Highlights
- •
Formation of dentin bonding interfaces under simulated pulpal pressure.
- •
The role of intrinsic water permeability in the formation of dentin bonds.
- •
Effects of thermo-mechanical loading on resin–dentin bond degradation.
- •
Ethanol-wet bonding helps to compensate for water permeation from dentin tubules.
Abstract
Objectives
The aim of this study was to evaluate the formation of dentin bonding interfaces using the water-wet and the ethanol-wet techniques under simulated pulpal pressure, and to assess the effect of adhesive solvent and thermomechanical loading.
Methods
Flat dentin surfaces were restored under 20 mm-simulated pulpal pressure following two bonding approaches (water-wet and ethanol-wet bonding) in combination with dental adhesives containing ethanol (Single Bond Plus and Scotchbond Multi-Purpose) or acetone (One-Step Plus and All-Bond 2) as solvent. Half of the restorations of each subgroup were subjected to thermocycling followed by cyclic loading (three teeth per group). Bond strength was measured using the microtensile bond strength test and fitted to a Weibull distribution ( α = 0.05). Ultrastructural analyses of the interface and leakage/nanoleakage evaluation were performed using confocal scanning microscopy (CLSM) and transmission electron microscopy (TEM).
Results
Water permeation through dentin tubules during adhesive application prevented adequate penetration of adhesive monomers into the demineralized collagen matrix in both bonding techniques, but more severely for water-wet bonding. Acetone-solvated adhesives showed worse bonding performance and hybridization than ethanol-based systems when applied in the ethanol-wet mode, both before and after thermomechanical challenge.
Significance
The ethanol-wet bonding technique helps to compensate for water permeation from dentin tubules during the bonding procedures to form more stable dentin bonds, especially when used in conjunction to ethanol-solvated systems.
1
Introduction
Water is a polar solvent that plays a polar role in dentin bonding. Water is needed to prevent the collapse of the etched, hydroxyapatite-depleted collagen network, serving as a medium for subsequent infiltration of solvated hydrophilic monomer mixtures . This approach has shaped the concept of dentin adhesion and seems to persevere as a successful clinical technique . Water therefore helps to achieve high quality bonds to dentin – immediate quality. Long-term degradation of dentin bonds, on the other hand, is generally associated to processes linked to the presence of water . To name some, water promotes the phase separation of hydrophobic monomers and creates a comonomer gradient within the hybrid layer , where the low-molecular weight, more hydrophilic monomers occupy its deepest regions retaining humidity, and soaking further water from the substrate. Water during application interferes with monomer polymerization, and trapped and absorbed water later flow through uncured routes within the polymer to hydrolyze and plasticize the resin ; chemical degradation proceeds invariably to mechanical degradation of the interface. Parallel to that, interfibrillar water mediates the activity of endogenous enzymes (endopeptidases) set loose during etching, triggering the proteolytic degradation of unenveloped collagen fibers in the hybrid layer, reducing its long-term structural integrity .
To circumvent these issues some current strategies aimed to prolong the integrity of the dentin bonding interface bet on the complete replacement of water from the etched surface with ethanol before infiltration with resin . Saturation with absolute ethanol promotes the full dehydration and shrinkage of collagen fibers, and in principle grants the use of more hydrophobic monomer solutions that are less prone to water uptake and hydrolysis. So far this rationale was proven right in vitro , in that hydrophobic bonding to dentin following the so-called ethanol-wet bonding technique has shown longstanding ultrastructural integrity and bond strength in comparison to its hydrophilic water-wet technique counterpart . Accountable for these improvements are, essentially, the deeper penetration of hydrophobic monomers that increase the mechanical quality of the hybrid layer in its entire depth , the minimal permeation from substrate moisture into the interface and the removal of unbound water from interfibrillar spaces prior to application of adhesive monomers . However, bound water in dentin matrices cannot be evaporated or removed by organic solvents such as ethanol .
