Abstract
Objective
The goal of this work is to investigate t–m phase transformation, and subsurface damage in 3Y-TZP after sandblasting.
Methods
Commercial grade 3Y-TZP powder was conventionally sintered and fully dense specimens were obtained. Specimens were sandblasted using different particle sizes (110 and 250 μm) and pressures (2 and 4 bar) for 10 s. Phase transformation was measured on the surface and in the cross-section using X-ray diffraction and micro Raman spectroscopy, respectively. Subsurface damage was investigated on cross-sections using SEM and in shallow cross-sections machined by focused ion beam.
Results
Sandblasting induced monoclinic volume fraction is in the range of 12–15% on the surface. In the cross-section, a non-homogeneous phase transformation gradient is found up to the depth of 12 ± 1 μm. The subsurface damage observed was plastic deformation in grains with the presence of martensite plates, and this effect is found to be larger in specimens sandblasted with large particles.
Significance
The extent of subsurface tetragonal–monoclinic transformation and damage induced by sandblasting are reported for different sandblasting conditions. This knowledge is critical in order to understand the effect of sandblasting on mechanical properties of zirconia used to fabricate dental crowns and frameworks.
1
Introduction
Due to the high esthetic demand of dental restorations, metal systems are now progressively being replaced by all-ceramic systems. Initially, in the 1960s feldspathic porcelain was used to make dental crowns, but now other ceramics such as alumina and tetragonal zirconia doped with 3 mol% of yttria (3Y-TZP) have made inroads into the field of restorative dentistry. In particular, the use of zirconia based ceramics in restorative dentistry has increased in the last decades and are currently employed in applications such as core for crowns, orthodontic brackets, fixed partial denture prosthesis (FPDP) frameworks and endodontic posts .
One of the main reasons for the interest of the dental community in 3Y-TZP is because it has the highest strength among ceramic oxides . This is related to their very small grain size and to the expansion that accompanies tetragonal–monoclinic (t–m) transformation in front of a propagating crack.
The metastable tetragonal zirconia is also prone to t–m transformation under the stress generated by grinding and sandblasting, and both methods are commonly used in dentistry for machining or final surface preparation. In particular, sandblasting is mainly used to roughen the intaglio surface of the zirconia crowns to achieve improved bonding between the dental crown and the luting cement, and, in some cases, for better adhesion between the crown and the veneering porcelain .
The t–m surface transformation generated during sandblasting is accompanied by compressive residual stresses and sometimes by surface cracks . It is not clear under which sandblasting conditions the residual compressive stresses may counteract the influence of sandblasting cracks. This information is important for the dental community as well as the material scientists to assess the long-term performance and to prevent clinical failures.
Kosmac et al. , Sato et al. and Zhang et al. have previously studied various aspects of sandblasting of dental zirconia ceramics such as phase transformation, bending strength and long term performance. Only few authors have studied the depth of the phase transformation in 3Y-TZP after sandblasting, however its direct measurement has not been reported previously.
It is obvious that the damage induced by sandblasting could have adverse consequences on the performance and reliability of 3Y-TZP. Scanning electron microscopy (SEM) examinations by Kosmac et al. on the sandblasted specimens revealed lateral crack chipping, which is the most prevalent mechanism of damage. Similarly, Guazzato et al. reported that the impact of sand particles on the surface of Y-TZP caused significant damage due to extensive erosive wear and lateral cracks. Zhang et al. emphasized that the examinations made by SEM may not resolve any individual microcracks, especially in microstructures with submicrometer grain sizes. Nevertheless, as the reduction in elastic modulus is related to the presence of micro cracks , nanoindentation measurements within the damage zones may detect their existence .
Although the effect of sandblasting has been studied previously , details on the phase transformation and subsurface damage are limited. The objective of this paper is focused on the effect of sandblasting on near surface phase transformation and damage in 3Y-TZP. The microstructure and properties of the near surface zone are crucial for the dental crowns and implants for good bonding with the other surface in contact and for long-term performance of the union.
