Plaque metabolism and dental disease
Publisher Summary
This chapter discusses plaque metabolism. Dental plaque is noticeable in the sheltered areas provided by fissures, the points of tooth contact, and the gingival margins. These are the areas that encourage food retention and are susceptible to carious attack. Caries only occurs to a limited extent on other surfaces that are accessible and subject to self-cleansing, and the incidence of caries at any particular site appears to be inversely related to its exposure to saliva. The lower anterior teeth that are continually bathed in saliva are, in general, caries-resistant, whereas the upper anteriors that have a limited saliva access are caries-prone. On the other hand, regions that are exposed to saliva, especially the lingual side of the lower incisors and the buccal side of the upper molars, tend to develop supragingival calculus in later life. A carious attack on the teeth must result from interaction between the oral bacteria and the food. Only those bacteria that can adhere effectively to the tooth surface and produce appreciable amounts of acid will cause demineralization; the types of the bacteria present and their metabolic activities depend on the properties of plaque.
If plaque is not removed soon after it is formed, it thickens and becomes less permeable. This limits the supply of oxygen and the number of anaerobic organisms increases at the expense of the aerobic types, although the streptococci, which are facultative anaerobes,* remain relatively constant. The bacterial composition of mature plaque is quite different from that found elsewhere in the mouth. Plaque which is a week old contains cocci, rods, filaments, vibrios and spirochaetes among many others. The bacteria are closely packed and often dominated by filamentous organisms which lie at right angles to the enamel surface. Coccal forms have been observed in close association with the filaments, and the outer layers of the plaque contain large numbers of Gram-positive and Gram-negative cocci (Table 33.2). Distinct regions may be observed within the plaque, each of which is dominated by a particular bacterial type, and this results in the existence of a variety of physical and chemical gradients which may affect bacterial growth and survival. Adjacent sites may be very different, not only with respect to the type and amount of nutrients available, but also in their oxygen content, pH, ionic strength and content of toxins. Consequently, although it is convenient to regard the plaque as a single metabolic entity, it actually consists of many relatively independent micro-territories whose biochemical properties may be appreciably different from those of the plaque as a whole.
From the metabolic point of view, plaque bacteria can be divided into two main groups, namely, those which utilize nitrogenous materials and produce basic substances which tend to cause a rise in pH, and those which convert carbohydrates into organic acids and tend to lower the pH. Although the metabolism of a particular microorganism may be primarily based on one or other type of activity, many bacteria use both kinds of substrate. However, in any given situation within plaque, the type of micro-organism that predominates will depend to a large extent on the supply of substrates. These may either be exogenous, i.e. transient substrates of dietary origin, or endogenous substrates, which are present in the plaque fluid at virtually all times. This fluid is mainly derived from the saliva but, in the gingival regions, the gingival fluid makes a significant contribution. The substrates include urea, amino acids, peptides, proteins, glycoproteins and cell debris, as well as extracellular glucans and fructans. How these various materials are utilized will depend on the types of organism present and the existing conditions. In a developing plaque, where energy is required for biosynthetic reactions, glucose will be broken down by glycolysis but the fate of the pyruvate will depend on the availability of oxygen. Aerobic conditions, which allow the pyruvate to be completely oxidized to carbon dioxide and water via the citrate cycle and respiratory chain, are found only at the outer surface of the plaque. In the interior of a thick, rapidly growing plaque the inwardly diffusing oxygen is rapidly exhausted and in the resulting anaerobic conditions the pyruvate is converted into lactate. Other organic acids, such as acetic, butyric, formic and propionic, are produced as well and are responsible for the low pH values often found in dense plaque. A simplified scheme showing how these acids may be derived from pyruvate in anaerobic bacterial systems is given in Figure 35.1.
