Graphical abstract

Abstract
Objectives
The combination of TiO 2 and H 2 O 2 under light activation constitutes a promising method for disinfection of dental prosthetics and implants, due to production of reactive oxygen species (ROS). The aim of this work was to investigate the organic degradation ability of TiO 2 particles in combination with H 2 O 2 and under light activation utilizing the organic dye rhodamine B (RhB).
Methods
Five different types of TiO 2 particles, consisting of anatase, rutile, or a mixture of these crystalline phases, were combined with H 2 O 2 and RhB, and subsequently exposed to UV (365 nm) or visible (405 nm) light at an irradiance of 2.1 mW/cm 2 .
Results
It was found that rutile in combination with low concentrations of H 2 O 2 (1.0–3.5 mM) resulted in a degradation of RhB of 96% and 77% after 10 min exposure to 365 nm and 405 nm light, respectively, which was the highest degradation of all test groups. Control measurements performed without light irradiation or irradiation at 470 nm, or without TiO 2 particles resulted in little or no degradation of RhB.
Conclusions
Low H 2 O 2 concentrations (1.0 mM–3.5 mM) and visible light (405 nm) used in combination with rutile TiO 2 particles showed the highest RhB degradation capacity.
Clinical significance
A combination of TiO 2 particles and H 2 O 2 exposed to low energy UV or high energy visible light has an organic degradation capability that could be utilized in applications to kill or inactivate bacteria on medical devices such as dental implants for treatment against, e.g. , peri-implantitis.
1
Introduction
Bacterial infection associated with dental implants and prosthetics is a serious problem that is difficult to combat. Biofilms readily form on the artificial surface of implants and often result in a destructive inflammatory process around the implants known as peri-implantitis. In fact, a review of the literature indicates a peri-implantitis prevalence of approximately 10% of implants and 20% of patients 5–10 years after implantation . There are several methods for decontaminating the implant surface and surrounding tissue, such as mechanical debridement using a curette or brush, ultrasonic and laser treatments, use of antiseptics such as chlorhexidine, hydrogen peroxide (H 2 O 2 ) or citric acid, and use of antibiotics . Unfortunately, none of these techniques are entirely satisfactory and thus there is presently no consensus on the gold standard for the treatment of peri-implantitis .
Photocatalytic generation of reactive oxygen species (ROS), such as hydroxyl radicals ( OH) and superoxide (O 2 − ), can be used to eliminate a wide range of organic contaminants and pollutants and can also be used in dental applications for removing bacterial biofilms . The photocatalytic reaction with titanium dioxide (TiO 2 ), which in its crystalline form is a semiconductor, takes place upon irradiating the material with light having sufficient energy. This generates a reactive electron-hole pair, which in turn produces ROS by reacting with O 2 or H 2 O at the surface of the crystal. Although effective in its own, it has been shown that the TiO 2 photocatalytic reaction can be combined with H 2 O 2 to generate synergetic effects , improving the ROS production and subsequent oxidation of organic material. In H 2 O 2 alone, absorption of light can cause a photolytic dissociation, producing two OH radicals directly. Applied separately or combined, these photo-induced reactions have been shown to be able to successfully remedy wastewater from a variety of organic contaminants , as well as inactivate bacteria that are known to cause biomedical implant associated infections , including methicillin resistant strains . ROS have also been reported to degrade the polysaccharide layers of bacterial biofilms , which make them suitable as disinfectant of peri-implantitis affected implants. As such, the photocatalytic approach offers an attractive alternative or compliment to certain antibiotic treatments. In a clinical setting, however, where decontamination of an infected implant takes place in situ , such as in the treatment of peri-implantitis, care must be taken to not harm any neighboring, healthy tissue. This implies that limits in concentration of oxidants and exposure to UV-light should be considered, motivating the development of TiO 2 /H 2 O 2 /UV–vis systems that are optimized for biomedical use.
The yield of ROS in TiO 2 photocatalytic reactions is dependent on the incoming photon energy. For the anatase crystalline phase of TiO 2 , which has a band gap energy of 3.2 eV , UV-light with a wavelength of 387 nm or less is required to generate an electron-hole pair. On the other hand, the rutile crystalline phase has a band gap energy of 3.03 eV , which corresponds to the energy of light in the visible region at 409 nm. In aqueous solutions of H 2 O 2 , the absorption of light is negligible in the visible and low energy UV region but increases exponentially with increasing energy in the UV region. Nevertheless, studies have reported generation of OH from TiO 2 /H 2 O 2 combinations under visible light conditions , and even from TiO 2 /H 2 O 2 suspensions kept in darkness . To date, however, no studies on the direct and interactive effects between these parameters have been made in the design space relevant for biomedical applications such as for disinfection of dental prosthetics and implants. Hence, this study systematically explores the organic degradation potential in the TiO 2 /H 2 O 2 /UV–vis system by varying TiO 2 and H 2 O 2 concentrations and photoactivation energy within acceptable limits from a biological perspective, to detect governing factors and potential synergy effects.
