Abstract
Objectives
To evaluate whether the concentration of phosphoric acid (PA) has an effect on the proteolytic activity of sound human demineralized dentin. It is hypothesized that the activity of matrix-bound and extracted enzymes depends on the PA concentration used to demineralize dentin.
Methods
One-gram aliquots of mid-coronal human dentin powder were demineralized with 1 wt%, 10 wt% and 37 wt% PA. Concentrations of released calcium were measured for each set of demineralization. Extracted MMP-2 was immunologically identified by western blot and its activity was determined by conventional gelatin zymography. Analysis of released hydroxyproline (HYP) and in situ zymography were performed to evaluate the activity of insoluble, bound-matrix enzymes.
Results
The amount of released calcium from dentin powder treated with 37 wt% PA was significantly higher (p ≤ 0.05) than that obtained by dentin demineralization with 10 wt% and 1 wt% PA. Expression and activity of endogenous enzymes, extracted from or bound to dentin matrix, were detected for all samples regardless of the PA concentration. However, the expression and activity of extracted MMP-2 were significantly higher when dentin was treated with 10 wt% PA (p < 0.05), followed by 1 wt% and 37 wt% PA. Similarly, the highest concentration of released HYP (i.e. meaning higher percentage of collagen degradation) and the highest activity in in situ zymography were observed when dentin samples were treated with 10 wt% PA (p < 0.05).
Conclusions
It was confirmed that PA does not denature endogenous enzymes of dentin matrices, but it may somehow modulate the expression and activity of these enzymes in a concentration-dependent manner.
Clinical significance
Endogenous proteases have been identified and suggested to be responsible for the digestion of dentin matrix when activated by the acidic components of dental adhesives. Proteolytic activity of dentinal MMPs showed to be dependent on phosphoric acid concentration. The clinically-used concentration (37%) does not inhibit MMPs activity, but slows it.
1
Introduction
Since the concept of acid-etching dentin has shown to be safe and provide consistent immediate bond strength , dental bonding procedures are based on either direct (i.e. etch-and-rinse approach) or indirect (acidic resin monomers derived from phosphoric acid for self-etching approach) acid-etching to partially solubilize dentin minerals and superficially expose the dentin organic matrix, including – its major component – collagen type I as well as non-collagenous proteins and proteases.
A critical problem in bonding procedures is that both etch-and-rinse and self-etch adhesives are not able to completely displace the free and loosely bound water from the internal and external water compartments of collagen fibrils . The consequence is that porous/defective resin-dentin bonded interfaces and that compromise their mechanical and biochemical integrity after prolonged function.
Incomplete resin infiltration results in collagen fibrils being surrounded by water instead of resin, which causes hydrolysis of resin by esterases and collagen fibrils by endogenous and exogenous collagenolytic enzymes . Matrix metalloproteases (MMPs) and cysteine cathepsins are two classes of endogenous proteases that have so far been described as being in the dentin-pulp complex, with the potential capacity to mediate the hydrolysis of collagen and other extracellular dentin matrix proteins . In dentin matrices, collagenases (MMP-8) and gelatinases (MMP-2 and -9) can cleave native triple-helical type I collagen into 1/4- and 3/4-fragments at the Gly-Leu/Isoleu peptide bond and telopeptides .
Initially, it was thought that acid-etching might release and activate pro-MMPs trapped within the mineralized dentin . However, clinical etching of dentin with 37% phsorphoric acid (PA) has been reported to decrease MMP activity . The subsequent application of relatively acidic resin components (i.e. primers\with pH higher than 37% PA) in etch-and-rinse adhesives or self-etch adhesives has been then shown to increase the collagenolytic and gelatinolytic activities of completely or partially demineralized collagen matrices.
The activity of dentin proteases has usually been assayed after extraction from mineralized dentin . When some investigators have proteases from the dentin matrix using low and intermediate concentrations of PA (1% and 10%), they reported higher intrinsic proteolytic activity of demineralized dentin compared to those obtained when using 37% PA. While suggestive, it is not clear yet whether the opposing effects of PA, either increasing or decreasing proteases activities, are due to differences in PA concentrations or to the formation of insoluble calcium phosphate precipitates that cover enzymes, hence reducing their potential activity . We believe that once activated, the insoluble matrix-bound enzymes are those with actual potential to degrade collagen fibrils and, ultimately, contribute for the degradation of dentin matrix. Thus, the aim of this study was to evaluate the effect of different concentrations of PA on the enzymatic activity of MMPs, in especial of MMP-2, the most abundant and robust MMP in pulp-dentin complex, when extracted from or left bound to dentin matrix. It was hypothesized that PA concentration affects dentinal MMPs activity regardless of whether the enzyme is extracted or remains bound to the dentin matrix.