Sugar metabolism and acid production
Streptococci are the most important organisms in terms of acid production and special features of their metabolism are worth mentioning. They can take up sugars from the surrounding medium by a high-affinity transport mechanism which is energized by phosphoenolpyruvate (page 534) and is known as the phosphotransferase system. It is specific for individual sugars and operates at neutral pH values. This allows the organism to survive at low sugar concentrations and neutral pH values, conditions which are found naturally in the saliva and on the enamel in the absence of food. In addition, streptococci have a second, low affinity sugar uptake system which is activated by a protonmotive force (pmf) (page 221). This operates maximally in S. mutans at pH 5·5 and allows the organism to take advantage of the pH gradient generated when the extracellular pH is low compared to the intracellular pH. Consequently, the cell can continue to transport and metabolize sugars under acidic conditions which are unfavourable to other bacteria. This adaptation to a sugar-rich environment and a low pH make it a dominant organism in cariogenic plaques.
Streptococci do not contain enzymes of the citrate cycle, nor do they possess cytochrome systems but, instead, they rely on anaerobic glycolysis for their energy. The fate of pyruvate produced by this pathway is then determined by the overall availability of sugars. The enzyme lactate dehydrogenase is allosterically activated by the glycolytic intermediate fructose 1,6-bisphosphate, which together with glyceraldehyde 3-phosphate tends to accumulate when sugar is readily available so that lactate formation is favoured (Figure 35.2). The alternative pathway, catalysed by the enzyme pyruvate formate lyase, is inhibited by glyceraldehyde 3-phosphate so that this pathway only becomes operative when glycolytic intermediates are low, that is when sugar is in short supply. This allows the organism to generate an extra molecule of ATP for each glucose utilized, from the intermediate acetyl phosphate.
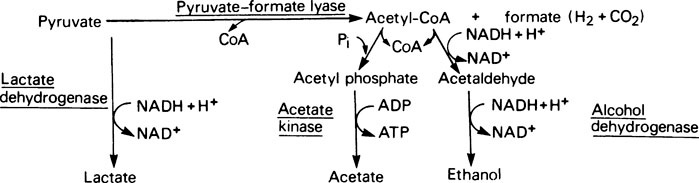
< ?xml:namespace prefix = "mml" />

In practice, it is not possible to equate plaque acid production directly with the presence of any one type of bacterium. Analyses of organic acids in overnight fasting plaque before exposure to dietary sugar showed a predominance of acetic acid with some propionic, butyric and formic acids. The total acid concentration increased immediately after exposure to sugar, mainly due to the formation of lactic acid, while the concentration of the other acids tended to decrease. Fasting plaque has a neutral or slightly alkaline pH, and Stephan in 1940, using a micro pH electrode, demonstrated that the application of a sugar solution caused the intraplaque pH to fall rapidly to about pH 5.5 within 2–3 min, corresponding to the maximum rate of lactic acid production. It took up to 40 min for the pH to return to resting conditions, as lactic acid was slowly replaced by the other organic acids. Modern techniques using indwelling electrodes fixed in the dentition allow plaque pH to be measured continuously and values as low as pH 4·0 have been measured in response to sucrose. Acid is produced from a range of carbohydrate-containing foods, fruits and juices and this technique is used to determine the potential cariogenicity of foodstuffs. pH changes vary from site to site depending on salivary access and plaque thickness and typical Stephan curves are shown in Figure 35.3.
Nitrogen metabolism and base production
The provision of a source of nitrogen is just as important for bacterial growth as the provision of a source of energy and reference of Table 33.1 (page 478) shows that salivary urea is the most readily available source. Plaque possesses a high urease activity and a number of different reactions are known by which microorganisms incorporate ammonia into amino acids, namely:
Subsequent transamination reactions then provide the other amino acids needed for protein synthesis. Glutamine serves as a –NH2 donor in a number of biosynthetic reactions and Figure 35.4 shows how carbohydrate and nitrogen metabolism may be interrelated in the plaque. An additional source of amino acids within the interior may be provided by hydrolysis of matrix proteins.
