasing system exhibited the potential to enhance cartilage formation . Therefore, in the present study, microspheres carrying 10 ng of TGF-β 1 were loaded into each 10-mm diameter membrane disk for dentin regeneration. This resulted in a TGF-β 1 concentration of 0.6 ng/mm 3 of scaffold. This TGF-β 1 concentration was within the range of previous studies, from 100 ng of TGF-β 1 in an implant with a volume of 100 mm 3 (concentration = 1 ng/mm 3 ) , to 20 ng in an implant with a volume of 50 mm 3 (concentration = 0.4 ng/mm 3 ) . The chitosan bilayer membrane containing MS-TGF was designated as “membrane + MS-TGF”.
2.6
In vitro odontoblast-like cell culture
Odontoblast-like cells MDPC-23 were cultured following a previous study . Culture was done in a medium of DMEM with 10% FBS, 100 IU/mL penicillin, 100 μg/mL streptomycin and 2 mM glutamine (Gibco) in a humidified atmosphere containing 5% CO 2 at 37 °C. After adjusting to 2 × 10 5 cells/mL, a cell suspension of 100 μL (containing 2 × 10 4 cells) was added into each chitosan membrane disk with a diameter of 10 mm. The constructs were placed into the wells of 24-well plates, and 1.5 mL of the culture medium was added to each well. The medium was changed every 2 d. At time periods of 1, 3, 5 and 7 d, five samples were used for cell counting to measure cell proliferation vs. time ( n = 5). Four groups were tested:
- (1)
cells were cultured on chitosan membrane with MS-TGF containing 10 ng of TGF-β 1 (membrane + MS-TGF);
- (2)
cells were cultured on chitosan membrane without MS-TGF (membrane, no TGF);
- (3)
cells were cultured on well surface with medium having 10 ng of TGF-β 1 addition, but without chitosan membrane (medium + TGF, no membrane); and
- (4)
cells were cultured on well surface in normal medium (no TGF, no membrane).
2.7
In vivo dog pulp model for reparative dentin formation
The animal study was approved by the ethical committee of the Fourth Military Medical University. A total of 48 teeth (4th pre-molar or 1st molar) of six healthy male beagle dogs (18–24 months old, weighing 8–10 kg) were used. The animals received anesthesia by intramuscular injection of 20 mg/kg ketamin HCl and 0.05 mg/kg acepromazine, following a previous study . Four groups were tested for direct pulp capping:
- (1)
control with no pulp-capping material;
- (2)
Dycal radiopaque calcium hydroxide control (Dentsply, Milford, DE);
- (3)
chitosan bilayer membrane without MS-TGF (membrane, no TGF); and
- (4)
chitosan bilayer membrane with MS-TGF (membrane + MS-TGF).
Two time intervals of 10 or 60 d were used for pulp response examination. The 4th pre-molar and 1st molar on the left maxillary and mandible were used for the 60-d evaluation. The 4th pre-molar and 1st molar on the right maxillary and mandible were used for the 10-d evaluation. Teeth on the left side of each dog (four teeth) were randomized into 4 groups. Six dogs were used for the study, hence six teeth were enrolled for each group ( n = 6). After local anesthesia with mepivacaine, the operating field was disinfected with 3% hydrogen peroxide, followed by 0.2% chlorhexidine. Class V cavities with an approximate circular shape having a diameter of 2 mm were prepared in the mid-crown areas of the buccal surfaces of the teeth using sterile fissure diamond (0.5 mm diameter, SS White) with a high-speed hand-piece. The cavity depth was limited by the appearance of pulpal reddish hue through the dentin. Standardized pulp exposures were made in the middle of the axial walls with a round diamond bur (0.5 mm diameter, SS White) under water spray. A new bur was used for each tooth. Bleeding was controlled with 0.9% saline solution irrigation and pressure of sterile cotton pellets soaked in saline solution on the exposures. The pulp exposures were then covered with the materials of each group as described above. For groups 3 and 4, the chitosan membrane was disk-shaped with a diameter of 2 mm. For group 4, using a concentration of 10 ng TGF-β 1 in a membrane of 10 mm diameter, each pulp with a membrane of 2 mm diameter received 0.4 ng of TGF-β 1 . Group 1 received no pulp-capping material. Then, all the cavities for all four groups were filled with a resin-modified glass ionomer cement (GC Fuji, Japan). A resin-modified glass ionomer was used to avoid the need for etching and bonding, to enable the investigation of the effect of chitosan membrane and TGF-β 1 on the exposed pulp, without interference from etchant and bonding agent. After fifty days, the teeth on the right side were prepared and restored according to protocol of each group as mentioned above.