2
Material and methods
Reagents were purchased from Sigma Chemical Co. (St. Louis, MO, USA) unless otherwise specified. A flowchart summarizes the study experimental conditions ( Fig. 1 ).
2.1
Dentin powder preparation and demineralization
Twenty human molars were collected from the human teeth bank of the Dental School of the University of São Paulo, wherein this protocol was further revised and approved by the Ethics Committee (Protocol 16/11–n° 0014.0.017.000-11). After complete removing enamel, cementum and pulp tissue from all teeth, fragments of mid-coronal dentin were obtained, pooled, dried and frozen at −80 °C for 24 h. Those fragments were further reduced in a ball-mill (MM401, Retsch, Newtown, USA) at 30 Hz for 7 min. The resultant powder was sieved (#400 mesh) and three aliquots of 1 g each were treated with phosphoric acid (PA) solutions in different concentrations: 1% (pH 1.4), 10% (pH 1.0) and 37% (pH 0.4) for 10 min, 1 min or 20 s, respectively ( Fig. 1 ). This variation of time application was chosen in order to allow a proportional demineralization of dentin between testing solutions. Thus, since 10% and 37% PA solutions are, respectively, 10 and 37 times more concentrated than the 1% solution, their time of application were, accordingly, reduced 10- and 37-fold. To neutralize the acid demineralization of dentin, 4 M NaOH was added in volumes that varied from 0.2 to 8.6 mL to complete react with PA. Protein extraction from demineralized dentin powder was performed using the protocol described by Breschi et al. (2010). Briefly, the samples were re-suspended in 4 mL extraction buffer (50 mM Tris-HCl containing 5 mM CaCl 2 , 100 mM NaCl, 0.1% Triton X-100, 0.1% NONIDET P-40, 0.1 mM ZnCl 2 , 0.02% NaN 3 ) for 24 h at 4 °C under stirring. Then, they were centrifuged, the supernatants were collected and protein content was precipitated with 25 wt% trichloroacetic acid (TCA) at 4 °C. TCA precipitates were re-solubilized in 1.5 M Tris–HCl (pH 8.8). Aliquots of mineralized dentin powder were used as negative controls; they were processed as the other samples with the exception they were not treated with phosphoric acid, but instead remained mineralized and stored in extraction buffer. Total protein concentration was determined by the Lowry et al. method.
2.2
Measurement of calcium released from mineralized dentin
The neutralized supernatants obtained after demineralization of dentin powder were used for determination of calcium content ( Fig. 1 ). 1-mL aliquots of each supernatant (1%, 10% and 37% PA) were transferred to plastic vials containing 0.5 mL of 0.1% chloranilic acid and kept undisturbed for 30 min. The samples were centrifuged (for 30 min at 1200 rpm – rotor Sorvall SM-24), the respective supernatants were discarded and pellets were re-suspended in 3 mL of 50% isopropyl alcohol. Further centrifugation was performed (for 30 min at 1200 rpm − rotor Sorvall SM-24), the supernatant was again discarded and 3 mL of 5% EDTA was added to the resultant precipitate . The same procedures were performed for the supernatant (extraction buffer) of negative controls (mineralized dentin powder). All samples were analyzed against a standard curve of known concentrations of calcium (0.25, 0.50, 0.75, 1.00 mg/mL) in a spectrophotometer (Beckman Coulter DU-800, California, USA) at 520 nm wavelength. The same Experiments were run in triplicates.