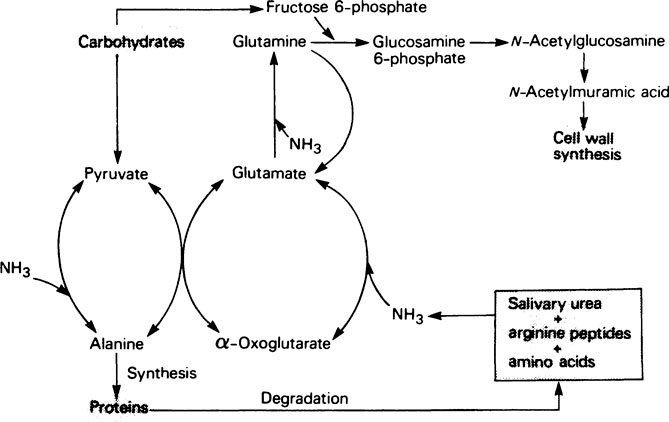

This pathway which is widely distributed in bacteria, acts as an energy source under anaerobic conditions, and produces a marked pH-rise effect due to the production of NH3. It is induced by arginine supplementation. It also acts as a source of ornithine which can subsequently be decarboxylated, particularly at low pH values, to produce an increase in the pH (page 508) and the evil-smelling amine putrescine which occurs in decaying meat.

In a long-established plaque, when bacterial growth is limited, more ammonia may be produced than is required for amino acid production. Accumulation of either ammonia or organic acids will influence the acid-base ratio and hence the pH of the plaque. However, when the system is an open one and there is a good flow of saliva, pH changes will be resisted since the bacterial waste products are able to diffuse away from their site of origin (Figure 35.5a). This is the situation in the thin loosely aggregated type of plaque which contains little or no extracellular polysaccharide. However, if plaque is allowed to accumulate, through inadequate oral hygiene, formation of extracellular polysaccharides, especially when sucrose is readily available, will lead to the formation of closed plaque which retains organic acids and ammonium salts within its matrix (page 497). The accumulation of these charged metabolites is greatest at sites where access for saliva is poor, resulting in pronounced changes in the pH which only returns slowly to normal after the supply of substrate has been exhausted (Figure 35.5b). Sometimes a new steady state may be established at a higher or lower pH value and this may provoke a long-term modification in the plaque flora which will cause pathological changes in the host.
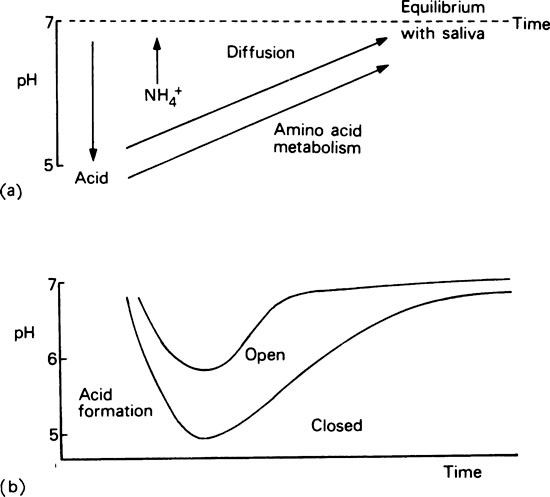
Certain factors tend to counteract changes in plaque pH.
1. As shown in Figure 35.5(a) ammonia produced at acid pH values will tend to neutralize accumulated acid, while acid that is produced under alkaline conditions will neutralize accumulated base.
2. Pyruvic and 2-oxoglutaric acids may take up ammonia to form amino acids (page 280), thereby removing both acidic and basic metabolites. This could explain the relatively high levels of alanine and glutamic acid found in aqueous extracts of plaque.
3. Metabolites produced by one type of bacterium may be used by another type; for example, Veillonella are unable to break down carbohydrates but utilize lactic acid as an energy source instead. Their numbers have been found to increase with the number of streptococci.
