Introduction
Mandibular molar uprighting is indicated when mesial inclination of the second molars occurs because of missing first molars. There are many methodologies to perform such movement. In this study, we aimed to analyze and compare the stress distributions in different molar uprighting techniques.
Methods
Four photoelastic models were designed to evaluate different mandibular second molar uprighting techniques: a miniscrew positioned in the retromolar region, a beta-titanium alloy cantilever spring, a beta-titanium alloy wire with a T-loop spring, and an 0.018-in stainless steel archwire with an open-coil spring between the second premolar and the second molar.
Results
On the miniscrew test specimen, the greatest concentration of strains was observed in the cervical zone of the distal root. The cantilever spring had many strains in the cervical zone of the mesial root. On the T-loop spring test specimen, mainly the observed strains were in the apical zone of the mesial root. The open-coil spring specimen showed fringes in the cervical zone and the apical zone of the mesial root without formation of large sequences of strains.
Conclusions
The miniscrew mechanical action had the least and the cantilever spring mechanical action had the greatest strain means on the roots of mandibular second molars.
Highlights
- •
We analyzed 4 second molar uprighting techniques.
- •
The strain distribution on the mandibular second molar roots was investigated.
- •
Photoelasticity is useful to verify the strain on the dental roots during mechanical loads.
- •
The miniscrew technique induced lower strain means on the second molar roots.
- •
The cantilever technique induced greater strain means on the mandibular second molar roots.
One frequently encountered situation in adult patients is the mesial inclination of mandibular molars; it may be caused by early loss of a deciduous or permanent molar, or agenesis. This inclination can cause periodontal problems and extrusion of the antagonist tooth. A traumatic occlusion may be a consequence, due to premature contacts caused by interferences in centric relation and mandibular excursive movements. Oral rehabilitation in these patients is more difficult, and molar uprighting is indicated. This orthodontic movement is useful and necessary because it allows for better distribution of occlusal forces parallel to the long axes of the teeth. As a result, it minimizes side effects such as vertical bone defects, promoting improvement in the injured periodontal tissue in the inclined teeth as well as space recovery for prosthetic oral rehabilitation.
The following are several mechanical techniques for uprighting these teeth: box spring, T-loop spring, helicoide spring, tipback spring, distal jet, simple technique for molar uprighting, open-coil spring, cantilever, and miniscrew.
One possibility for studying the effects caused by mandibular molar uprigthing is photoelasticity, considered a technique based on birefringence—an optimechanical property of transparent polymers. This is an experimental method used in various studies in orthodontics that analyzes the stress and strain fields of structures in the areas of compression and traction.
Although uprighting is frequently performed by orthodontists, it is difficult to find studies in the literature that have examined the effects of the stress produced in periodontal tissues on teeth in response to mandibular second molar uprighting. For this reason, we aimed to analyze and compare the stress distributions in the following 4 molar uprighting techniques using photoelasticity: miniscrew, cantilever, T-loop spring, and open-coil spring.
Material and methods
In this study, 4 artificial models made from flexible epoxy resin, artificial teeth, and a miniscrew were used. To produce them, a typodont was built with wax and artificial teeth, simulating the clinical situation of a mandibular hemiarch in an adult with a missing first molar and mesial inclination of the second molar. Then an impression of this typodont was taken with silicon (Moldflex Products, São Paulo, Brazil); afterward, the artificial teeth (Orto-Art, Piracicaba, Brazil) and miniscrew (Neodent, Curitiba, Brazil) were positioned in the silicone impression. A flexible epoxy resin was poured into this impression (Epoxi Glass, Diadema, Brazil). In model 1, a miniscrew was positioned in the retromolar region. An orthodontic button was bonded to the mesial aspect of the mandibular second molar, and force was applied with an elastic power chain that was loaded from the screw to the molar ( Fig 1 ). In model 2, brackets were bonded to the premolars and second molar, and a 0.019 × 0.025-in stainless steel archwire was used as an anchorage system; also, a 0.019 × 0.025-in beta-titanium alloy cantilever spring was inserted into the mandibular second molar and supported on the segmented arch between the premolars ( Fig 2 ). In model 3, brackets were bonded to the premolars and second molar, and a 0.019 × 0.025-in beta-titanium alloy wire with a T-loop spring was used ( Fig 3 ). In model 4, brackets were bonded to the premolars and second molar, and an 0.018-in stainless steel archwire with an open-coil spring between the second premolar and second molar was used ( Fig 4 ). A moment of force diagram of each type of mechanics ( Fig 5 ) describes the expected movement for these 4 scenarios.





