Abstract
Objective
To use a compliance-variable instrument to simultaneously measure and compare the polymerization stress (PS) evolution, degree of conversion (DC), and exotherm of a bulk-fill flowable composite to a packable composite.
Methods
A bulk-fill flowable composite (Filtek Bulk-fill, FBF) and a conventional packable composite (Filtek Z250, Z250) purchased from 3 M ESPE were investigated. The composites were studied using a cantilever-beam based instrument equipped with an in situ near infrared (NIR) spectrometer and a microprobe thermocouple. The measurements were carried out under various instrumental compliances (ranging from 0.3327 μm/N to 12.3215 μm/N) that are comparable to the compliances of clinically prepared tooth cavities. Correlations between the PS and temperature change as well as the DC were interpreted.
Results
The maximum PS of both composites at 10 min after irradiation decreased with the increase in the compliance of the cantilever beam. The FBF composite generated a lower final stress than the Z250 sample under instrumental compliances less than ca. 4 μm/N; however, both materials generated statistically similar PS values at higher compliances. The reaction exotherm and the DC of both materials were found to be independent of compliance. The DC of the FBF sample was slightly higher than that of the packable Z250 composite while the peak exotherm of FBF was almost double that of the Z250 composite. For FBF, a characteristic drop in the PS was observed during the early stage of polymerization for all compliances studied which was not observed in the Z250 sample. This drop was shown to relate to the greater exotherm of the less-filled FBF sample relative to the Z250 composite.
Significance
While the composites with lower filler content (low viscosity) are generally considered to have lower PS than the conventional packable composites, a bulk-fill flowable composite was shown to produce lower PS under a lower compliance of constraint as would be experienced if the composite was used as the base material in clinical procedures.
1
Introduction
Extensive efforts have been employed over the years to improve the physical and mechanical properties of photopolymerized dental composites that are used to restore the appearance and function of teeth . As monomers used in dental composite resins react to form covalent bonds during the polymerization process, the volume of the resulting polymer contracts. Subsequently, enormous polymerization stresses (PS) can develop due to external constrains (i.e., tooth walls or substrates) during the process . This stress is considered undesirable and could lead to deleterious clinical signs and symptoms, such as post-operative sensitivity, debonding, marginal discoloration, secondary caries, and cusp fractures . Incorporating a larger amount of filler, increasing the molar mass or polarity of monomers, and tuning the reaction kinetics are some of the strategies used to mitigate the adverse effects of polymerization shrinkage. Conventionally, the restorative resins are incorporated with a high content (ca. 80% by mass) of silica filler. The presence of filler in these composite resins, known as “packable composites”, reduces the overall shrinkage relative to an unfilled resin. In addition, these composites have an increased modulus due to the presence of the filler which improves the hardness and performance life time of the composite in the tooth cavity. However, PS is primarily determined by the reciprocal relationship between the shrinkage and modulus. Recently, with modifications in the filler content and/or the organic matrix, a new generation of composites known as “bulk-fill flowable composites” has been introduced which claim to exhibit low PS as one of the main advancements . The lower filler content of the bulk-fill flowable composites (ca. 60 mass%) results in a lower modulus and is considered to be the main contributing factor to the reduced PS . It has been recommended that most of the bulk-fill flowable materials be used as base materials in dental cavities, which are then veneered with 2 mm of the higher filler content packable composites with relatively better mechanical properties such as hardness, stiffness and strength . Besides showing the reciprocal relationship between the shrinkage and modulus, many studies also indicate that the effect of the filler content on PS can be significantly influenced by the compliance of the testing systems used to measure the PS . Clinically, the compliance is characterized by the geometry of the prepared tooth cavity and the stiffness (or compliance) of the remaining tooth structure . Thus, it remains elusive whether bulk-fill flowable materials always generate relatively lower PS under varied compliances, or whether the flowable composites are suitable as a base material in the dental restoration. The main goal of this study is to compare the evolution in the PS of a bulk-fill flowable composite under different compliances to a conventional packable composite during polymerization.