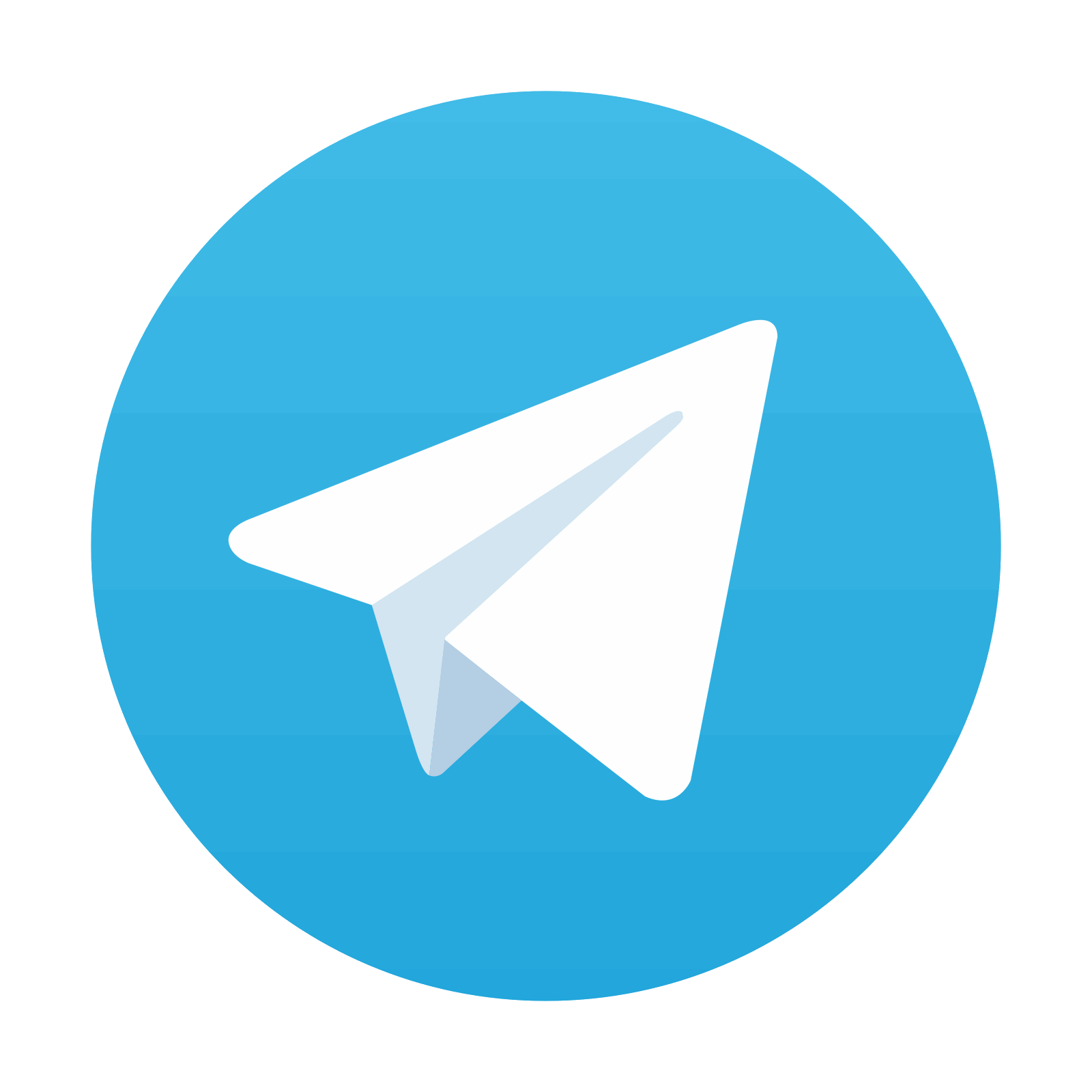
Stay updated, free dental videos. Join our Telegram channel

VIDEdental - Online dental courses
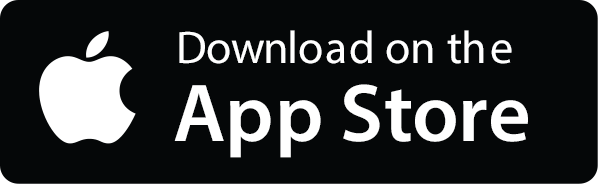
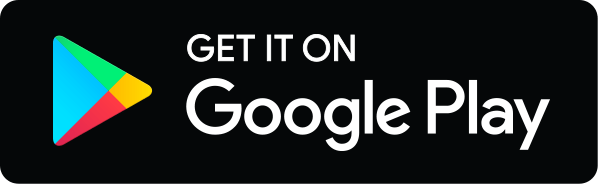