Yet in vital teeth dentin permeability increases considerably after etching, and pulpal fluid is continuously pumped toward the interface during adhesive application and after polymerization. Pulpal pressure places water at the interface unless dentin tubules are occluded, potentially undermining the aforementioned benefits of a hydrophobic seal. Also playing against the clinical feasibility of the ethanol-wet technique is the technical sensitivity of the dehydration procedure , the prolonged clinical time for proper dehydration to take place, and the immense variability in composition of available commercial adhesive systems.
Motivated by these open issues the present study was set to evaluate the role of intrinsic water permeability in the formation of dentin bonds following the ethanol and water-wet techniques using commercial adhesives having different compositions and solvents. Our null hypotheses were: (1) that bond integrity in terms of interface morphology and bond strength are comparable for bonds produced by the ethanol-wet and water-wet techniques; (2) that adhesive system and solvent (ethanol or acetone) have no significant effect on bond integrity assessed by microscopy, and; (3) that thermo-mechanical loading results in no bond degradation irrespective of adhesive system or technique employed.
2
Materials and methods
One hundred and twelve intact, freshly extracted, non-carious human third molars were collected after the donors’ informed consent and under a protocol reviewed and approved by an Ethical Committee. The teeth were scaled, cleaned, stored in 0.5% chloramine solution at 4 °C to prevent bacteria growth and used within 3 months after extraction. The teeth were sectioned perpendicular to the longitudinal axis of the tooth 3.5 mm below the cement-enamel junction (CEJ), using a diamond saw under water-cooling. A second parallel cut removed the occlusal enamel and superficial dentin creating a flat dentin surface of middle dentin A caliper was used to control the remaining dentin thickness to 2.5 ± 0.1 mm from the deepest pulpal horn. The pulpal tissue was gently removed from the exposed pulp chamber without damaging the pre-dentin. The occlusal surfaces of crown segments were polished using a 600-grit silicon carbide paper under running water for 60 s to create a standard smear layer. The crown segments were attached to perforated plexiglass disks and connected to polyethylene 18-gauge tubings to simulate pulpal pressure. Twenty centimeters of water pressure was delivered to the prepared dentin surface during the adhesive procedures and restoration build-up.
The teeth were divided into two main groups according to the bonding technique: water-wet (W) or ethanol-wet (E) bonding. Adhesive systems were selected (see Table 1 ) based on their solvent (ethanol or acetone) and number of application steps (two-step or three-step etch-and-rinse systems). The exposed occlusal dentin surface was etched with 35% phosphoric acid gel (Scotchbond Etchant, 3M ESPE) for 15 s and rinsed with distilled water for 15 s. The excess of water was blotted with a tissue but left visibly moist to prevent collapse of the collagen matrix. In the water-wet bonding group the primers/adhesives were applied to the moist dentin surface, while in the ethanol-wet bonding groups the surface was saturated with 1 ml of 100% ethanol for 30 s before primer/adhesive application.
Adhesive system | Chemical composition | Application mode | Batch number |
---|---|---|---|
Scotchbond Etchant (3M ESPE) | 35% H 3 PO 4 | – Acid-etch for 15 s – Rinse for 15 s – Blot excess water using absorbent paper |
9NA |
Adper Single Bond Plus (3M ESPE) | Adhesive : dimethacrylates, HEMA, polyalkenoid acid copolymer, colloidal silica, ethanol, water, photo-initiator | – Apply two consecutive coats of adhesive for 15 s with gentle agitation – Gently blow air for 5 s at 10 cm to evaporate solvent – Light-cure for 10 s |
8UK |
Adper Scotchbond Multi-Purpose (3M ESPE) | Primer : HEMA, polyalkenoic acid polymer, water Adhesive : Bis-GMA, HEMA, tertiary amines, photo-initiator |
