Abstract
Objectives
To assess depth of cure (DOC), degree of conversion (DC), and flexural strength (FS) of several resin composites with low-stress behavior.
Methods
SonicFill (Kerr), SureFil ® SDR™ (Dentsply), everX Posterior (GC), Kalore (GC), and Filtek Silorane (3M ESPE) were tested. DOC was measured with the Acetone Shake test. DC was assessed with Fourier Transform Infra-Red spectroscopy on top and at the bottom of 4 mm-thick disk-shaped specimens. Bottom to top ratios of DC percentages were calculated. FS was evaluated with the Three-Point Bending test. DOC, DC, and FS data were statistically analyzed.
Results
SureFil ® SDR™ and everX Posterior achieved significantly greater DOC than Kalore and Filtek Silorane. Also, SonicFill had significantly greater DOC than Filtek Silorane. Mean top DCs ranged between 71.46% and 52.44%. Kalore and everX Posterior had significantly lower top DCs than the other materials. Mean DC values at 4 mm ranged largely from 57.95% to 6.82%. Kalore and Filtek Silorane had the lowest values of bottom DC and the difference was statistically significant. EverX Posterior and SonicFill recorded significantly higher FSs than the other materials.
Significance
SureFil ® SDR™ and everX Posterior exhibited DOC over 4 mm, the maximum thickness recommended for bulk placement, while SonicFill recorded DOC values very close to the 4 mm threshold. SonicFill achieved the highest DC at the irradiated surface, as well as at 4 mm depth. SureFil ® SDR™ demonstrated similarly uniform curing through the bulk increment. All the tested composites complied with the requirements of FS established by ISO 4049/2009.
1
Introduction
Polymerization shrinkage stress of resin-based composites can affect marginal integrity and lead to marginal leakage, debonding, secondary caries, post-operative sensitivity, development of perimarginal white lines . Curing stress can also be responsible for cusp deflection in high C-factor direct composite restorations, such as large Class I and mesio-occluso-distal Class II cavities . Since all the mentioned conditions adverse the durability of resin-based restorations, research has constantly aimed at the development of materials with low-stress behavior and recently pursued the introduction of novel composites for ‘bulk’ application. Owing to innovations in monomer chemistry, filler characteristics or polymerization kinetics, such materials provide low curing shrinkage that may enable the omission of incremental layering, thus easing the restorative procedure and saving chair time . However, for effective bulk-filling of large and deep cavities, other characteristics of the restorative composites are desirable beside low shrinkage. Particularly, optical properties and photoiniating system should ensure adequate depth of cure to the bulk-applied increment. Several recently marketed ‘bulk-fill’ materials have been claimed to achieve adequate polymerization through a depth of over 4 mm .
In order to assess the maximal increment thickness of resin composites, researchers have referred to depth of cure (DOC) measurements recorded according to ISO 4049:2000 and ISO 4049:2009 . Polymerization efficiency of resin composites has also been assessed by measuring the degree of conversion (DC) with spectroscopic techniques that infer the amount of remaining double bonds . Current literature provides DOC and DC data for several materials for bulk filling . However, in most of the published studies DC was not measured at the clinically relevant depth for bulk-fill composites of 4 mm . Moreover, DOC was assessed as per ISO 4049 with the ‘Scrape test’ , yet the suitability of this method for bulk-fill composites has recently been criticized for providing an overestimation of curing depth in comparison with Vickers hardness profiles . In general, the procedure of scraping off the uncured resin-based material has been considered difficult to standardize , and the Acetone Shake test, a method involving physical removal of the unreacted monomers, has been preferred by some researchers .
Another clinically relevant feature of a bulk-fill composite is the ability to function as a ‘dentin-replacement’ material. Such potential can be estimated in laboratory research through the assessment of mechanical properties. Mechanical properties can be expected to vary quite largely among the available bulk-fill composites, in relation to differences in filler load and characteristics. Some products present flowable consistency to enable self-adaptation to cavity walls , while other materials have higher filler load , or feature short glass fibers for reinforcement . Among the macromechanical properties that can be tested in laboratory, flexural strength provides an estimate of the composite resin potential to serve as dentin-replacement in high stress bearing areas.
The present study was conducted to assess DOC with the Acetone Shake test and DC at a clinically relevant depth for several resin composites with low-stress behavior. A further objective of the investigation was to measure the flexural strength (FS) attained by the same materials. The null hypotheses that the materials achieve similarly efficient cure and comparable FS were placed under test.
2
Materials and methods
The following resin composites for bulk-filling of posterior restorations were tested: SonicFill (Kerr, Orange, CA, USA), SureFil ® SDR™ (Dentsply, Milford, DE, USA), everX Posterior (GC, Tokyo, Japan). Additionally, the nanohybrid composite Kalore (GC, Tokyo, Japan) and the silorane-based composite Filtek Silorane (3M ESPE, St. Paul, MN, USA), exhibiting low-stress behavior, although not specifically marketed for bulk placement , were included in the study in order to verify their applicability in this simplified filling technique. The chemical composition of the materials is reported in Table 1 .