2.3
Measurement of collagen solubilization (Hydroxyproline assay)
Precipitates obtained after the protein extraction were lyophilized and separated into 10 aliquots (40 mg each) of each experimental condition (1%, 10% or 37% PA) ( Fig. 1 ). Samples were incubated in 1 mL of artificial saliva (AS) (50 mmol/L HEPES, 5 mmol/L CaCl 2 , 0.001 mmol/L ZnCl 2 , 150 mmol/L NaCl, 0.3 mmol/L PMSF, 3 mmol/L NaN 3 ) at 37 °C for 24 h under stirring. After incubation, the AS storage medium was completely removed and analyzed for dentin collagen solubilization by measuring the amount of hydroxyproline (HYP) released. The same procedures were performed for the negative controls (mineralized dentin powder). Measurement of HYP was determined using a colorimetry assay, slightly modified from that described by Reddy & Enwemeka . Briefly, 500 μL of supernatant was lyophilized, re-suspended in 10 μL deionized water and 40 μL of 2 N NaOH was added and were hydrolyzed by autoclaving (at 120 °C) in two cycles of 45 min each. Then, samples’ oxidation was performed with chloramine-T for 25 min and the chromophore was developed by incubation with Ehrlich’s reagent for 40 min at 65 °C. Absorbance values were measured in a spectrophotometer (Beckman Coulter DU-800, USA) against standard curves of known concentrations of HYP (2, 5, 10, 15 and 25 μg/mL) and the solubilized collagen was expressed as micrograms of HYP per milligram of demineralized dentin (μg/mg dentin).
2.4
Western blot
To confirm the immunological identity of MMP-2 in the extracts, the supernatant obtained after protein extraction (80 μg of protein) were electrophorezed on 10% SDS-PAGE gels ( Fig. 1 ) under reducing conditions and proteins were transferred with a semi-dry protocol (Trans-Blot, Bio-Rad, California, USA) to nitrocellulose membranes (Bio-Rad, California, USA). After washing with TBS–Tween-20, the membranes were incubated with primary antibody, overnight at 4 °C. Polyclonal primary antibody was rabbit anti-human MMP-2 (Abcam Inc., Massachusetts, USA) diluted (1:500) in 0.01% PBS Tween-20 buffer. Following washings, membranes were incubated with goat polyclonal secondary antibodies (Abcam Inc., Massachusetts, USA) (1:10,000) for 1 h at room temperature. Immunoreactive bands were detected by chemiluminescent reagents (ECL, Abcam Inc., Massachusetts, USA) on radiographic films (GE, England) and evaluated by densitometry with image analysis program (Scion Image, www.scioncorp.org).
2.5
Conventional gelatin zymography
Precipitates obtained after the protein extraction (80 μg) were electrophorized ( Fig. 1 ) under non-reduced conditions on 10% SDS-polyacrylamide gel containing 1 mg/mL fluorescent gelatin, which was previously labeled with MDPF (2-Methoxy-2,4-diphenyl-3(2H)-furanone) . Enzymes activation was achieved with 2 mM p -aminophenylmercuric acetate (APMA) for 1 h at 37 °C. After electrophoresis, the gels were washed for 1 h in 2% Triton X-100 and were then incubated in activation solution (50 mM Tris-HCl, 5 mM CaCl 2 pH 7.4) for 24 h. Negative control gels were incubated with the same buffer containing 2 mM of 1,10-phenanthroline. After incubation, the zymograms were photographed under UV illumination (Quantity One, BioRad, California, USA) and evaluated by densitometry using an image analysis program (Scion Image, www.scioncorp.org). Experiments were run in triplicates.
2.6
In situ zymography
Mid-coronal dentin slices (0.3 mm) were acid-etched with the tested PA solutions (1%, 10% and 37%) under conditions previously described. In situ zymography was performed using a quenched fluorescein-conjugated gelatin as substrate (E-12055, Molecular Probes, Eugene, USA), as described by Mazzoni et al. . 80-μL of that gelatin substrate were placed on top of each dentin slab and incubated in a dark humid chamber at 37 °C for 24 h. The hydrolysis of quenched fluorescein-conjugated gelatin was assessed by a multi-photon confocal microscope, with an excitation wavelength of 488 nm and emission wavelength of 530 nm (Zeiss, LSM 780, Carl Zeiss, Oberkochen, Germany). Optical sections of 85 μm thick were acquired and the stacked images were analysed, quantified, and processed with ZEN 2010 software (Carl Zeiss).
2.7
Statistical analysis
Results were analyzed by ANOVA and Student-Newman-Keuls test for multiple comparisons (α = 0.05) for western blot and conventional zymograph and Kruskal-Wallis and Student-Newman-Keuls test (α = 0.05) for calcium and HYP release.