– Apply one coat of primer – Gently blow air for 5 s at 10 cm to evaporate solvent – Apply one coat of adhesive – Light-cure for 10 s |
Primer: 9CA Adhesive: 9RK |
One-Step Plus (Bisco) | Adhesive : BPDM, Bis-GMA, HEMA, acetone, photo-initiator, 8.5 wt% fluoroaluminosilicate glass fillers | – Apply two consecutive coats of adhesive for 15 s with gentle agitation – Gently blow air for 5 s at 10 cm to evaporate solvent – Light-cure for 10 s |
1000004239 |
All-Bond 2 (Bisco) | Primer A : NTG-GMA, acetone, ethanol, water Primer B : BPDM, photo-initiator, acetone D/E Resin : Bis-GMA, UDMA, HEMA, photo-initiator |
– Dispense and mix equal amounts of Primer A and B – Apply five consecutive coats of mixed primers – Gently blow air for 5 s at 10 cm to evaporate solvent – Apply a thin layer of D/E Resin – Light-cure for 20 s |
Primer A: 1000003169 Primer B: 1000003170 D/E Resin: 1000002247 |
The adhesive systems were applied to the etched dentin strictly following the manufacturers’ instructions in regard to application layers and time (see Table 1 ). Light-curing was performed using a halogen unit (Elipar 2500, 3M ESPE) with 600 mW/cm 2 light output. Resin composite restorations made with a micro-hybrid composite resin (Filtek Z250, shade A2, 3M ESPE) were built on top of the bonded surfaces in five successive increments of 1 mm, each light-cured for 20 s. From etching until this point the teeth were under 20 cm simulated water pressure.
After conclusion of restoration build-up the teeth were removed from the pulpal simulator apparatus (the pulpal chamber was left open) and for each adhesive system half ( n = 4) of the teeth were stored in distilled water for 24 h, while the other half were subjected to a thermo-mechanical challenge before testing for microtensile bond strength (μTBS). The thermo-mechanical challenge was performed by means of 20,000 thermal cycles at 5 °C and 55 °C (30 s dwell time, 10 s transfer time) (THE-1100, SD Mechatronik, Germany) followed by 600,000 cycles of mechanical loading using a 5 mm-radius flat metallic antagonist under 40 N load in a chewing simulator (CS-3.8, SD Mechatronik) in water at 37 °C.
For bond strength testing each tooth/restoration was sectioned perpendicular to the adhesive interface in x – y directions according to the non-trimming technique using a diamond saw under water-cooling to create rectangular beams with a cross-sectional area of 0.7 ± 0.1 mm 2 . The exact dimensions of the cross-sectional area of each beam were measured using a digital caliper with an accuracy of 0.001 μm. For testing, each beam (specimen) was individually attached to a stainless steel notched Geraldeli’s jig using cyanoacrylate adhesive (Zapit, Dental Ventures of North America, Corona, CA, USA). The jigs with specimens were stressed under uniaxial tension until failure in a universal testing machine (Model 1125, Instron, Inc., Canton, MA, USA) using a load cell of 100 N at a crosshead speed of 1 mm/min. Specimens that failed before testing (during cutting or mounting) were designated as pre-testing failures (PTF).
A uniform distribution of defects results in a strength variability in brittle materials/interfaces that can be statistically treated by fitting the data to a Weibull distribution, describing the failure probability P F as P F = 1 − exp[−( σ / σ 0 ) m ]. For each set of specimens the Weibull shape parameter m and characteristic strength parameter σ 0 were determined in a ln σ − ln(1/(1 − P F )) plot . The slope of the regression describes the scatter in strength and gives the m value from ln(ln 1/(1 − P F )) = m ln σ − m ln σ 0 , and σ 0 represents the strength at a failure probability of 63.2% when ln(ln 1/(1 − P F )) = 0. Based on a mean value of the weakest specimens in all groups, a value of 5 MPa was assigned to all PTFs, which were treated as left-censored data using the Maximum Likelihood Estimation (MLE) procedure. The MLE method incorporates the contribution of both uncensored and left-censored data to the likelihood function of the distribution. The 95% confidence intervals were calculated and differences were considered to be significant when they did not overlap.