Numerous studies have investigated the effect of filler content on PS with different testing systems with conflicting results. This could be attributed to the fact that these studies did not take into account the compliance of the testing system as a systematic variable. Condon et al. evaluated the magnitude of the PS of a variety of commercial composites containing different levels of filler content using a mechanical testing instrument with very low compliance and concluded that the PS increased with increasing filler content . Boaro et al. reported the same trend of PS in relation to filler content by investigating seventeen commercial dental composites using a stress-strain analyzer with a feedback system, which allows for a near zero compliance . Braga et al. found an inverse trend when evaluating the PS of eight, and then twenty in their later study, experimental composites loaded with incremental filler content in a universal test machine using an acrylic as a bonding substrate. The presence of the acrylic material resulted in an increase in the compliance of the testing system relative to the previously mentioned studies where glass was usually adopted as the bonding substrate. Stansbury et al. measured the PS of five composites with incremental filler content using an instrument with relatively low compliance . They reported no significant statistical difference in the PS due to the filler content. Nevertheless, these studies of PS development as a function of filler content were performed under testing systems with a fixed compliance that might not be clinically relevant; no systematic variation of compliance has been incorporated in their studies. Recently, Fok et al. developed a mathematical model to predict the development of PS and reported that differences in the compliance of the experimental systems would significantly influence the effect of the filler content on the PS. This study showed that with increasing filler content, the PS could increase or stay constant depending on the compliance of the testing system . Recently, a work was conducted in our lab using experimental and analytical approaches to systematically study the effect of filler content on PS using model composites with incremental filler contents (0–75% by mass). This study demonstrated that the PS actually increases, decreases, or remains constant with increasing filler content depending upon the instrumental compliance . This work also emphasized the importance of the testing systems compliance in obtaining unbiased or misleading test results in PS evaluation.
Another important aspect of the polymerization of dental materials is the degree of conversion (DC) of the methacrylate monomers to polymer. This conversion is correlated with the volumetric shrinkage and modulus development in the polymerization process. It has been shown that the degree of conversion of the methacrylate monomer progressively decreases with increasing filler content ; an inverse relationship was also found between the filler content and the magnitude of the polymerization exotherm (i.e., less filled flowable composites would show higher DC and exotherm than conventional composites ). The exotherm would affect not only the pulp vitality during dental treatment, but also the polymerization process . Therefore, measurements of DC and exotherm are indispensable for better interpretation of the PS evolution during polymerization process.
In the present study, real-time and simultaneous measurements of the PS, DC and exotherm were performed on commercially available bulk-fill flowable and packable composites using a NIST-developed cantilever-beam based instrument . The key advantage for the simultaneous measurements is that these properties can be correlated directly, thus providing higher measurement confidence and a more in-depth understanding of the network formation process. Unlike other stress measurement instruments with a fixed compliance (high or low), the instrument used in this study readily allows for a variety of compliances to be employed. A systematic variation of instrument compliance comparable to possible clinical compliances of tooth cavities was obtained by changing the sample position along the cantilever beam. The instrument was coupled to a fast near infrared (NIR) spectrometer collecting spectra in transmission mode to measure the DC during the polymerization process. The polymerization exotherm was determined with a microprobe thermocouple embedded into the center of the sample. Our results indicated that, depending on the compliance of testing system, the PS generated from the bulk-fill flowable composite is not always lower than that from the higher filler content packable composite as is usually expected. Nevertheless, the bulk-fill flowable composite develops less PS than packable composite under the lower compliances studied. This validates the use of bulk-fill flowable composite as a base material in clinical procedures. The bulk-fill flowable composite shows a higher exotherm and DC than the packable composites but neither the exotherm nor DC are dependent on the compliance of the instrument. It is expected that the significantly higher exotherm induces a greater thermal expansion of the bulk-fill flowable material which contributes to the reduced final PS under low compliances.