2
Material and methods
Reagents were purchased from Sigma Chemical Co. (St. Louis, MO, USA) unless otherwise specified. A flowchart summarizes the study experimental conditions ( Fig. 1 ).
2.1
Dentin powder preparation and demineralization
Twenty human molars were collected from the human teeth bank of the Dental School of the University of São Paulo, wherein this protocol was further revised and approved by the Ethics Committee (Protocol 16/11–n° 0014.0.017.000-11). After complete removing enamel, cementum and pulp tissue from all teeth, fragments of mid-coronal dentin were obtained, pooled, dried and frozen at −80 °C for 24 h. Those fragments were further reduced in a ball-mill (MM401, Retsch, Newtown, USA) at 30 Hz for 7 min. The resultant powder was sieved (#400 mesh) and three aliquots of 1 g each were treated with phosphoric acid (PA) solutions in different concentrations: 1% (pH 1.4), 10% (pH 1.0) and 37% (pH 0.4) for 10 min, 1 min or 20 s, respectively ( Fig. 1 ). This variation of time application was chosen in order to allow a proportional demineralization of dentin between testing solutions. Thus, since 10% and 37% PA solutions are, respectively, 10 and 37 times more concentrated than the 1% solution, their time of application were, accordingly, reduced 10- and 37-fold. To neutralize the acid demineralization of dentin, 4 M NaOH was added in volumes that varied from 0.2 to 8.6 mL to complete react with PA. Protein extraction from demineralized dentin powder was performed using the protocol described by Breschi et al. (2010). Briefly, the samples were re-suspended in 4 mL extraction buffer (50 mM Tris-HCl containing 5 mM CaCl 2 , 100 mM NaCl, 0.1% Triton X-100, 0.1% NONIDET P-40, 0.1 mM ZnCl 2 , 0.02% NaN 3 ) for 24 h at 4 °C under stirring. Then, they were centrifuged, the supernatants were collected and protein content was precipitated with 25 wt% trichloroacetic acid (TCA) at 4 °C. TCA precipitates were re-solubilized in 1.5 M Tris–HCl (pH 8.8). Aliquots of mineralized dentin powder were used as negative controls; they were processed as the other samples with the exception they were not treated with phosphoric acid, but instead remained mineralized and stored in extraction buffer. Total protein concentration was determined by the Lowry et al. method.
2.2
Measurement of calcium released from mineralized dentin
The neutralized supernatants obtained after demineralization of dentin powder were used for determination of calcium content ( Fig. 1 ). 1-mL aliquots of each supernatant (1%, 10% and 37% PA) were transferred to plastic vials containing 0.5 mL of 0.1% chloranilic acid and kept undisturbed for 30 min. The samples were centrifuged (for 30 min at 1200 rpm – rotor Sorvall SM-24), the respective supernatants were discarded and pellets were re-suspended in 3 mL of 50% isopropyl alcohol. Further centrifugation was performed (for 30 min at 1200 rpm − rotor Sorvall SM-24), the supernatant was again discarded and 3 mL of 5% EDTA was added to the resultant precipitate . The same procedures were performed for the supernatant (extraction buffer) of negative controls (mineralized dentin powder). All samples were analyzed against a standard curve of known concentrations of calcium (0.25, 0.50, 0.75, 1.00 mg/mL) in a spectrophotometer (Beckman Coulter DU-800, California, USA) at 520 nm wavelength. The same Experiments were run in triplicates.
2.3
Measurement of collagen solubilization (Hydroxyproline assay)
Precipitates obtained after the protein extraction were lyophilized and separated into 10 aliquots (40 mg each) of each experimental condition (1%, 10% or 37% PA) ( Fig. 1 ). Samples were incubated in 1 mL of artificial saliva (AS) (50 mmol/L HEPES, 5 mmol/L CaCl 2 , 0.001 mmol/L ZnCl 2 , 150 mmol/L NaCl, 0.3 mmol/L PMSF, 3 mmol/L NaN 3 ) at 37 °C for 24 h under stirring. After incubation, the AS storage medium was completely removed and analyzed for dentin collagen solubilization by measuring the amount of hydroxyproline (HYP) released. The same procedures were performed for the negative controls (mineralized dentin powder). Measurement of HYP was determined using a colorimetry assay, slightly modified from that described by Reddy & Enwemeka . Briefly, 500 μL of supernatant was lyophilized, re-suspended in 10 μL deionized water and 40 μL of 2 N NaOH was added and were hydrolyzed by autoclaving (at 120 °C) in two cycles of 45 min each. Then, samples’ oxidation was performed with chloramine-T for 25 min and the chromophore was developed by incubation with Ehrlich’s reagent for 40 min at 65 °C. Absorbance values were measured in a spectrophotometer (Beckman Coulter DU-800, USA) against standard curves of known concentrations of HYP (2, 5, 10, 15 and 25 μg/mL) and the solubilized collagen was expressed as micrograms of HYP per milligram of demineralized dentin (μg/mg dentin).