For microstructural analysis of the adhesive interfaces, three-dimensional images were generated using a confocal laser scanning microscope (FV1000 Fluoview, Olympus, Japan). For that, three drops of each component of the adhesive systems were mixed with one grain of tetramethylrhodamine B isothiocyanate (Sigma–Aldrich, St. Louis, MO, USA), creating a homogeneous solution, and the adhesive procedures were performed just as described above. After removal from the pulpal pressure simulation device, the pulpal chambers of the teeth were filled with 0.1% fluorescein sodium solution (Sigma–Aldrich) for 4 h. Two extra teeth for each bonding technique and adhesive were produced without simulated pulpal pressure for comparison. Two slabs with 1.0 mm thickness were removed from the center of each tooth and fluorescence images of the adhesive interface were generated using both a helium-neon gas laser and an argon-ion laser. The fluorescent light was conducted through a filter-based fluorescence detection system, discriminating it from reflected and scattered light to obtain images of the resin–dentin interface with a magnification of 100 times at 1024 × 1024 pixels and 2.0 μs/pixel speed.
Two resin-bonded dentin slabs from each group were further processed for transmission electron microscopy (TEM). The slabs were coated with nail varnish, leaving 1 mm around the bonding interface exposed. Specimens were rehydrated with distilled water for 20 min and then immersed in an aqueous solution of 50% ammoniacal silver nitrate dye (pH 9.5) for 24 h to trace voids and porosity within the hybrid layer. The slabs were then placed into a photo-developing solution for 8 h under a fluorescent light to permit the reduction of the diamine silver ions into metallic silver grains, followed by washing under running water for 1 min and removal of the nail polish. For fixation, the slabs were immersed in 2.5% formaldehyde/glutaraldehyde in 0.1 M sodium cacodylate buffer at pH 7.4 for 12 h at 4 °C, rinsed four times with 20 mL of 0.2 M sodium cacodylate buffer at pH 7.4 for a total of 1 h, followed by distilled water bath for 1 min. The slabs were dehydrated in ascending concentrations of ethanol, embedded in epoxy resin, and sectioned with an ultramicrotome to obtain mineralized sections of 90–100 nm of thickness. The characteristics of nanoleakage at the resin–dentin interfaces were examined using a transmission electron microscope (H-8100, Hitachi, Tokyo, Japan) at an accelerating voltage of 100 kV.
2
Materials and methods
One hundred and twelve intact, freshly extracted, non-carious human third molars were collected after the donors’ informed consent and under a protocol reviewed and approved by an Ethical Committee. The teeth were scaled, cleaned, stored in 0.5% chloramine solution at 4 °C to prevent bacteria growth and used within 3 months after extraction. The teeth were sectioned perpendicular to the longitudinal axis of the tooth 3.5 mm below the cement-enamel junction (CEJ), using a diamond saw under water-cooling. A second parallel cut removed the occlusal enamel and superficial dentin creating a flat dentin surface of middle dentin A caliper was used to control the remaining dentin thickness to 2.5 ± 0.1 mm from the deepest pulpal horn. The pulpal tissue was gently removed from the exposed pulp chamber without damaging the pre-dentin. The occlusal surfaces of crown segments were polished using a 600-grit silicon carbide paper under running water for 60 s to create a standard smear layer. The crown segments were attached to perforated plexiglass disks and connected to polyethylene 18-gauge tubings to simulate pulpal pressure. Twenty centimeters of water pressure was delivered to the prepared dentin surface during the adhesive procedures and restoration build-up.
The teeth were divided into two main groups according to the bonding technique: water-wet (W) or ethanol-wet (E) bonding. Adhesive systems were selected (see Table 1 ) based on their solvent (ethanol or acetone) and number of application steps (two-step or three-step etch-and-rinse systems). The exposed occlusal dentin surface was etched with 35% phosphoric acid gel (Scotchbond Etchant, 3M ESPE) for 15 s and rinsed with distilled water for 15 s. The excess of water was blotted with a tissue but left visibly moist to prevent collapse of the collagen matrix. In the water-wet bonding group the primers/adhesives were applied to the moist dentin surface, while in the ethanol-wet bonding groups the surface was saturated with 1 ml of 100% ethanol for 30 s before primer/adhesive application.