After another 10 d, the dogs were sacrificed by an overdose of anesthetics (3% pentobarbital sodium), and venous infiltration perfusion was performed with 4% paraformaldehyde solution. The teeth and surrounding tissues were dissected from the jaws. A block section was prepared for each tooth and placed in an individual bottle containing 4% paraformaldehyde for 2 weeks. The roots were cut at mid-root to facilitate fixative penetration. Demineralization of the specimens was performed with 10% formic acid for 4 weeks. The specimens were rinsed with water, dehydrated in ascending concentration of ethanol and then embedded in paraffin. Sections with a thickness of 5 μm were cut in the bucco-lingual direction along the long axis of the tooth at every 50 μm through the exposure. The sections were stained with hematoxylin and eosin (H&E) for histomorphologic analysis. The sections were examined for pulp response and reparative dentin formation (Olympus IX83, Japan). For each tooth of the 60-d evaluation, the thickness of the reparative dentin formation was measured for all the sections throughout the exposure field using Adobe Photoshop software CS (Adobe Systems, San Jose, CA) . The average value was recorded as the reparative dentin thickness of the tooth. Hence, six thickness values for each group were obtained for statistical analysis (mean ± sd; n = 6).
One-way analysis of variance (ANOVA) was performed to detect the significant effects of the variables. Tukey’s multiple comparison was used to compare the data at a p value of 0.05.
2
Materials and methods
A chitosan bilayer membrane was synthesized that consisted of a dense film on one side and a macroporous sponge on the other side. The porous sponge layer was loaded with microspheres containing TGF-β 1 (MS-TGF) for sustained release. Odontoblast-like cells (MDPC-23) were cultured with MS-TGF-loaded membrane to evaluate its effect on the odontoblast proliferation in vitro. Pulp capping in teeth of beagle dogs was performed to test the effect of chitosan membrane with MS-TGF on reparative dentin formation.
2.1
Synthesis and characterization of chitosan microspheres
Chitosan (75–85% deacetylated) and surfactant Span-80 were purchased from the Sigma–Aldrich (St. Louis, MO). TGF-β 1 was obtained from Peprotech Inc. (Rocky Hill, NJ). Fetal bovine serum (FBS) and Dulbecco’s minimum Eagle’s medium (DMEM) were obtained from Gibco (Grand Island, NY). All solvents used were of analytical grade.
Chitosan microspheres were prepared using an emulsion-crosslinking method . Briefly, 100 mg of chitosan was dissolved in 4 mL of 2% acetic acid solution and centrifuged to remove any undissolved residue. The chitosan solution was dropped into 30 mL of liquid paraffin containing surfactant Span-80, and was emulsified at 1000 rpm for 5 min in a homogenizer (Mixer, Omni, Kennesaw, GA), resulting in the formation of water-in-oil emulsion. Next, 0.6 mL of glutaraldehyde was added into this emulsion as a crosslinking agent and agitated at 1000 rpm for 20 min in the homogenizer at 37 °C. After incubating for 30 min to complete the polymerization reaction, the precipitated microspheres were collected and washed with acetone and water, and then lyophilized by freeze-drying (Genesis Virtis, SP Scientific, Stone Ridge, NY).