The isocrometric fringes were observed in a circular polaroscope. The lack of initial stress induced in each model was verified before the tests. Force intensities of 50, 100, 150, 200, 250, and 300 g were applied. After each activation, photographs were taken; then the images were transferred to a computer with a 21-in screen for analysis.
The appearance of optical fringes is the visible expression of loads used in photoelastic models that cause resin deformation, showing areas of stress. By applying a load in an unloaded piece, the fringes initially appear in points with a higher stress level. By increasing the load, new fringes will appear, and the previous fringes will be redistributed to lower stress areas. The fringes can be designated by ordinal numbers according to their appearance, and they have an individual identity (order) that has been classified internationally and described by the American Society for Testing and Materials (D4093). Areas with fine fringes and small spaces indicate zones with a high strain. Large black or gray areas usually suggest the absence of strain. The fringes are recognized when the transition from 1 band to another occurs from the change of fringe coloration. The first one, of black color, is the one of order 0; the one of violet color, order 1; the violet/blue transition, order 2; and transition from red to green, order 3. From the fringe of order 3, the subsequent fringes are always counted in the transition from red to green. These fringes are used for qualitative and quantitative analyses. In qualitative analysis, we observe the zones where the fringe is formed as well as its morphology and density. In the quantitative analysis, numeric orders of the fringes are used in the sites of interest. In this study, we evaluated the areas surrounding the mandibular second molar root—mesial root cervical zone, mesial root apical zone, distal root cervical zone, and distal root apical zone—when different vertical movements were applied.
Statistical analysis
The values of the fringes were described in each measurement site with descriptive statistical terms (means and standard deviations), verifying agreement and reproducibility between the measurements using intraclass correlation coefficients with 95% confidence intervals, and the measure repeatability was calculated, which is the estimate of the differences between assessments.
The values of the first assessment of the fringes were described for each site and mechanical type using summary measures (means, standard deviations, medians, minimums, and maximums), and the fringes of the 4 mechanical types were compared with the nonparametric Friedman test. When the test showed statistical significance, multiple nonparametric paired comparisons were performed to verify the types of mechanical differences on the fringes. The tests were performed with a significance level of 5%.
Results
In the qualitative analysis, the following results were observed.
For test specimen 1,—miniscrew,—it was possible to observe that the fringes were basically expressed around the miniscrew. In the studied sites, the greatest concentration and number of fringes were found in the cervical zone of the distal root, which was more intense from the application of 200 g, when an increase in the strains around the mesial root apices was also observed. Strains were not found in the cervical zone of the mesial root in any applied load ( Fig 6 ).
For test specimen 2—cantilever spring—greater strains were observed from the application of 100 g, especially in the missing first molar region. As the load was increased, new fringes arose, which also affected the teeth of the anchoring segment (premolars and canines) that were extended from the edge of the ridge to the middle third of the second molar root. They appeared in greater numbers and concentrations, especially in the mesial root cervical zone and the distal root apical zone ( Fig 7 ).
For test specimen 3—T-loop spring—the fringes arose, showing a color sequence during the initial loading in the missing mandibular first molar region that apparently originated from the distal zone of the second premolar heading toward the second molar. As the loading reached 150 g, fringes were observed in the studied zones, mainly on the mesial root apical zone; as the loading was increased, more fringes arose including other zones. However, they lacked a visible color sequence formation ( Fig 8 ).
For test specimen 4—open-coil spring—strains were already observed in the 50-g loading, and they increased as the loading increased. Fringes were found in the cervical zone and the mesial root apical zone; however, the formation of large sequences of colors was not verified, as in other test specimens ( Fig 9 ).
