div epub:type=”chapter” role=”doc-chapter”>
P. Jain, M. Gupta (eds.)Digitization in Dentistryhttps://doi.org/10.1007/978-3-030-65169-5_3
3. Digital Oral Radiography
Digital oral radiographyCone beam computed tomographyDental implantEndodonticsMaxillofacial surgery
3.1 Introduction
Since the discovery of X-rays and the first dental radiography, radiographic examination is essential for diagnosis. Until the mid-1980s, analog radiographic films were the only options for image receivers and played an important role in health services, clinics, and in teaching at dental schools. Besides the high radiation dose, there was a need for chemical processing to visualize the image, which is harmful to the environment.
Digital radiology was a revolution to dentistry and medicine. It was in 1987, in Geneva, that Francis Moyen demonstrated the first intraoral digital radiography system for dentistry. It was called radiovisiography. Since then, there were many technological advancements, which made it possible, that, nowadays, we have the best equipment available in the market [1]. The radiographic process waived the use of chemicals and started being digital, besides the advancement of the image manipulation resources, which greatly contributes to the diagnoses [1, 2].
The conventional radiographic examinations presented as two-dimensional images are widely used in dentistry, especially panoramic and intraoral radiographies (periapical and interproximal). Besides the innumerous advantages, such as high spatial resolution for the intraoral images and low radiation dose for both, some limitations are evident, such as the compression of three-dimensional anatomy, geometry distortion, and anatomical noise, which may impair the diagnosis. In cases where tridimensional assessment is required, patients will be referred for a computed tomography scan, CBCT being the most requested for dental and maxillofacial purposes.
This chapter will cover digital images features and acquisition, digital receivers, the pre- and post-processing phases of digital images, and the image manipulation tools. Additionally, many aspects of the CBCT will be described, emphasizing on the main indications of this examination and how to improve image quality for diagnosis.
3.2 Digital Radiograph
Intraoral radiographs are still considered the first-choice method for diagnosis, treatment, and patient follow-up, since they are efficient, low dose, and low cost. Digital radiographs have several advantages, such as image quality enhancements, nearly instantaneous availability of images, reduction of working time, the elimination of chemical processing, and easier image storage and communication [1, 2].
After exposure, the X-ray beam is attenuated by the tissues and reaches the image receiver, which receives analog information. This information is subsequently converted into numbers by a computerized process called analog to digital conversion [2]. This process takes place in two steps: sampling (a process that groups one voltage range) and quantification (a process that attributes a number to each range). Finally, the digital image is presented and stored in a computer.
Digital image is formed by a matrix that corresponds to a group of lines and columns of pixels (the smallest units of a digital image). Digital images are fundamentally composed of numbers that determine pixels’ spatial distribution and the gray values attributed to them. The gray values vary according to the intensity of radiation absorbed during the digital receiver’s exposure to the X-rays. Thereby, it is ensured that digital images are numerical and distinct [3].
Pixel matrix, pixel size, and bit depth determine important features of the digital image. Currently, the pixel size of radiographic images acquired through intraoral digital receptors is about 19–60 μm, thus providing a maximum spatial resolution that is no less than the usual radiographic film. Digital image acquisition software offers various bit depths, allowing image acquisition with 8, 10, 12, 14, or 16 bits generating images with respectively 256, 1024, 4096, 16,384, and 65,536 levels of gray. It is worth pointing out that the deeper the bit and the smaller the pixel, the bigger the contrast resolution and the spatial resolution, respectively. In theory, this allows better representation capacity of the different degrees of tissue attenuation, as well as the distinction of contiguous points in the image, resulting in better visualization of subtle differences [4]. Nevertheless, it is known that the human eye is only able to distinguish a limited amount of shades of gray.
3.3 Digital Image Acquisition
Digital image acquisition can be performed in three distinct ways: indirect acquisition by means of digitizing films, direct acquisition, using solid-state digital receivers and semi-direct acquisition using a phosphor plate system [1].
3.3.1 Indirect Digital Acquisition
On the indirect acquisition, the image is acquired through the usual method, using a conventional radiographic film and, after that, the information is captured and digitalized. The film’s image capture and digitalization can be accomplished through a flatbed scanner with transparency adapted or digital cameras [1].This digital radiographic image acquisition method enables the use of the available digital tools to enhance the digital image in various software. However, a larger amount of working time is required for the digital image acquisition, thus adding one more step to the image acquisition.