Adhesive system | Chemical composition | Application mode | Batch number |
---|---|---|---|
Scotchbond Etchant (3M ESPE) | 35% H 3 PO 4 | – Acid-etch for 15 s – Rinse for 15 s – Blot excess water using absorbent paper |
9NA |
Adper Single Bond Plus (3M ESPE) | Adhesive : dimethacrylates, HEMA, polyalkenoid acid copolymer, colloidal silica, ethanol, water, photo-initiator | – Apply two consecutive coats of adhesive for 15 s with gentle agitation – Gently blow air for 5 s at 10 cm to evaporate solvent – Light-cure for 10 s |
8UK |
Adper Scotchbond Multi-Purpose (3M ESPE) | Primer : HEMA, polyalkenoic acid polymer, water Adhesive : Bis-GMA, HEMA, tertiary amines, photo-initiator |
– Apply one coat of primer – Gently blow air for 5 s at 10 cm to evaporate solvent – Apply one coat of adhesive – Light-cure for 10 s |
Primer: 9CA Adhesive: 9RK |
One-Step Plus (Bisco) | Adhesive : BPDM, Bis-GMA, HEMA, acetone, photo-initiator, 8.5 wt% fluoroaluminosilicate glass fillers | – Apply two consecutive coats of adhesive for 15 s with gentle agitation – Gently blow air for 5 s at 10 cm to evaporate solvent – Light-cure for 10 s |
1000004239 |
All-Bond 2 (Bisco) | Primer A : NTG-GMA, acetone, ethanol, water Primer B : BPDM, photo-initiator, acetone D/E Resin : Bis-GMA, UDMA, HEMA, photo-initiator |
– Dispense and mix equal amounts of Primer A and B – Apply five consecutive coats of mixed primers – Gently blow air for 5 s at 10 cm to evaporate solvent – Apply a thin layer of D/E Resin – Light-cure for 20 s |
Primer A: 1000003169 Primer B: 1000003170 D/E Resin: 1000002247 |
The adhesive systems were applied to the etched dentin strictly following the manufacturers’ instructions in regard to application layers and time (see Table 1 ). Light-curing was performed using a halogen unit (Elipar 2500, 3M ESPE) with 600 mW/cm 2 light output. Resin composite restorations made with a micro-hybrid composite resin (Filtek Z250, shade A2, 3M ESPE) were built on top of the bonded surfaces in five successive increments of 1 mm, each light-cured for 20 s. From etching until this point the teeth were under 20 cm simulated water pressure.
After conclusion of restoration build-up the teeth were removed from the pulpal simulator apparatus (the pulpal chamber was left open) and for each adhesive system half ( n = 4) of the teeth were stored in distilled water for 24 h, while the other half were subjected to a thermo-mechanical challenge before testing for microtensile bond strength (μTBS). The thermo-mechanical challenge was performed by means of 20,000 thermal cycles at 5 °C and 55 °C (30 s dwell time, 10 s transfer time) (THE-1100, SD Mechatronik, Germany) followed by 600,000 cycles of mechanical loading using a 5 mm-radius flat metallic antagonist under 40 N load in a chewing simulator (CS-3.8, SD Mechatronik) in water at 37 °C.
For bond strength testing each tooth/restoration was sectioned perpendicular to the adhesive interface in x – y directions according to the non-trimming technique using a diamond saw under water-cooling to create rectangular beams with a cross-sectional area of 0.7 ± 0.1 mm 2 . The exact dimensions of the cross-sectional area of each beam were measured using a digital caliper with an accuracy of 0.001 μm. For testing, each beam (specimen) was individually attached to a stainless steel notched Geraldeli’s jig using cyanoacrylate adhesive (Zapit, Dental Ventures of North America, Corona, CA, USA). The jigs with specimens were stressed under uniaxial tension until failure in a universal testing machine (Model 1125, Instron, Inc., Canton, MA, USA) using a load cell of 100 N at a crosshead speed of 1 mm/min. Specimens that failed before testing (during cutting or mounting) were designated as pre-testing failures (PTF).