For scanning electron microscopy (SEM), the dried chitosan microspheres were placed onto an electric-adhesive film and sputter-coated with gold. The size and morphology of the microspheres were examined with SEM (JSM-6460, JEOL, Japan).
Chitosan could absorb water and swell, and this property was used to load TGF-β 1 into the microspheres. The swelling ratio of chitosan microspheres was assessed. Two types of solutions were used to immerse the chitosan microspheres: A 0.01 M phosphate buffered saline (PBS) at pH 7.4 to simulate physiological fluid, and an acetic acid buffer at pH 5.2 to mimic an acidic environment due to inflammation and bioresorption by cells such as osteoclasts. Twenty milligrams of dry chitosan microspheres were placed into each solution at 37 °C. The suspensions were placed in a shaking bath at 135 rpm. At time intervals of 0.5, 1, 1.5, 2, 4, 6, 8, and 12 h, the suspensions were centrifuged and the weight of each batch of the swelled microspheres was measured for each time period. The swelling ratio = ( W t − W 0 )/ W 0 , where W 0 denotes the initial weight of the dry microspheres, and W t designates the weight of microspheres after water uptake at time t .
2.2
Incorporation of TGF-β 1 into chitosan microspheres
To incorporate TGF-β 1 into chitosan microspheres, 80 mg of dry microspheres were placed into 0.8 mL of acetic acid buffer solution containing 2 μg of TGF-β 1 . The suspension was placed in a shaking bath with a speed of 135 rpm at 4 °C for 6 h. The microspheres suspension were collected by centrifugation and then lyophilized. The supernatant from the suspension without microspheres was collected for TGF-β 1 concentration analysis using an enzyme-linked immunosorbent assay (ELISA). The total amount of TGF-β 1 placed into the suspension was W T . The amount of TGF-β 1 left in the supernatant was measured to be W S . Hence, the amount of TGF-β 1 incorporated into the microspheres was W T − W S . The TGF-β 1 entrapment efficiency (EE) in the microspheres was EE = ( W T − W S )/ W T . The TGF-β 1 drug loading capacity DL = ( W T − W S )/ W M , where W M is the weight of the microspheres. The TGF-β 1 loaded microspheres were referred to as MS-TGF.
2.3
TGF-β 1 release from chitosan microspheres
TGF-β 1 release was measured via a dynamic monitor method . Briefly, 20 mg of the TGF-β 1 loaded microspheres (MS-TGF) were placed in sterile tube containing 1 mL of PBS, and incubated at 37 °C in a shaking bath at 135 rpm for pre-determined time periods up to 7 d. At each time period, the microsphere suspension was centrifuged, and the supernatant without microspheres was collected for TGF-β 1 concentration analysis. The microspheres thus obtained were then resuspended in fresh PBS to measure further TGF-β 1 release for the next time period. The TGF-β 1 concentrations in the solutions were measured via the ELISA assay, and the cumulative TGF-β 1 released out of the microspheres was calculated.
2.4
Fabrication and characterization of chitosan bilayer membrane
A porous chitosan membrane scaffold was fabricated following previous studies . The membrane was a bilayer, consisting of a dense chitosan thin film bonded with a macroporous chitosan sponge layer. To make the membrane, a 2% chitosan solution was prepared in 2% aqueous acetic acid, and 1.5 mL of this solution was poured into a round mold with a flat bottom having a diameter of 9 cm. The solvent was evaporated in air at 37 °C, resulting in a chitosan film. The film was washed with 1% NaOH solution to remove residual acetic acid, followed by rinsing with water. Next, 3 mL of the chitosan solution was poured into the mold with the dry chitosan film at its bottom. The mold was immediately frozen at −20 °C for 2 h and lyophilized for 24 h in the freeze dryer. This resulted in the chitosan membrane, which consisted of a macroporous spongy layer on the top of a dense chitosan film. The purpose of the dense chitosan film was to cover the perforated pulp and block bacterial invasion. The purpose of the macroporous layer was to be subsequently impregnated with microspheres carrying TGF-β 1 . Both the dense film and the macroporous side of the chitosan membrane were examined with SEM. The cross-section of the membrane was also examined with SEM.