2
Materials and methods
Commercial 3Y-TZP powder (TZ-3YSB-E Tosoh Co., Japan) was cold isostatically compacted under pressure of 200 MPa in a cylindrical mold for producing a green body, which was later sintered in an alumina tube furnace at 1450 °C for 2 h (3 °C/min heating and cooling rates). The sintered ceramic cylinder was cut into specimens in the form of disks (2 mm thick, 10 mm diam.), which were polished up to colloidal silica finishing. These will be referred as the “control” specimens. The average grain size was 0.3 ± 0.1 μm, the grain size measurement details were reported in our previous work . The bulk density was over 99% of the theoretical value as measured by the Archimedes method.
Sandblasting was carried out with the alumina particles using two particle sizes (110 and 250 μm), two pressures (2 and 4 bar), at a working distance of 25 mm and always perpendicularly to the surface of the specimens using a sandblaster (BEGO, Germany). The details of the sandblasting conditions together with the codes used for the specimens are shown in Table 1 .
Treatments | Description |
---|---|
Control | Polished |
110-2B | Sandblasted with 110 μm particles at 2 bar pressure |
110-4B | Sandblasted with 110 μm particles at 4 bar pressure |
250-2B | Sandblasted with 250 μm particles at 2 bar pressure |
250-4B | Sandblasted with 250 μm particles at 4 bar pressure |
The surface roughness of the disk specimens was analyzed after polishing and after sandblasting by means of a surface profilometer (SURFTEST) coupled with SURFPAK software. They were also analyzed for phase composition by X-ray diffraction (Bruker AXS D8). A Bragg–Brentano configuration was used in θ –2 θ mode. Spectra were obtained for 2 θ between 24° and 37°, with an increment of 0.02° and a velocity of one second per step. Cu K α with wavelength λ = 1.5418 Å was used as the radiation source. Voltage and current was set to 40 kV and 40 mA. The penetration depth of X-rays is ~3 μm.
The phase analysis of the samples was done using the equation of Toraya et al. . Tetragonal and monoclinic phases are quantified considering the diffraction peaks between 24° and 37°, as it is mostly done in the literature using the following equation.
X m = 1.311 ( I m 〈 1 ¯ 1 1 〉 + I m 〈 1 1 1 〉 ) 1.311 ( I m 〈 1 ¯ 1 1 〉 + I m 〈 1 1 1 〉 ) + I t 〈 1 1 1 〉
I m <SPAN role=presentation tabIndex=0 id=MathJax-Element-2-Frame class=MathJax style="POSITION: relative" data-mathml='(1¯11)’>(1¯11)(1¯11)
( 1 ¯ 1 1 )
and I m (1 1 1) are the monoclinic peak intensities at 2θ equal to 28.2° and 31.4°, respectively, and I t (1 1 1) is the tetragonal peak intensity at 31.1°. Integrated peak areas were obtained to calculate the monoclinic volume fraction.
Micro Raman spectroscopy was used to quantify the local phase transformation in the cross-sections of the sandblasted samples. A triple monochromator spectrometer (Horiba JobinYvon – LABRAM HR) with a coupled CCD detector (liquid nitrogen cooled) was used to obtain Raman spectra on the residual indentation imprints. An Ar-ion laser Innova 300 (Coherent Laser Group) was used as a light source and excited at a wavelength of 514 nm. Raman spectra were collected at 100× magnification and the spectrum integration time was 60 s, with the recorded spectra averaged over four successive measurements.