A uniform distribution of defects results in a strength variability in brittle materials/interfaces that can be statistically treated by fitting the data to a Weibull distribution, describing the failure probability P F as P F = 1 − exp[−( σ / σ 0 ) m ]. For each set of specimens the Weibull shape parameter m and characteristic strength parameter σ 0 were determined in a ln σ − ln(1/(1 − P F )) plot . The slope of the regression describes the scatter in strength and gives the m value from ln(ln 1/(1 − P F )) = m ln σ − m ln σ 0 , and σ 0 represents the strength at a failure probability of 63.2% when ln(ln 1/(1 − P F )) = 0. Based on a mean value of the weakest specimens in all groups, a value of 5 MPa was assigned to all PTFs, which were treated as left-censored data using the Maximum Likelihood Estimation (MLE) procedure. The MLE method incorporates the contribution of both uncensored and left-censored data to the likelihood function of the distribution. The 95% confidence intervals were calculated and differences were considered to be significant when they did not overlap.
For microstructural analysis of the adhesive interfaces, three-dimensional images were generated using a confocal laser scanning microscope (FV1000 Fluoview, Olympus, Japan). For that, three drops of each component of the adhesive systems were mixed with one grain of tetramethylrhodamine B isothiocyanate (Sigma–Aldrich, St. Louis, MO, USA), creating a homogeneous solution, and the adhesive procedures were performed just as described above. After removal from the pulpal pressure simulation device, the pulpal chambers of the teeth were filled with 0.1% fluorescein sodium solution (Sigma–Aldrich) for 4 h. Two extra teeth for each bonding technique and adhesive were produced without simulated pulpal pressure for comparison. Two slabs with 1.0 mm thickness were removed from the center of each tooth and fluorescence images of the adhesive interface were generated using both a helium-neon gas laser and an argon-ion laser. The fluorescent light was conducted through a filter-based fluorescence detection system, discriminating it from reflected and scattered light to obtain images of the resin–dentin interface with a magnification of 100 times at 1024 × 1024 pixels and 2.0 μs/pixel speed.
Two resin-bonded dentin slabs from each group were further processed for transmission electron microscopy (TEM). The slabs were coated with nail varnish, leaving 1 mm around the bonding interface exposed. Specimens were rehydrated with distilled water for 20 min and then immersed in an aqueous solution of 50% ammoniacal silver nitrate dye (pH 9.5) for 24 h to trace voids and porosity within the hybrid layer. The slabs were then placed into a photo-developing solution for 8 h under a fluorescent light to permit the reduction of the diamine silver ions into metallic silver grains, followed by washing under running water for 1 min and removal of the nail polish. For fixation, the slabs were immersed in 2.5% formaldehyde/glutaraldehyde in 0.1 M sodium cacodylate buffer at pH 7.4 for 12 h at 4 °C, rinsed four times with 20 mL of 0.2 M sodium cacodylate buffer at pH 7.4 for a total of 1 h, followed by distilled water bath for 1 min. The slabs were dehydrated in ascending concentrations of ethanol, embedded in epoxy resin, and sectioned with an ultramicrotome to obtain mineralized sections of 90–100 nm of thickness. The characteristics of nanoleakage at the resin–dentin interfaces were examined using a transmission electron microscope (H-8100, Hitachi, Tokyo, Japan) at an accelerating voltage of 100 kV.