2.5
Incorporation of MS-TGF into chitosan membrane
The MS-TGF were incorporated into the macroporous layer of the chitosan membrane. The membrane was cut into disks with a diameter of 10 mm. The membrane thickness was 0.21 mm. The MS-TGF were dispersed in 100 μL of 90% aqueous ethanol, and then pipetted into the macroporous side of the membrane. Then, the membrane was immediately lyophilized. Although there was no specific study on the use of TGF-β 1 microsphere-incorporated scaffold for reparative dentin regeneration; previous studies reported that the TGF-β 1 microspheres were encapsulated into the scaffold at a concentration of 10 ng per 10-mm diameter scaffold for cartilage tissue engineering, and this controlled TGF-β 1 releasing system exhibited the potential to enhance cartilage formation . Therefore, in the present study, microspheres carrying 10 ng of TGF-β 1 were loaded into each 10-mm diameter membrane disk for dentin regeneration. This resulted in a TGF-β 1 concentration of 0.6 ng/mm 3 of scaffold. This TGF-β 1 concentration was within the range of previous studies, from 100 ng of TGF-β 1 in an implant with a volume of 100 mm 3 (concentration = 1 ng/mm 3 ) , to 20 ng in an implant with a volume of 50 mm 3 (concentration = 0.4 ng/mm 3 ) . The chitosan bilayer membrane containing MS-TGF was designated as “membrane + MS-TGF”.
2.6
In vitro odontoblast-like cell culture
Odontoblast-like cells MDPC-23 were cultured following a previous study . Culture was done in a medium of DMEM with 10% FBS, 100 IU/mL penicillin, 100 μg/mL streptomycin and 2 mM glutamine (Gibco) in a humidified atmosphere containing 5% CO 2 at 37 °C. After adjusting to 2 × 10 5 cells/mL, a cell suspension of 100 μL (containing 2 × 10 4 cells) was added into each chitosan membrane disk with a diameter of 10 mm. The constructs were placed into the wells of 24-well plates, and 1.5 mL of the culture medium was added to each well. The medium was changed every 2 d. At time periods of 1, 3, 5 and 7 d, five samples were used for cell counting to measure cell proliferation vs. time ( n = 5). Four groups were tested:
- (1)
cells were cultured on chitosan membrane with MS-TGF containing 10 ng of TGF-β 1 (membrane + MS-TGF);
- (2)
cells were cultured on chitosan membrane without MS-TGF (membrane, no TGF);
- (3)
cells were cultured on well surface with medium having 10 ng of TGF-β 1 addition, but without chitosan membrane (medium + TGF, no membrane); and
- (4)
cells were cultured on well surface in normal medium (no TGF, no membrane).
2.7
In vivo dog pulp model for reparative dentin formation
The animal study was approved by the ethical committee of the Fourth Military Medical University. A total of 48 teeth (4th pre-molar or 1st molar) of six healthy male beagle dogs (18–24 months old, weighing 8–10 kg) were used. The animals received anesthesia by intramuscular injection of 20 mg/kg ketamin HCl and 0.05 mg/kg acepromazine, following a previous study . Four groups were tested for direct pulp capping:
- (1)
control with no pulp-capping material;
- (2)
Dycal radiopaque calcium hydroxide control (Dentsply, Milford, DE);
- (3)
chitosan bilayer membrane without MS-TGF (membrane, no TGF); and
- (4)
chitosan bilayer membrane with MS-TGF (membrane + MS-TGF).