3.3.2 Direct Digital Acquisition
In the direct digital radiographic acquisition, the radiographic film is replaced by a solid-state receiver, which can be charged coupled devices or complementary metal oxide semiconductors.
On the intraoral solid-state receivers, a thick, rectangular-shaped, rigid plastic casing protects a silicon chip and other components against deterioration and contamination. Most of these intraoral receivers are connected to the computer by a cable. The CCD receiver has a silicon matrix organized in the same way as the pixels. Above this matrix, there is a layer of scintillator material. Some manufacturers add an optic-fiber layer between the scintillator layer and the silicon chip to protect the matrix (pixels) from direct X-ray penetration and thus prolong the sensor life span. The CMOS-type sensors have a similar structure to the CCD type, consisting of a pixel array but differing from the aforementioned on the way charges are transferred and read [5].
There are intraoral direct digital systems that use wireless technology to transmit digital image information [1, 6]. These systems can use common radio-frequency waves [6] or bluetooth waves. On the systems that use bluetooth waves, the sensor is connected to a base station through a cable, and the data transmission from this station to the computer happens as waves. The base is magnetized, or the systems provide a holder to stow and fixate the equipment on any surface during image acquisition. The systems that use this technology usually have a receiver antenna in the form of a USB flash drive that must be inserted into the computer’s USB port.
One of the systems that use bluetooth waves on data transmission (X-POD, MyRay, Imola, Italy) also allows image acquisition without a computer, since it has a base unit with a touch screen to control image acquisition, visualization, and managing. The images are saved and organized in folders for each patient, stored on the removable secure digital memory card. In this case, the images are stored in a memory card, and afterward synchronize the equipment with the computer software and use bluetooth waves or connect the base unit to the computer through a USB cable to transmit the radiographic images.
The CDR wireless system (Schick Technologies, Inc., Long Island City, NY, USA) was the first wireless solid-state digital system, and it uses a battery on the sensor’s posterior surface (non-active side) and emits radio-frequency waves to a base station. This station captures the waves through a receiver antenna and transmits the information to a computer via an optic-fiber cable and a USB interface. The disadvantage of this system is the increased thickness of the sensor compared to other receivers [1, 6].
Extraoral direct digital sensor presents pixels on long, linear, and thin matrix. This matrix has few pixels widthwise and many pixels lengthwise. The long and narrow pixel matrix is aligned with the X-ray beam and, for the image acquisition, there is a scanning movement of the area to be exposed. When solid-state receivers are used, the extraoral radiography acquisition process takes a few seconds from the exposure to X-rays until the appearance of the image on the computer monitor [1].
The main advantage of solid-state digital systems is that the image appears almost instantly after the exposure of the receiver to X-rays. The smaller active area of image receivers and the reduced dynamic scale when compared to films and PSP plates are disadvantages of the intraoral solid-state receivers. Besides, the rigid and thicker plastic pack and the cable may bring discomfort to the patient and complicate the placement of the receiver.
3.3.3 Semi-Direct Digital Acquisition
In the semi-direct digital acquisition, the conventional radiographic film is replaced by the PSP plate. When exposed to X-rays, these plates absorb and store energy, forming a latent image. To visualize the image, the PSP plate must be processed after exposure by scanning the plate through each system’s scanner (data reading) [3].
PSP plates are comprised of a polyester base with one of its surfaces covered by a europium-doped barium fluoride halide (active side). The combination of barium with chloride, iodine, or boron forms a crystalline gelatin. When those plates are exposed to X-rays, europium’s valence electrons absorb energy and may jump to the conduction bands. These electrons might migrate to halide vacancies (F centers) and be captured in a latent state. While remaining in this state, the number of captured electrons is proportional to the exposure to X-rays, and it represents a latent image (invisible and nonpermanent).
During the scanning step, after adapting the plate on the system scanner, the scanner will perform the sweeping of the plate through a thin collimated laser beam that will stimulate the release the energy stored. The information is converted to electrical signals, subsequently converted into binary digits through an analog to digital converter, and the image is visualized. The time required for the plate reading step varies from some seconds to a few minutes depending on the digital system scan resolution, brand, and manufacturer. Usually, intraoral PSP scanners process and display images in a matter of seconds.