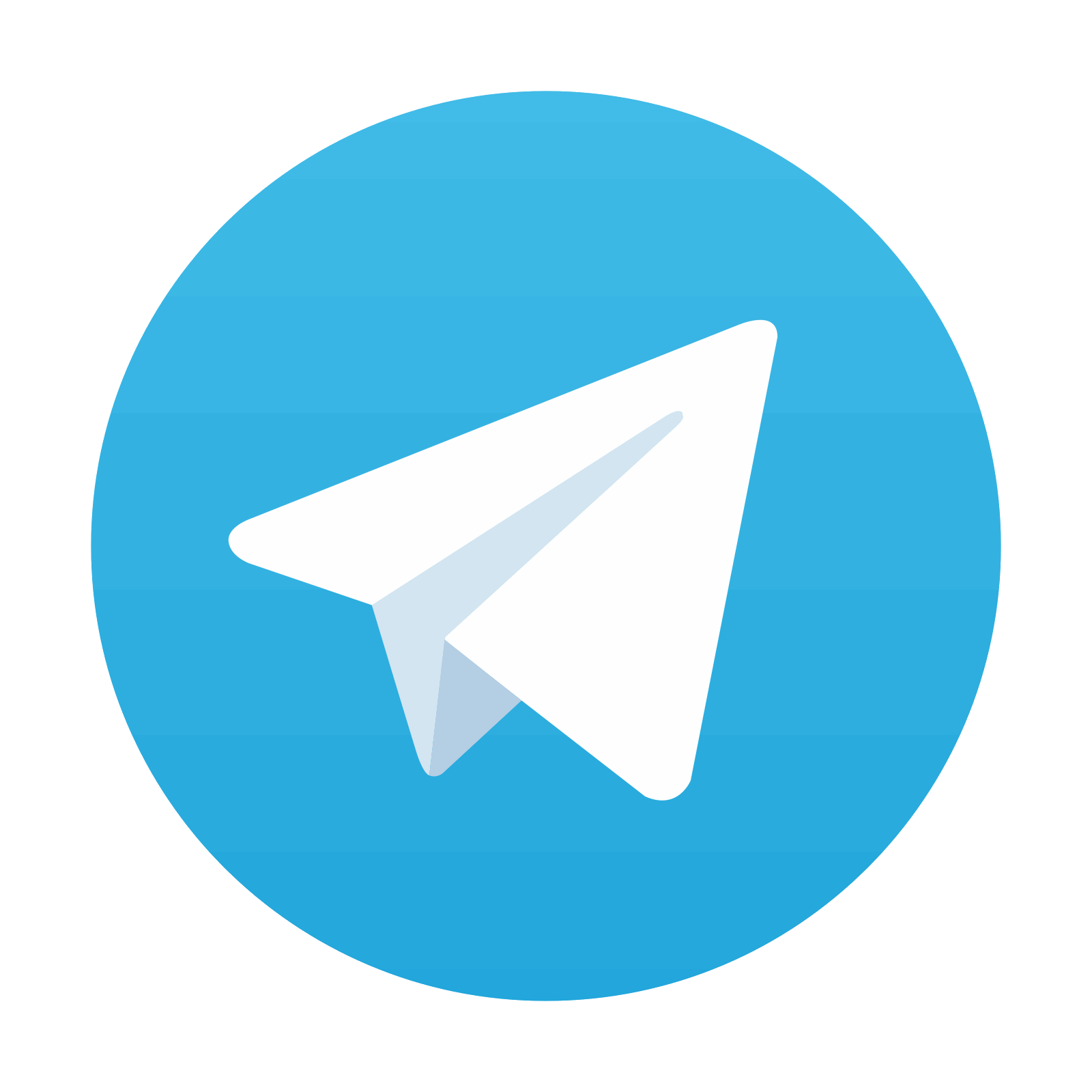
Stay updated, free dental videos. Join our Telegram channel

VIDEdental - Online dental courses
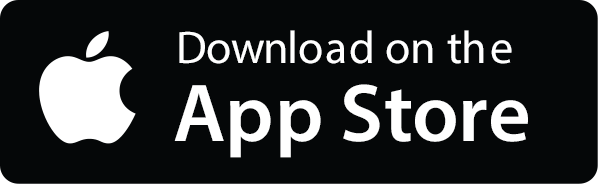
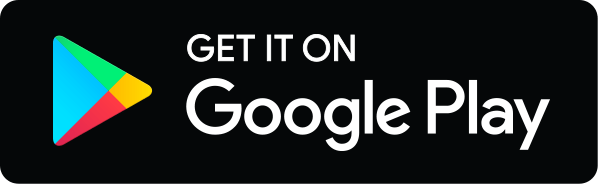