Material | Chemical composition |
---|---|
Filtek Silorane (3M ESPE, St. Paul, MN, USA) | Silorane resin; quartz filler; yttrium fluoride; initiating system: camphorquinone, iodonium salt, electron donor; stabilizers; pigments. |
Filler load 76 wt%; 55 vol% | |
Kalore (GC Corp., Tokyo, Japan) | Urethane dimethacrylate, DX-511 co-monomers, dimethacrylate (18 wt%); pre-polymerized filler (20–30 wt%); fluoroaluminosilicate glass (20–33 wt%); strontium/barium glass (20–33 wt%); silicon dioxide nanofiller (1–5 wt%); camphorquinone (<1 wt%), pigment (<1 wt%). |
Filler load 82 wt%; 69 vol% | |
SonicFill (Kerr Corp., Orange, CA, USA) | Glass, oxide, chemicals (10–30%); 3-trimethoxysilylpropyl methacrylate (10–30%); silicon dioxide (5–10%); ethoxylated bisphenol-A-dimethacrylate (1–5%); bisphenol-A-bis-(2-hydroxy-3-mehacryloxypropyl) ether (1–5%); triethylene glycol dimethacrylate (1–5%). |
Filler load 83.5 wt%; 83 vol% | |
SureFil ® SDR™ (Dentsply De Trey, Konstanz, Germany) | SDR™ patented urethane dimethacrylate resin, ethoxylated bisphenol A dimethacrylate, triethylene glycol dimethacrylate, butylated hydroxyl toluene, barium-alumino-fluoro-borosilicate glass, strontium alumino-fluoro-silicate glass, camphorquinone, UV stabilizer, titanium dioxide, iron oxide pigments. |
Filler load 68 wt%; 44%vol% | |
everX Posterior (GC, Tokyo, Japan) | Bisphenol A-diglycidyl dimethacrylate, triethylene glycol dimethacrylate (24.4 wt%); polymethyl methacrylate (0.9 wt%); E-glass fibers, barium borosilicate glass filler (74.2 wt%); camphorquinone, 2-dimethylamino ethyl dimethacrylate, hydroquinone (0.5 wt%). |
Filler load 74.2 wt%; 53.6 vol% |
For the materials available in more shades, in accordance with ISO 4049/2009, only shade A3 was used, in order to minimize the effects of shade on light polymerization .
2.1
Depth of cure (DOC)
DOC of the resin composite was assessed with the Acetone Shake test . Each material was placed in bulk in a cylindrical silicone mold (height 12 mm, internal diameter 5 mm). A mylar strip was then pressed over the applied material with a glass plate to obtain a flat surface. Following manufacturers’ recommendations, light-curing was performed for 20 s using Demi LED light (Orange, CA, USA; output 1100 mW/cm 2 ; tip diameter 8 mm), placing the light tip on the exposed side of the specimen. After curing and removal from the mold, the resin composite cylinder was placed into a hermetically sealed capsule containing 99.9 per cent pure acetone (2004/000113, Polichimica srl, Bologna, Italy). The capsule was vibrated on a mixing device for 15 s (TAC-135/A n°3437, Tac Dental, Asti, Italy) . Such vibrating motion removed the uncured material in a reproducible manner , leaving the polymerized portion undamaged. At this stage, the resin composite specimen was removed from the container and dried. The height of the specimen was measured in mm using a digital caliper with a 10 μm resolution (Mitutoyo, Miyazaki, Japan) and divided by two, as defined for the ‘Scrape test’ in ISO 4049. The rationale for such subdivision is that not all the hardened specimen is in fact optimally polymerized , and dividing the remaining sample thickness by two has been considered to provide a ‘safe’ measure of adequate cure.