2
Materials and methods
Two series of experiments were performed to determine the organic degradation ability of the TiO 2 /H 2 O 2 /UV–vis system. The organic dye rhodamine B (RhB; Sigma Aldrich, St. Louis, Missouri, United States) was used as an indicator to estimate the system’s capacity to degrade organic material, and indirectly its capacity to produce ROS.
In the first series of experiments, mixtures of nano-sized TiO 2 particles Aeroxide ® P25 (lot number 4166031598, Evonik Industries, Hanau Wolfgang, Germany) and H 2 O 2 (Sigma Aldrich PERDROGEN TM, Steinheim, Germany) under different illumination conditions were investigated for their ability to degrade RhB. The TiO 2 particles consist of 80% anatase and 20% rutile, and are denoted as Anatase/Rutile in this study. When included in a sample, Anatase/Rutile was mixed to a concentration of 0.5 g/l and sonicated for 5 min to reduce particle aggregation. The concentration of H 2 O 2 was 1.9 M when included in a sample and the concentration of RhB for all test samples was 15 μM. Components were mixed with deionized water to the appropriate concentrations immediately prior to illumination of the entire sample area with 365 nm, 405 nm or 470 nm light using an LED at an exposure distance of 5 cm and irradiance of 2.1 mW/cm 2 . Irradiance was measured using an Ocean Optics HR 4000 spectrometer with an integrating sphere. A fourth illumination condition in which the samples were kept in darkness for 10 min was used as a control. Each sample had a volume of 4 ml and magnetic stirring was used for homogenization and to minimize settling of the TiO 2 particles. After irradiation the samples were introduced into a centrifuge tube and centrifuged for 10 min at 1500 g . A 1 ml aliquot of the supernatant was then placed in a 1.5 ml cuvette and the absorbance of the solution at 554 nm was measured in a UV–vis spectrophotometer (UV-1800, Shimadzu, Kyoto, Japan) for determination of RhB concentration. Each sample condition was tested in triplicate.
In the second series of experiments, the organic degradation potential of four TiO 2 powders were compared against each other at varying concentrations of H 2 O 2 and using one of two wavelengths of irradiation, namely 365 nm or 405 nm. In addition to Anatase/Rutile, the three other TiO 2 powders evaluated were Sachtleben Titandioxid Hombitan Anatase FF-Pharma, lot number 1170261004 (Sachtleben Chemie, Duisburg, Germany), titanium (IV) oxide rutile, lot number 03008JS (Sigma Aldrich, St. Louis, Missouri, United States), Kronos 1171 Titandioxid E 171, lot number 6073 (Kronos International, Leverkusen, Germany) and Aeroxide ® P25, lot number 4122298 (Evonik Industries, Hanau Wolfgang, Germany), and are denoted Anatase S, Rutile and Anatase K, respectively, in this study. All TiO 2 powders were mixed to a concentration 0.5 g/l and sonicated for 5 min to reduce particle aggregation. The concentrations of H 2 O 2 used were 0 M, 1.0 mM, 2.0 mM, 3.5 mM, 19.6 mM, 0.9 M or 1.9 M and the concentration of RhB in all test samples was 15 μM. Components were mixed with deionized water to the appropriate concentrations immediately prior to testing and thereafter 200 μl of the mixture was placed in a well of a black 96 well plate and exposed to 365 or 405 nm light at 2.1 mW/cm 2 for 10 min. During illumination, the well plate was placed on an orbital shaker (Talboys) to ensure sample homogenization and minimize settling of the TiO 2 particles. After illumination, the samples were removed from the well plate, centrifuged at 900 g to remove TiO 2 particles, then returned to a new 96 well plate for determination of RhB concentration using fluorescence measurements in a plate reader (Tecan Infinite M200; excitation wavelength 540 nm, emission wavelength 625 nm). Each sample condition was tested in triplicate.
The specific surface area (SSA) of the powders was determined from nitrogen gas sorption isotherms recorded at −196 °C in an ASAP 2020 instrument from Micromeritics (Norcross, GA, USA). Prior to analysis, all samples were degassed for 12 h at 90 °C. The SSA was calculated using the multipoint BrunauerEmmettTeller (BET) method for adsorption values in the relative pressure range between 0.05–0.40.
The TiO 2 particles were imaged using the in-lens detector in a LEO 1530 scanning electron microscope (SEM; Zeiss, Oberkochen, Germany), operated at 5.0 kV. The size of the particles, presented in Table 1 , was determined from SEM images as the average diameter of ten particles.
Name | % anatase | % rutile | Manufacturer | SSA (m 2 /g) | Particle size (nm) |
---|---|---|---|---|---|
Anatase S | 100 | 0 | Sachtleben | 11.2 ± 0.18 | 200 ± 60 a |
Rutile | 0 | 100 | Sigma Aldrich | 4.2 ± 0.15 | 900 ± 600 b |
Anatase K | 100 | 0 | Kronos | 6.1 ± 0.11 | 190 ± 60 a |
Anatase/Rutile | 80 | 20 | Evonik | 53.6 ± 0.6 | 30 ± 7 c |
Statistical analysis was performed using a one way analysis of variance (ANOVA) followed by a Tukey post hoc test, or a Dunnett T3 post hoc test in the case of unequal variances. The Anderson-Darling test was used to verify if the data sets were normally distributed while Levene’s test was used to assess the equality of variances.