The spatial resolution of images acquired by PSP plates systems is determined by some factors, such as phosphor layer thickness and laser diameter. The thicker the plate and the bigger the laser diameter, the smaller the image resolution. Another important issue is the interval between exposure and scanning of the PSP plate. After exposure to X-rays, the plates must be scanned as swiftly as possible, since some studies have shown changes in pixel values and these changes may cause loss of quality and affect clinical image interpretation when too much time elapses between exposure and the scanning [7]. Furthermore, during plate manipulation for scanning, one must follow the manufacturer’s recommendations regarding the ambient light since it can potentially alter image quality.
After the plate scanning process, some energy is still left on the plate, and thus some electrons remain as “energy traps” (residual image). To use the PSP plate for another radiographic acquisition, the residual image sign must be eliminated by the active side of the plate exposure to a bright light source [1]. This process is done within the scanner itself right after the scanning. Subsequently, the phosphor plates must be stored on a lightproof recipient. It is recommended that the intraoral plates be stored on polyvinyl envelopes to protect them from oral fluids. Extraoral phosphor plates are positioned in chassis without image intensifiers.
PSP plates are available in various sizes which are compatible with the many sizes of conventional radiographic films. There are PSP digital systems that have phosphor plates with sizes compatible with intraoral radiographic films (sizes 0, 1, 2, 3, and 4) and others that, in addition to these plates sizes, have plates with similar sizes to the extraoral radiographic films.
PSP digital system has a wide dynamic range that avoids retakes by under-exposed and over-exposed images [7]. Besides, the PSP plate is more acceptable to patients because it is a wireless receiver, has comparable flexibility to conventional films, and is thinner than a solid-state receiver [7]. On the other hand, image acquisition and visualization happens in a few seconds since more steps are needed to visualize the image. Besides, the PSP plates are easily damaged during handling in dental practice, which deteriorates image quality by the presence of image artifacts as scratches and stains and are sensitive to rubbing with some disinfectants [7]. It is a disadvantage as it represents the need for phosphor plate continual reposition. To reduce damage risk during handling, some intraoral systems have protective covers.
The intraoral digital receivers and the surfaces of head stabilizing and positioning apparatus of extraoral direct digital Xray machines must be protected by disposable surface barriers against cross-contamination. A two-layer barrier may be used to prevent contamination of the intraoral receivers [7]. Some manufacturers introduced an automated internal ultraviolet (UV) disinfection feature that according to them inactivates viruses and bacteria on the plate transport mechanism of intraoral PSP digital systems.
3.4 Digital Radiographic Processing
Digital radiographic systems have several tools that allow imaging adjustment, which generally aim to improve its quality and/or ease its assessment. These adjustments are called radiographic processing and can occur at two different moments: first at the digital reading and conversion of data by the computer immediately after the radiographic acquisition, but before the image display on the monitor, named pre-processing; and after the appearance of the image on the computer screen, also known as post-processing.
3.4.1 Pre-Processing of Digital Images
All the adjustments automatically made by the software owner of the digital systems before the image appears on the computer screen are classified as pre-processing. The occurrence and degree of adjustments to the images happen regardless of the professional’s will and, in most systems, these adjustments cannot be disabled.
Among the main pre-processing adjustments, the replacement of defective pixel values in CCD systems and the automatic exposure compensation (AEC), present in most direct digital systems, can be mentioned. In the first case, the replacement of defective pixel values of CCD systems by the weighted average of the values of neighboring pixels is performed in order to homogenize the images and reduce noise. The AEC is a nonlinear enhancement of the image that identifies the lowest and highest pixel values and changes the grayscale to increase radiographic contrast. Thus, the AEC favors the production of images of high contrast even when there are variations in the exposure of the image receiver to the X-rays, possibly increasing the dynamic range of digital radiography (exposure range in which it is possible to obtain images clinically suitable) [8]. Furthermore, it was recently demonstrated that the presence of high-density materials in the imaged region, such as metallic restorations, endodontic materials, and dental implants, is able to alter the gray values of the whole image because of the presence of AEC, further increasing the contrast of the images [9]. However, little is known about the possible influence of this automatic correction on the diagnosis of different dental conditions.
One of the advantages of pre-processing integrated with digital systems is the improvement of image quality, often automatically correcting under- or overexposed images. On the other hand, the fact that this processing is hidden from the user often prevents such conditions to be perceived by the professional and corrected clinically.