Each sandblasted sample was cut into two symmetrical pieces and the cross-section of one piece was finely polished. Micro Raman spectra were collected by mapping an area of 140 μm 2 . Two maps were acquired from the cross-section at positions in the range of 1 mm distance from the center of the sample with a lateral resolution of 2 μm. The phase quantification was done by using the equation proposed by Katagiri et al. :
V m = 0.5 ( I m 180 + I m 190 ) 2.2 I t 150 + 0.5 ( I m 180 + I m 190 )
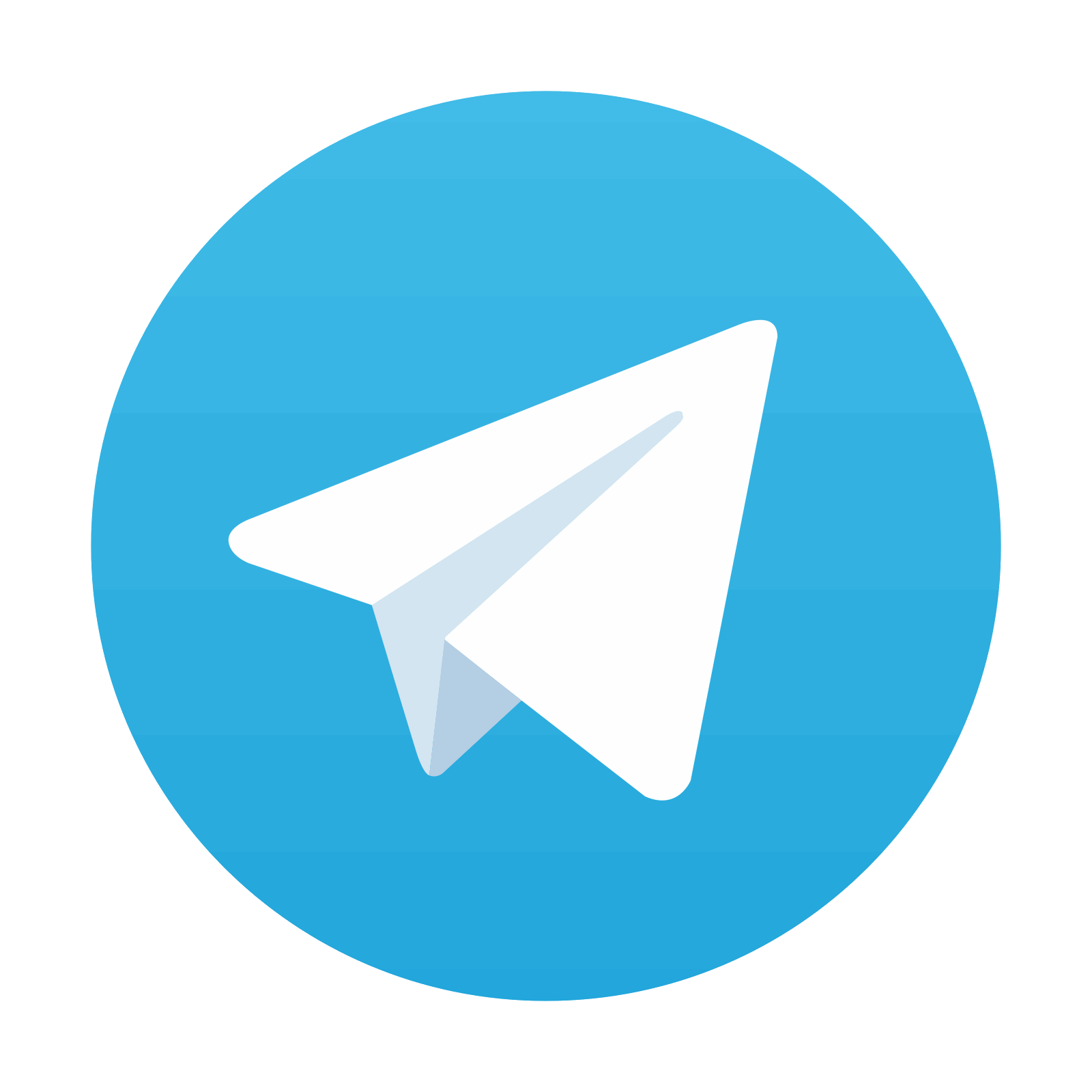
Stay updated, free dental videos. Join our Telegram channel

VIDEdental - Online dental courses
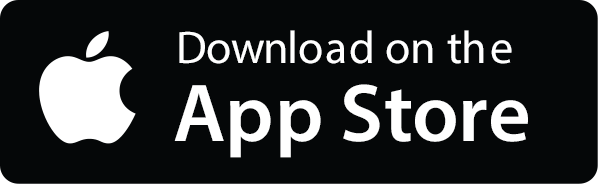
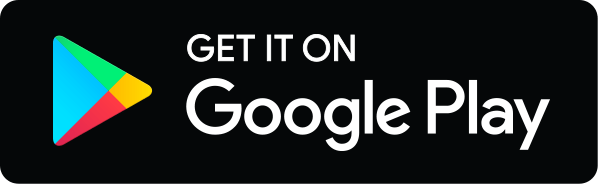