The results obtained by the quantitative analysis are described in Tables I and II . Table I describes the comparisons between the mechanical types in predefined locations for classification of the fringes. Table II gives the paired nonparametric multiple comparisons of the fringes among the mechanical types. Figure 10 is a chart with a comparison among the fringe sequence means of each mechanical type.
Mechanical type and zone | Appraisal 1 | Appraisal 2 | Intraclass correlation coefficient | 95% CI | Reproducibility | |||
---|---|---|---|---|---|---|---|---|
Mean | SD | Mean | SD | Lower bound | Upper bound | |||
Miniscrew/cervical zone of mesial root | 0.00 | 0.00 | 0.00 | 0.00 | ∗ | |||
Miniscrew/apical zone of mesial root | 0.33 | 0.52 | 0.33 | 0.52 | 1.000 | 0.00 | ||
Miniscrew/cervical zone of distal root | 0.50 | 0.84 | 0.50 | 0.84 | 1.000 | 0.00 | ||
Miniscrew/apical zone of distal root | 0.33 | 0.52 | 0.33 | 0.52 | 1.000 | 0.00 | ||
Cantilever spring/cervical zone of mesial root | 2.17 | 1.33 | 1.67 | 1.03 | 0.835 | 0.159 | 0.976 | 0.39 |
Cantilever spring/apical zone of mesial root | 1.50 | 0.84 | 1.67 | 1.03 | 0.906 | 0.543 | 0.986 | 0.29 |