Two time intervals of 10 or 60 d were used for pulp response examination. The 4th pre-molar and 1st molar on the left maxillary and mandible were used for the 60-d evaluation. The 4th pre-molar and 1st molar on the right maxillary and mandible were used for the 10-d evaluation. Teeth on the left side of each dog (four teeth) were randomized into 4 groups. Six dogs were used for the study, hence six teeth were enrolled for each group ( n = 6). After local anesthesia with mepivacaine, the operating field was disinfected with 3% hydrogen peroxide, followed by 0.2% chlorhexidine. Class V cavities with an approximate circular shape having a diameter of 2 mm were prepared in the mid-crown areas of the buccal surfaces of the teeth using sterile fissure diamond (0.5 mm diameter, SS White) with a high-speed hand-piece. The cavity depth was limited by the appearance of pulpal reddish hue through the dentin. Standardized pulp exposures were made in the middle of the axial walls with a round diamond bur (0.5 mm diameter, SS White) under water spray. A new bur was used for each tooth. Bleeding was controlled with 0.9% saline solution irrigation and pressure of sterile cotton pellets soaked in saline solution on the exposures. The pulp exposures were then covered with the materials of each group as described above. For groups 3 and 4, the chitosan membrane was disk-shaped with a diameter of 2 mm. For group 4, using a concentration of 10 ng TGF-β 1 in a membrane of 10 mm diameter, each pulp with a membrane of 2 mm diameter received 0.4 ng of TGF-β 1 . Group 1 received no pulp-capping material. Then, all the cavities for all four groups were filled with a resin-modified glass ionomer cement (GC Fuji, Japan). A resin-modified glass ionomer was used to avoid the need for etching and bonding, to enable the investigation of the effect of chitosan membrane and TGF-β 1 on the exposed pulp, without interference from etchant and bonding agent. After fifty days, the teeth on the right side were prepared and restored according to protocol of each group as mentioned above.
After another 10 d, the dogs were sacrificed by an overdose of anesthetics (3% pentobarbital sodium), and venous infiltration perfusion was performed with 4% paraformaldehyde solution. The teeth and surrounding tissues were dissected from the jaws. A block section was prepared for each tooth and placed in an individual bottle containing 4% paraformaldehyde for 2 weeks. The roots were cut at mid-root to facilitate fixative penetration. Demineralization of the specimens was performed with 10% formic acid for 4 weeks. The specimens were rinsed with water, dehydrated in ascending concentration of ethanol and then embedded in paraffin. Sections with a thickness of 5 μm were cut in the bucco-lingual direction along the long axis of the tooth at every 50 μm through the exposure. The sections were stained with hematoxylin and eosin (H&E) for histomorphologic analysis. The sections were examined for pulp response and reparative dentin formation (Olympus IX83, Japan). For each tooth of the 60-d evaluation, the thickness of the reparative dentin formation was measured for all the sections throughout the exposure field using Adobe Photoshop software CS (Adobe Systems, San Jose, CA) . The average value was recorded as the reparative dentin thickness of the tooth. Hence, six thickness values for each group were obtained for statistical analysis (mean ± sd; n = 6).
One-way analysis of variance (ANOVA) was performed to detect the significant effects of the variables. Tukey’s multiple comparison was used to compare the data at a p value of 0.05.