2.4
Western blot
To confirm the immunological identity of MMP-2 in the extracts, the supernatant obtained after protein extraction (80 μg of protein) were electrophorezed on 10% SDS-PAGE gels ( Fig. 1 ) under reducing conditions and proteins were transferred with a semi-dry protocol (Trans-Blot, Bio-Rad, California, USA) to nitrocellulose membranes (Bio-Rad, California, USA). After washing with TBS–Tween-20, the membranes were incubated with primary antibody, overnight at 4 °C. Polyclonal primary antibody was rabbit anti-human MMP-2 (Abcam Inc., Massachusetts, USA) diluted (1:500) in 0.01% PBS Tween-20 buffer. Following washings, membranes were incubated with goat polyclonal secondary antibodies (Abcam Inc., Massachusetts, USA) (1:10,000) for 1 h at room temperature. Immunoreactive bands were detected by chemiluminescent reagents (ECL, Abcam Inc., Massachusetts, USA) on radiographic films (GE, England) and evaluated by densitometry with image analysis program (Scion Image, www.scioncorp.org).
2.5
Conventional gelatin zymography
Precipitates obtained after the protein extraction (80 μg) were electrophorized ( Fig. 1 ) under non-reduced conditions on 10% SDS-polyacrylamide gel containing 1 mg/mL fluorescent gelatin, which was previously labeled with MDPF (2-Methoxy-2,4-diphenyl-3(2H)-furanone) . Enzymes activation was achieved with 2 mM p -aminophenylmercuric acetate (APMA) for 1 h at 37 °C. After electrophoresis, the gels were washed for 1 h in 2% Triton X-100 and were then incubated in activation solution (50 mM Tris-HCl, 5 mM CaCl 2 pH 7.4) for 24 h. Negative control gels were incubated with the same buffer containing 2 mM of 1,10-phenanthroline. After incubation, the zymograms were photographed under UV illumination (Quantity One, BioRad, California, USA) and evaluated by densitometry using an image analysis program (Scion Image, www.scioncorp.org). Experiments were run in triplicates.
2.6
In situ zymography
Mid-coronal dentin slices (0.3 mm) were acid-etched with the tested PA solutions (1%, 10% and 37%) under conditions previously described. In situ zymography was performed using a quenched fluorescein-conjugated gelatin as substrate (E-12055, Molecular Probes, Eugene, USA), as described by Mazzoni et al. . 80-μL of that gelatin substrate were placed on top of each dentin slab and incubated in a dark humid chamber at 37 °C for 24 h. The hydrolysis of quenched fluorescein-conjugated gelatin was assessed by a multi-photon confocal microscope, with an excitation wavelength of 488 nm and emission wavelength of 530 nm (Zeiss, LSM 780, Carl Zeiss, Oberkochen, Germany). Optical sections of 85 μm thick were acquired and the stacked images were analysed, quantified, and processed with ZEN 2010 software (Carl Zeiss).
2.7
Statistical analysis
Results were analyzed by ANOVA and Student-Newman-Keuls test for multiple comparisons (α = 0.05) for western blot and conventional zymograph and Kruskal-Wallis and Student-Newman-Keuls test (α = 0.05) for calcium and HYP release.