2
Materials and methods 1
1 Certain commercial materials and equipment are identified in this manuscript in order to specify adequately the experimental and analysis procedures. In no case does such identification imply recommendation or endorsement by the National Institute of Standards and Technology (NIST) nor does it imply that they are necessarily the best available for the purpose.
Two composite materials from 3 M ESPE (St. Paul, MN, USA) were investigated ( Table 1 ). One composite was the bulk-fill flowable composite, Filtek Bulk Fill (FBF). This FBF composite has a reduced filler content which lowers its viscosity and allows free flow into the cavity. It is meant to be used in a single application to completely fill the cavity followed by photo curing; however, a veneer cap of a higher modulus composite is often required. The second composite was the traditional universal methacrylate-based composite, Filtek Z250 (Z250) with a higher filler content and consequently a higher viscosity. It is typically used in a conventional filling application where 2 mm incremental layers of the material are applied and cured to fill the entire cavity. All experiments were conducted under a yellow light environment to minimize premature photopolymerization at an initial temperature of 22 ± 1 °C.
Material | Code | Type | Shade | Resin composition | Filler composition | Filler content | Filler size |
---|---|---|---|---|---|---|---|
Filtek Bulk Fill | FBF | Flowable bulk-fill | Universal | Bis-GMA (1–10%), UDMA (10–20%), Bis-EMA (1–10%), Procrylat resins (10–20%) | Zirconia/silica, Ytterbium trifluoride | 64.5 wt% | 0.01–3.5 μm (Zirconia/silica), 0.1–5.0 μm (Ytterbium trifluoride) |
Filtek Z250 | Z250 | Packable composite | A3 | Bis-GMA (1–10%), UDMA (1–10%), Bis-EMA (1–10%) | Zirconia/silica | 82 wt% | 0.01–3.5 μm |
In this study, a NIST-developed cantilever-beam based instrument ( Fig. 1 ) was coupled to a high-speed data acquisition NIR spectrometer and a microprobe thermocouple to enable real-time simultaneous measurement of polymerization stress (PS), degree of conversion (DC), and exotherm during the polymerization . The instrument was constructed according to design criteria following first principles of mechanics to provide the accuracy and sensitivity of the PS measurement . The desired compliance of the testing instrument can be easily achieved by varying the sample position along the cantilever beam. In order to generate PS values that most closely reflect a clinical situation, a rigid steel beam (modulus: 203 GPa; width: 6.32 mm; height: 12.65 mm) that provides compliances ranging from 0.3327 μm/N to 12.3215 μm/N was selected. A capacitive displacement sensor with 20 nm resolution (Driver Model: CPL190, Probe Model: C18-13, Lion Precision, St. Paul, MN) was used to detect the beam deflection for calculating the PS development. The analog data detected by the sensor were collected using the LabView software program (National Instruments, Austin, TX) via a 24-bit DAQ device (USB-2404-10, Measurement Computing, Norton, MA). For both composites, at least three replicates were tested at each position on the beam. Detailed experimental procedures can be obtained from previous publications . The size of the specimen disk was 2.5 mm in diameter and 2 mm in height. A QHL 75™ curing lamp (Dentsply-Caulk, Milford, DE) was used to provide the irradiance intensity of 500 ± 10 mW/cm 2 , measured with a Cure Rite radiometer (model #8000, EFOS Inc., Williamsville, NY). The irradiation starting and ending times were controlled by the LabView program through a controllable power outlet, such that the data collection and the photoactivation were synchronized. Upon photopolymerization, the composite shrinkage induced a deflection in the cantilever beam, which was recorded by the displacement sensor at the free end of the beam. Using this displacement and the known beam compliance, the PS was then calculated using a beam formula . For all the samples, the DC was measured using the coupled NIR spectrometer by assessing the variation in the ratio of the absorbance intensities of the methacrylate functional group C C-H peak centered at 1638 nm. The real-time DC was calculated for each sample by taking the peak area of the uncured sample prior to irradiation (Area uncured ) and at each time point during the polymerization after irradiation (Area cured ) using the following formula: DC = 1 − Area cured /Area uncured . The temperature change during polymerization was also monitored ( Fig. 1 b) using a 100 μm microprobe thermometer (Type T Quick-response PFA-insulated thermocouple probe from Cole-Palmer Instrument Company, Vernon Hills, IL) inserted into the center of the sample. The PS, DC and temperature change (exotherm) were simultaneously recorded at an interval of 0.15 s for 10 min using the LabView software. A Student’s t -test was performed to identify the difference between the two composites at a significance level of p < 0.05 using statistical software (SPSS 17, IBM Inc., USA).