2.2
Degree of conversion (DC)
DC was determined using a Fourier Transform Infra-Red Attenuated Total Reflectance equipment (FTIR-ATR, Nicolet 6700, Thermo scientific, Milan, Italy). A silicon mold was used to prepare disk-shaped specimens (height = 4 mm, diameter = 4 mm) of each composite ( n = 5), which were placed and polymerized in bulk. During photopolymerization a mylar strip was positioned between the curing tip and the specimen to exclude oxygen. Photopolymerization was performed for 20 s, using the same light-curing unit as in the preparation of specimens for DOC measurements, and applying the curing tip on the top of the specimen. The specimens were then placed on the diamond crystal of a horizontal attenuated total reflectance (ATR) stage of the FTIR equipment. Infrared (IR) spectra were obtained between 4000 and 500 cm −1 at a resolution of 6 cm −1 on top and at the bottom of each specimen immediately after photopolymerization. For the methacrylates-based composites, the FTIR bands used to calculate the DC were the carbonyl (C O) peak at 1715 cm −1 as an internal reference and the vinyl C C peak at 1635 cm −1 as a reaction peak. For the silorane composite the signals used to determine the DC were the stretching vibration of the epoxy ring (C O C) at 884 cm −1 as a reaction peak and of the Si CH 3 bond at 695 cm −1 as a reference peak. The DC was calculated using the ratio between the reaction and internal reference peak (Eqs. (1) and (2) for methacrylates and silorane respectively)
DC % = 1 − ( ( C = C ) ( t = n ) / ( C = O ) ( t = n ) ) ( ( C = C ) ( t = 0 ) / ( C = O ) ( t = 0 ) ) × 100
DC % = 1 − ( ( C – O – C ) ( t = n ) / ( Si – CH 3 ) ( t = n ) ) ( ( C – O – C ) ( t = 0 ) / ( Si – CH 3 ) ( t = 0 ) ) × 100
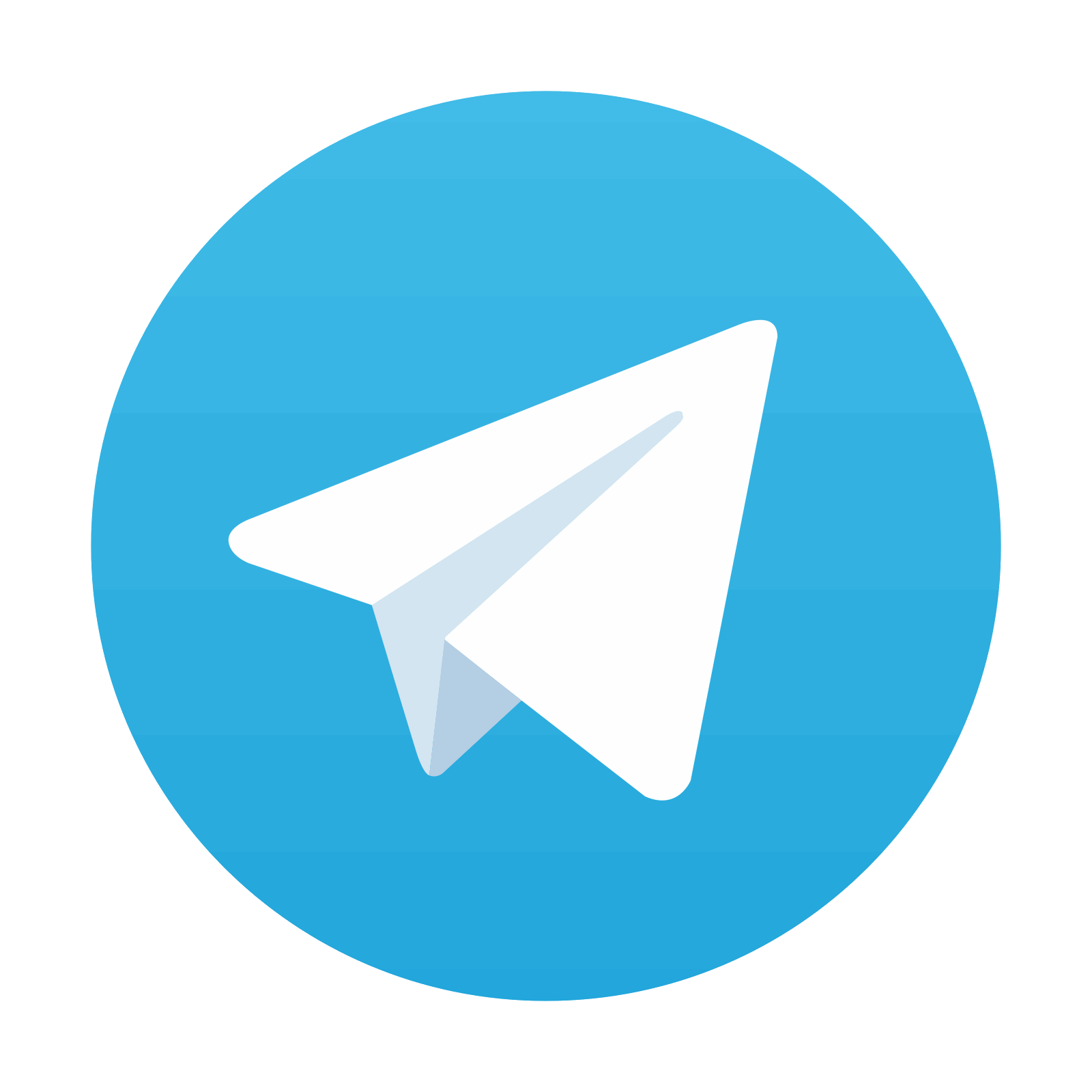
Stay updated, free dental videos. Join our Telegram channel

VIDEdental - Online dental courses
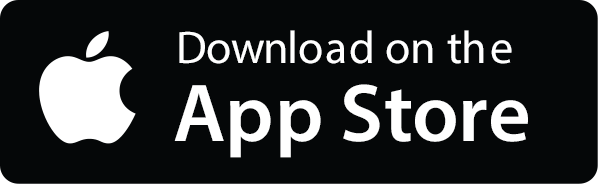
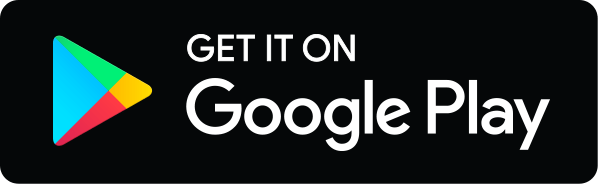