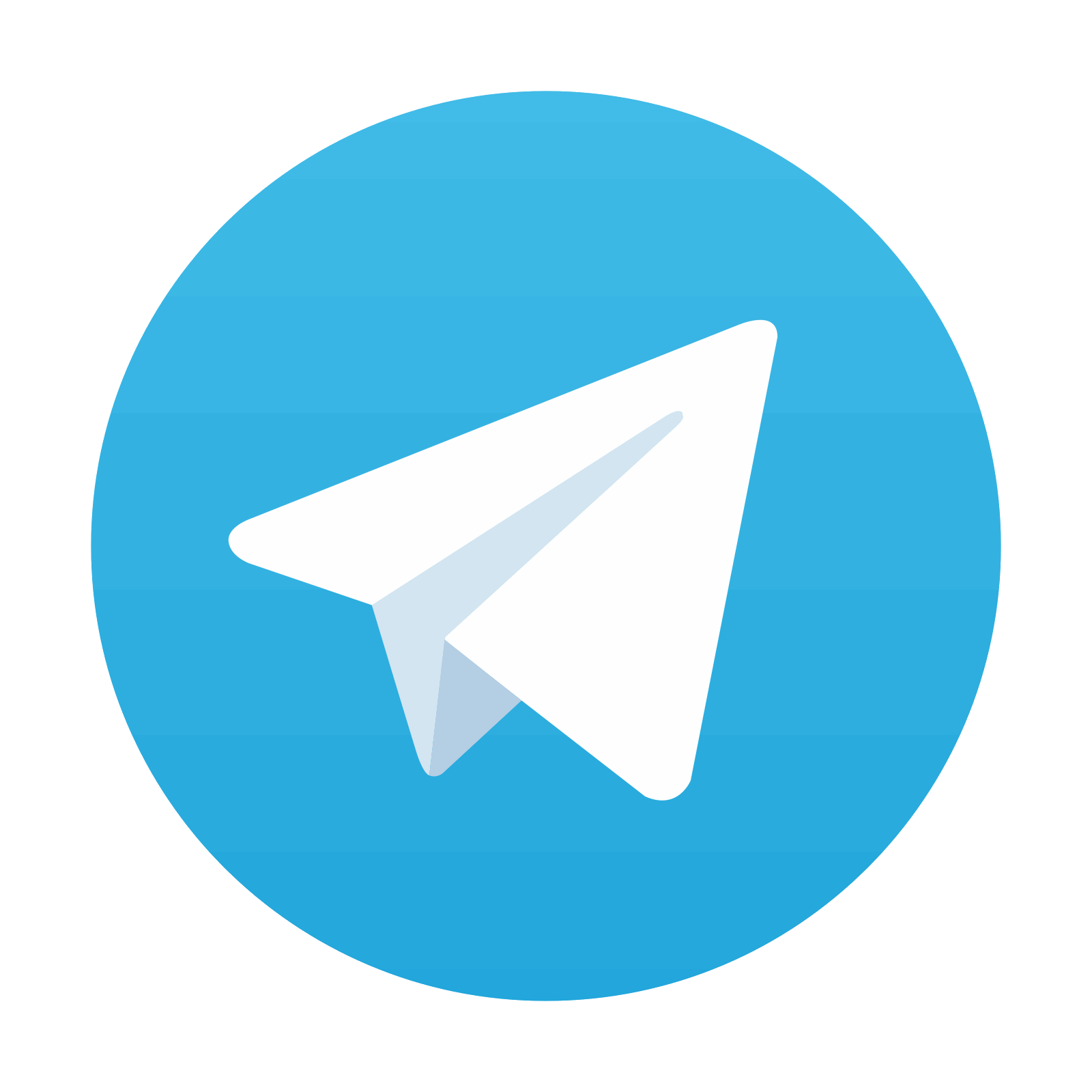
Stay updated, free dental videos. Join our Telegram channel

VIDEdental - Online dental courses
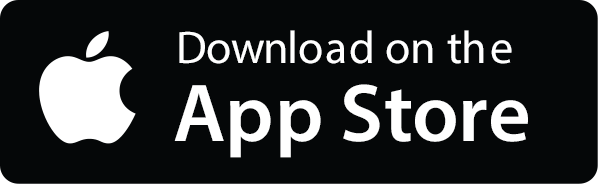
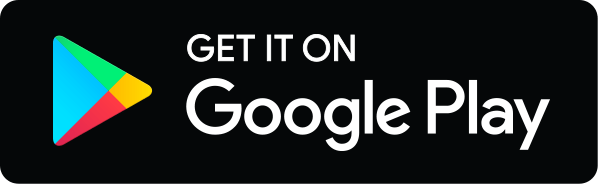