2
Materials and methods 1
1 Certain commercial materials and equipment are identified in this manuscript in order to specify adequately the experimental and analysis procedures. In no case does such identification imply recommendation or endorsement by the National Institute of Standards and Technology (NIST) nor does it imply that they are necessarily the best available for the purpose.
Two composite materials from 3 M ESPE (St. Paul, MN, USA) were investigated ( Table 1 ). One composite was the bulk-fill flowable composite, Filtek Bulk Fill (FBF). This FBF composite has a reduced filler content which lowers its viscosity and allows free flow into the cavity. It is meant to be used in a single application to completely fill the cavity followed by photo curing; however, a veneer cap of a higher modulus composite is often required. The second composite was the traditional universal methacrylate-based composite, Filtek Z250 (Z250) with a higher filler content and consequently a higher viscosity. It is typically used in a conventional filling application where 2 mm incremental layers of the material are applied and cured to fill the entire cavity. All experiments were conducted under a yellow light environment to minimize premature photopolymerization at an initial temperature of 22 ± 1 °C.
Material | Code | Type | Shade | Resin composition | Filler composition | Filler content | Filler size |
---|---|---|---|---|---|---|---|
Filtek Bulk Fill | FBF | Flowable bulk-fill | Universal | Bis-GMA (1–10%), UDMA (10–20%), Bis-EMA (1–10%), Procrylat resins (10–20%) | Zirconia/silica, Ytterbium trifluoride | 64.5 wt% | 0.01–3.5 μm (Zirconia/silica), 0.1–5.0 μm (Ytterbium trifluoride) |
Filtek Z250 | Z250 | Packable composite | A3 | Bis-GMA (1–10%), UDMA (1–10%), Bis-EMA (1–10%) | Zirconia/silica | 82 wt% | 0.01–3.5 μm |
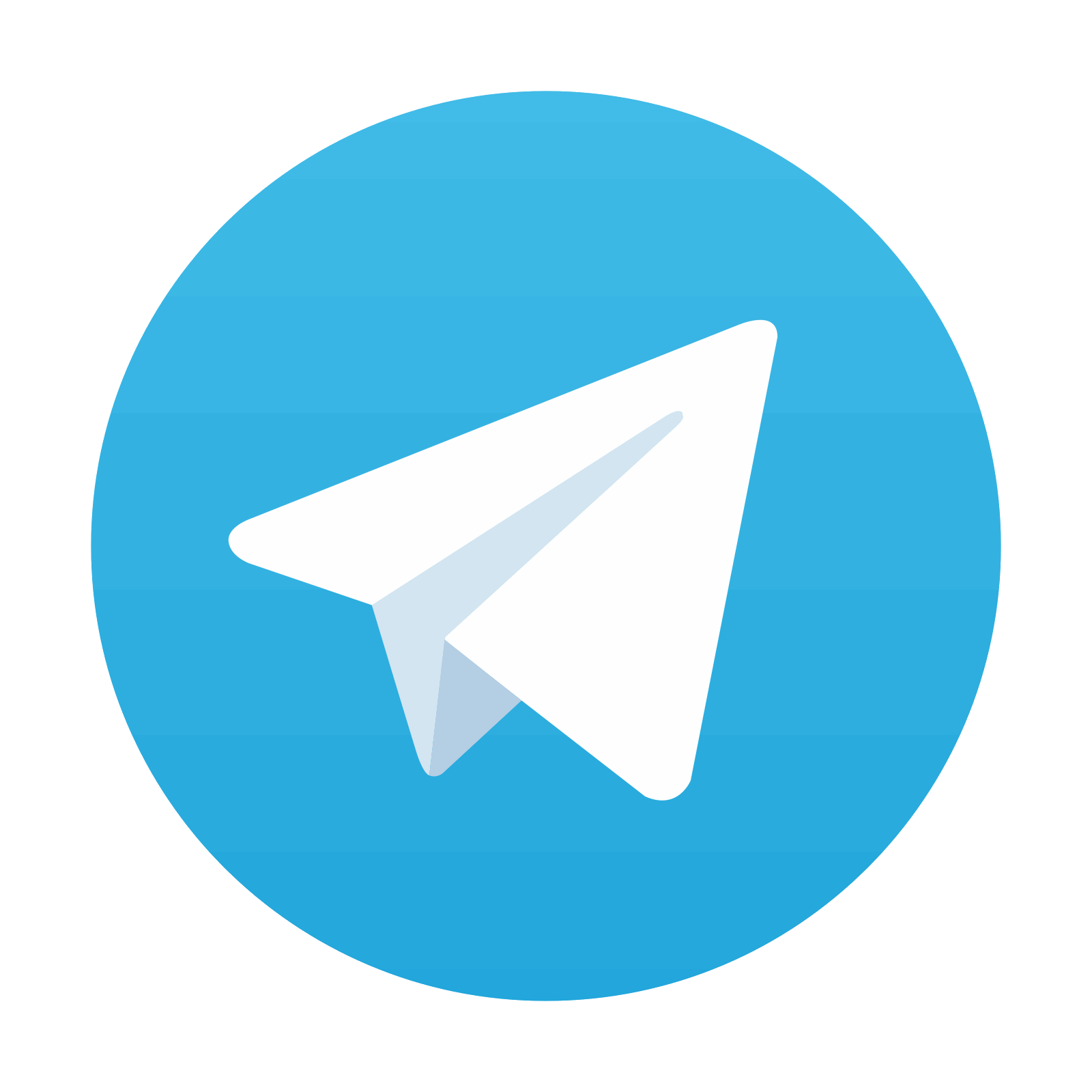
Stay updated, free dental videos. Join our Telegram channel

VIDEdental - Online dental courses
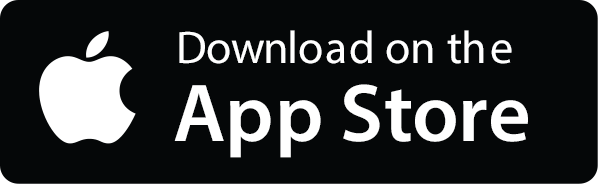
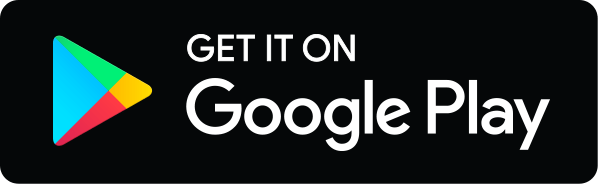
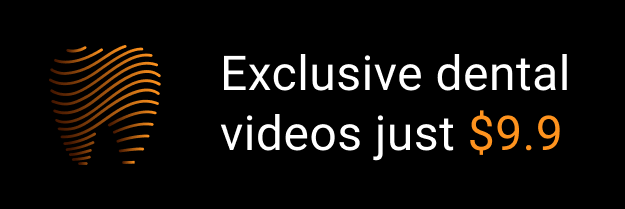