2
Materials and methods
Two series of experiments were performed to determine the organic degradation ability of the TiO 2 /H 2 O 2 /UV–vis system. The organic dye rhodamine B (RhB; Sigma Aldrich, St. Louis, Missouri, United States) was used as an indicator to estimate the system’s capacity to degrade organic material, and indirectly its capacity to produce ROS.
In the first series of experiments, mixtures of nano-sized TiO 2 particles Aeroxide ® P25 (lot number 4166031598, Evonik Industries, Hanau Wolfgang, Germany) and H 2 O 2 (Sigma Aldrich PERDROGEN TM, Steinheim, Germany) under different illumination conditions were investigated for their ability to degrade RhB. The TiO 2 particles consist of 80% anatase and 20% rutile, and are denoted as Anatase/Rutile in this study. When included in a sample, Anatase/Rutile was mixed to a concentration of 0.5 g/l and sonicated for 5 min to reduce particle aggregation. The concentration of H 2 O 2 was 1.9 M when included in a sample and the concentration of RhB for all test samples was 15 μM. Components were mixed with deionized water to the appropriate concentrations immediately prior to illumination of the entire sample area with 365 nm, 405 nm or 470 nm light using an LED at an exposure distance of 5 cm and irradiance of 2.1 mW/cm 2 . Irradiance was measured using an Ocean Optics HR 4000 spectrometer with an integrating sphere. A fourth illumination condition in which the samples were kept in darkness for 10 min was used as a control. Each sample had a volume of 4 ml and magnetic stirring was used for homogenization and to minimize settling of the TiO 2 particles. After irradiation the samples were introduced into a centrifuge tube and centrifuged for 10 min at 1500 g . A 1 ml aliquot of the supernatant was then placed in a 1.5 ml cuvette and the absorbance of the solution at 554 nm was measured in a UV–vis spectrophotometer (UV-1800, Shimadzu, Kyoto, Japan) for determination of RhB concentration. Each sample condition was tested in triplicate.
In the second series of experiments, the organic degradation potential of four TiO 2 powders were compared against each other at varying concentrations of H 2 O 2 and using one of two wavelengths of irradiation, namely 365 nm or 405 nm. In addition to Anatase/Rutile, the three other TiO 2 powders evaluated were Sachtleben Titandioxid Hombitan Anatase FF-Pharma, lot number 1170261004 (Sachtleben Chemie, Duisburg, Germany), titanium (IV) oxide rutile, lot number 03008JS (Sigma Aldrich, St. Louis, Missouri, United States), Kronos 1171 Titandioxid E 171, lot number 6073 (Kronos International, Leverkusen, Germany) and Aeroxide ® P25, lot number 4122298 (Evonik Industries, Hanau Wolfgang, Germany), and are denoted Anatase S, Rutile and Anatase K, respectively, in this study. All TiO 2 powders were mixed to a concentration 0.5 g/l and sonicated for 5 min to reduce particle aggregation. The concentrations of H 2 O 2 used were 0 M, 1.0 mM, 2.0 mM, 3.5 mM, 19.6 mM, 0.9 M or 1.9 M and the concentration of RhB in all test samples was 15 μM. Components were mixed with deionized water to the appropriate concentrations immediately prior to testing and thereafter 200 μl of the mixture was placed in a well of a black 96 well plate and exposed to 365 or 405 nm light at 2.1 mW/cm 2 for 10 min. During illumination, the well plate was placed on an orbital shaker (Talboys) to ensure sample homogenization and minimize settling of the TiO 2 particles. After illumination, the samples were removed from the well plate, centrifuged at 900 g to remove TiO 2 particles, then returned to a new 96 well plate for determination of RhB concentration using fluorescence measurements in a plate reader (Tecan Infinite M200; excitation wavelength 540 nm, emission wavelength 625 nm). Each sample condition was tested in triplicate.
The specific surface area (SSA) of the powders was determined from nitrogen gas sorption isotherms recorded at −196 °C in an ASAP 2020 instrument from Micromeritics (Norcross, GA, USA). Prior to analysis, all samples were degassed for 12 h at 90 °C. The SSA was calculated using the multipoint BrunauerEmmettTeller (BET) method for adsorption values in the relative pressure range between 0.05–0.40.
The TiO 2 particles were imaged using the in-lens detector in a LEO 1530 scanning electron microscope (SEM; Zeiss, Oberkochen, Germany), operated at 5.0 kV. The size of the particles, presented in Table 1 , was determined from SEM images as the average diameter of ten particles.
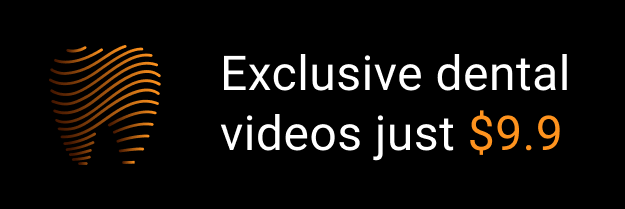