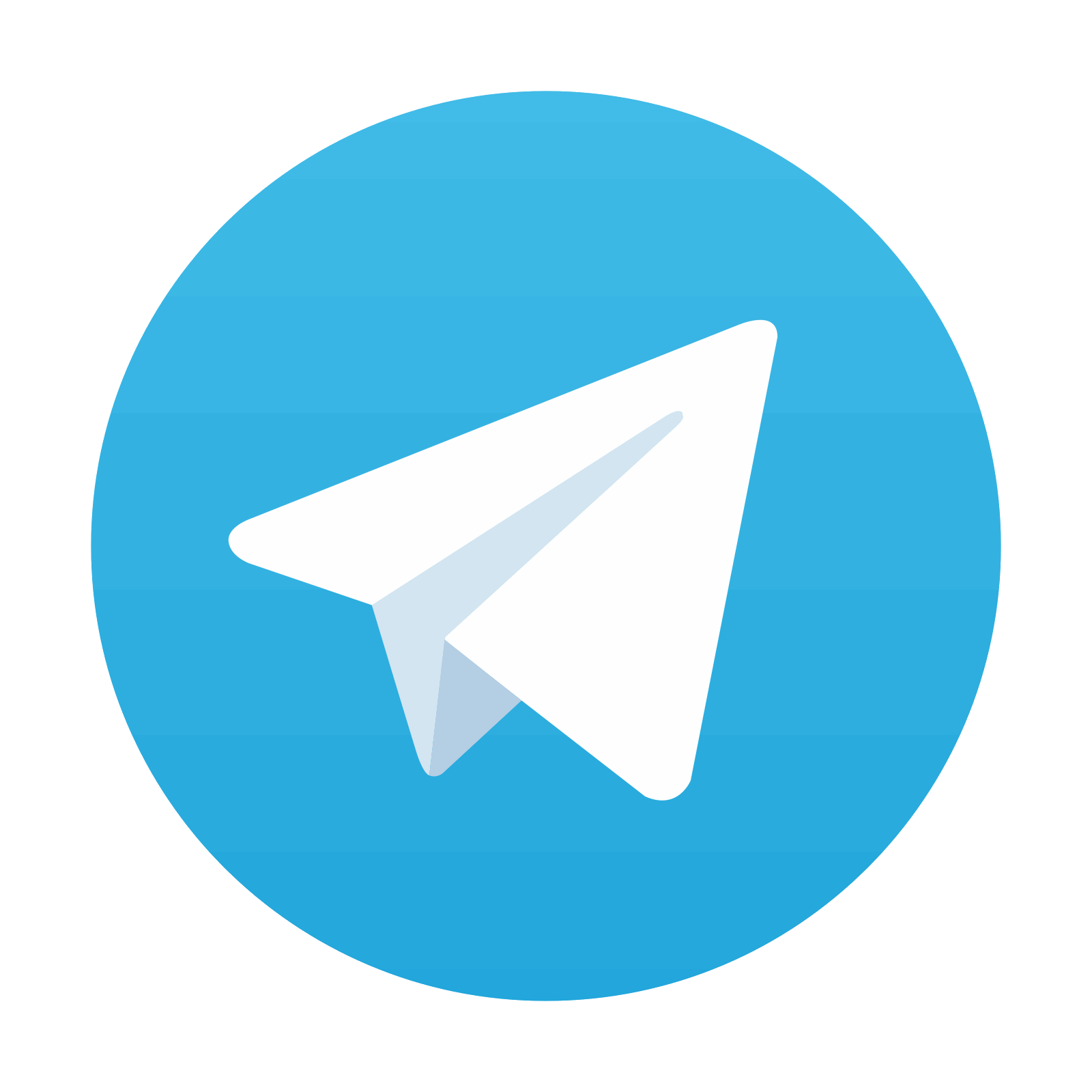
Stay updated, free dental videos. Join our Telegram channel

VIDEdental - Online dental courses
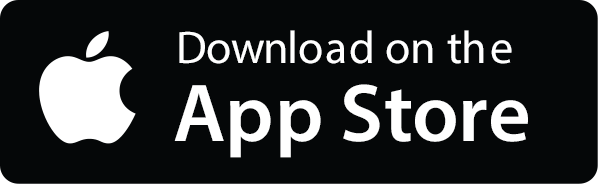
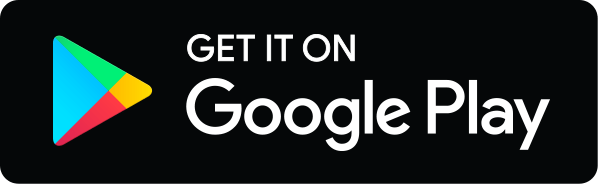