Cantilever spring/cervical zone of distal root | 0.17 | 0.41 | 0.17 | 0.41 | 1.000 | 0.00 | ||
Cantilever spring/apical zone of distal root | 0.00 | 0.00 | 0.00 | 0.00 | ∗ | |||
T-loop spring/cervical zone of mesial root | 0.67 | 0.52 | 0.67 | 0.52 | 1.000 | 0.00 | ||
T-loop spring/apical zone of mesial root | 1.67 | 0.52 | 1.33 | 1.03 | 0.762 | 0.123 | 0.962 | 0.37 |
T-loop spring/cervical zone of distal root | 0.17 | 0.41 | 0.17 | 0.41 | 1.000 | 0.00 | ||
T-loop spring/apical zone of distal root | 0.67 | 0.52 | 0.67 | 0.52 | 1.000 | 0.00 | ||
Open-coil spring/cervical zone of mesial root | 0.67 | 0.52 | 0.67 | 0.52 | 1.000 | 0.00 | ||
Open-coil spring/apical zone of mesial root | 0.83 | 0.41 | 0.83 | 0.41 | 1.000 | 0.00 | ||
Open-coil spring/cervical zone of distal root | 0.17 | 0.41 | 0.17 | 0.41 | 1.000 | 0.00 | ||
Open-coil spring/apical zone of distal root | 0.17 | 0.41 | 0.00 | 0.00 | 0.00 | −0.754 | 0.754 | 0.29 |
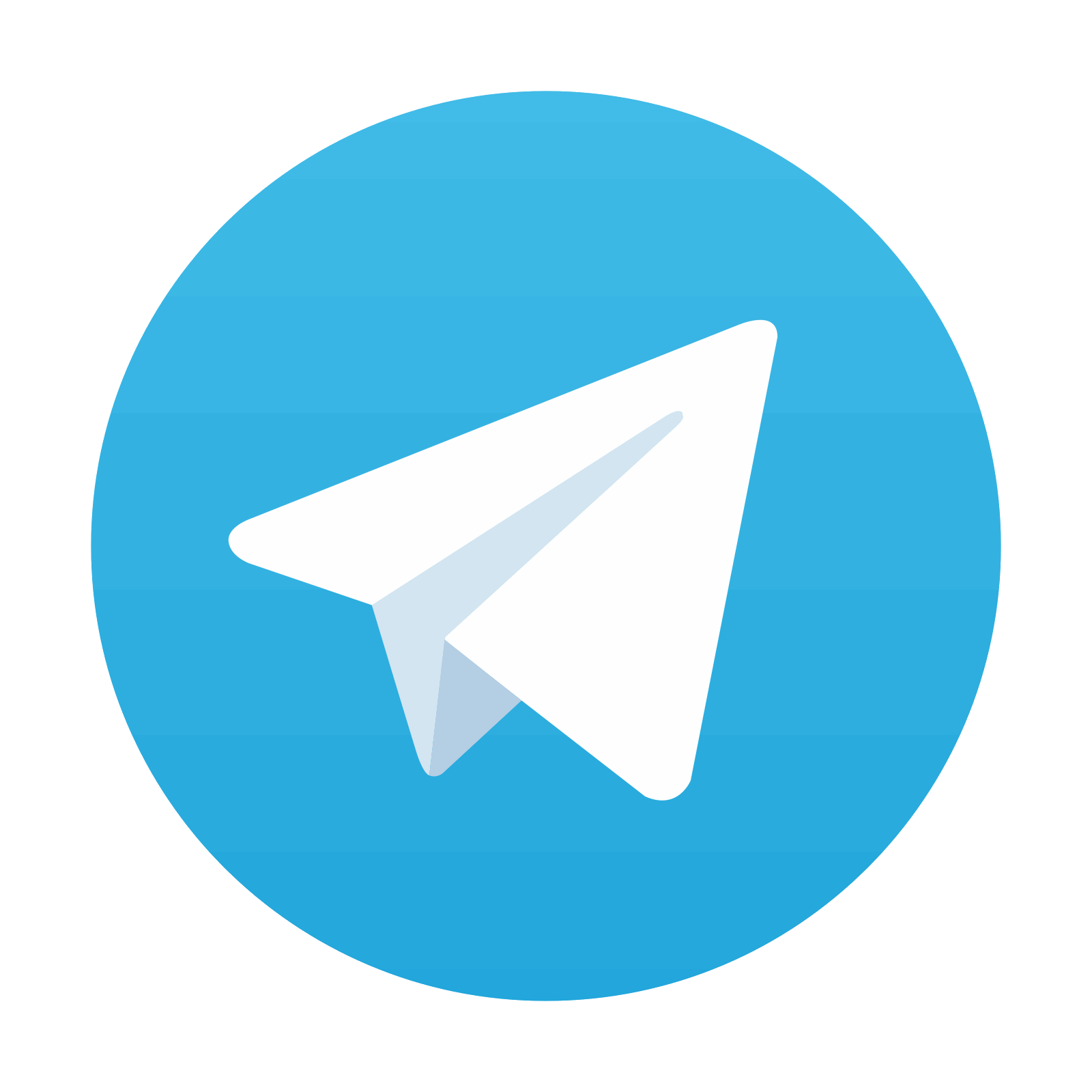
Stay updated, free dental videos. Join our Telegram channel

VIDEdental - Online dental courses
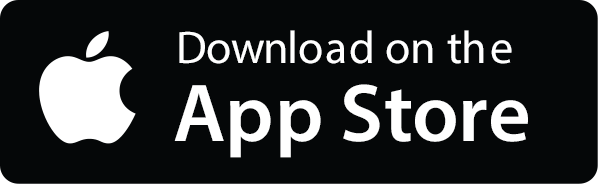
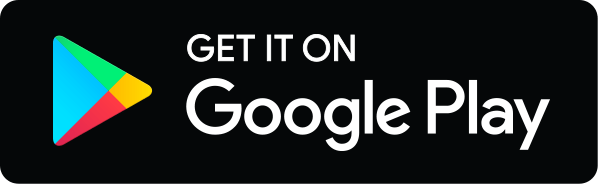
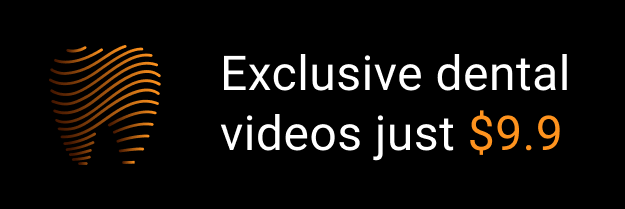