3.4.2 Post-Processing of Digital Images
The post-processing of the images comprises the adjustments made manually and subjectively by the operator, after viewing the image on the computer monitor. Any operation that the professional performs to improve, restore, modify, or analyze the image is considered post-processing. Generally, such modifications can be undone in the software used during post-processing.
The main adjustments that can be made in digital radiography are related to the modification of the size of the pixels and changes in the histogram (distribution of gray values) of the image. Digital systems can have several image manipulations tools, and these can be accessed in the radiographic acquisition system software or in specific software for this purpose. Among the most commonly used tools, that we can mention are manipulation of brightness and contrast, zoom, linear and angular measurements. In addition, the inverted radiography and pseudocolor tools and the filters of relief, sharpness, and smoothing are also generally available in the post-processing software. The main modifications made to the image by each tool are described as follows.

Examples of post-processing of a digital image: original image (a) and enhanced images with brightness (b, c) and contrast (d, e) adjustments, linear measurements (f) and, high relief (g), pseudocolor (h), and radiographic inverted view (i)
Zoom: Zoom refers to the magnification of the image on the monitor and, together with the brightness and contrast, is one of the tools most used in the assessment of digital radiographs. The zoom facilitates the visualization of details in the image, but it must be used sparingly, since when enlarging the digital image, its pixels may become evident. The higher the spatial resolution of the image, the greater its power of magnification without loss of image quality.
Measurements: Linear and angular measurements are widely used in several specialties in dentistry, and their precision has been proven by various studies. Examples of its applications involve the determination of the measurements of root canal systems in endodontics, cephalometric measurements in orthodontics, and determining the height of the alveolar ridge in edentulous regions in implantology (Fig. 3.1f).
Sharpness and smoothing: These filters are intended, respectively, to enhance and soften the boundary between the structures. Both filters represent mathematical algorithms that reduce the blur or noise image, improving its quality. In this way, the radiograph becomes more attractive. However, the improvement in the diagnostic performance of images with filters is still controversial in the literature.
High relief: The high relief, relief, or three-dimensional tool gives the appearance of relief to structures of greater density, highlighting the limits of structures of different degrees of attenuation to X-rays, such as enamel and dentin (Fig. 3.1g).
Pseudocolor: Consists in transforming gray tones to image color. This tool does not have clinical applicability, considering that it does not add any real factor that can improve the diagnosis. On the contrary, pseudocolor can modify the limits and the appearance of the real object (Fig. 3.1h).
Inverted radiography: The radiographic inverted view is based on the inversion of the gray values of the image pixels. With the use of this tool, radiolucent structures become radiopaque and vice versa on radiographs. However, this tool does not seem to bring benefits to the diagnosis in dentistry (Fig. 3.1i).
3.5 Digital Subtraction Radiography
In addition to these post-processing tools, in some software it is possible to perform a technique called digital subtraction radiography. It aims to compare two standardized radiographs from the same region, taken at different times. To do this, the software subtracts similar structures in the two images, that is, those that have not changed during the time interval, presenting them in an intermediate gray shade. In the areas of tissue loss and gain, respectively, a darker shade of gray and a lighter shade of gray are added to the image. Subtraction is indicated for the evaluation of tissue repair or healing, monitoring of patients with periodontal disease, among other indications. The main challenge for performing this technique, however, is the accurate standardization of the radiograph, since small changes in the image acquisition geometry can substantially interfere with the result of radiographic subtraction.
3.6 Cone Beam Computed Tomography (CBCT)
Cone beam CT (CBCT) is a three-dimensional imaging technique commonly used in dentistry. Initially, it was developed to use in angiography and, after that, based on the studies by Mozzo et al. [10] in 1998, a machine specifically designed for dental and maxillofacial evaluation was created. However, it was only in the mid-2000 that its use became more widespread. Since then, dental practitioners have been requesting CBCT for their patients. The criteria for referring patients to CBCT should be carefully selected because of its higher radiation dose compared to conventional radiographs, especially in cases of younger patients.
In all dental specialties, CBCT should be indicated only when it is believed that this examination will provide additional information that may alter the patient’s diagnosis, treatment plan, or prognosis.
3.7 Clinical Applications of CBCT
3.7.1 Dental Implant Treatment Planning

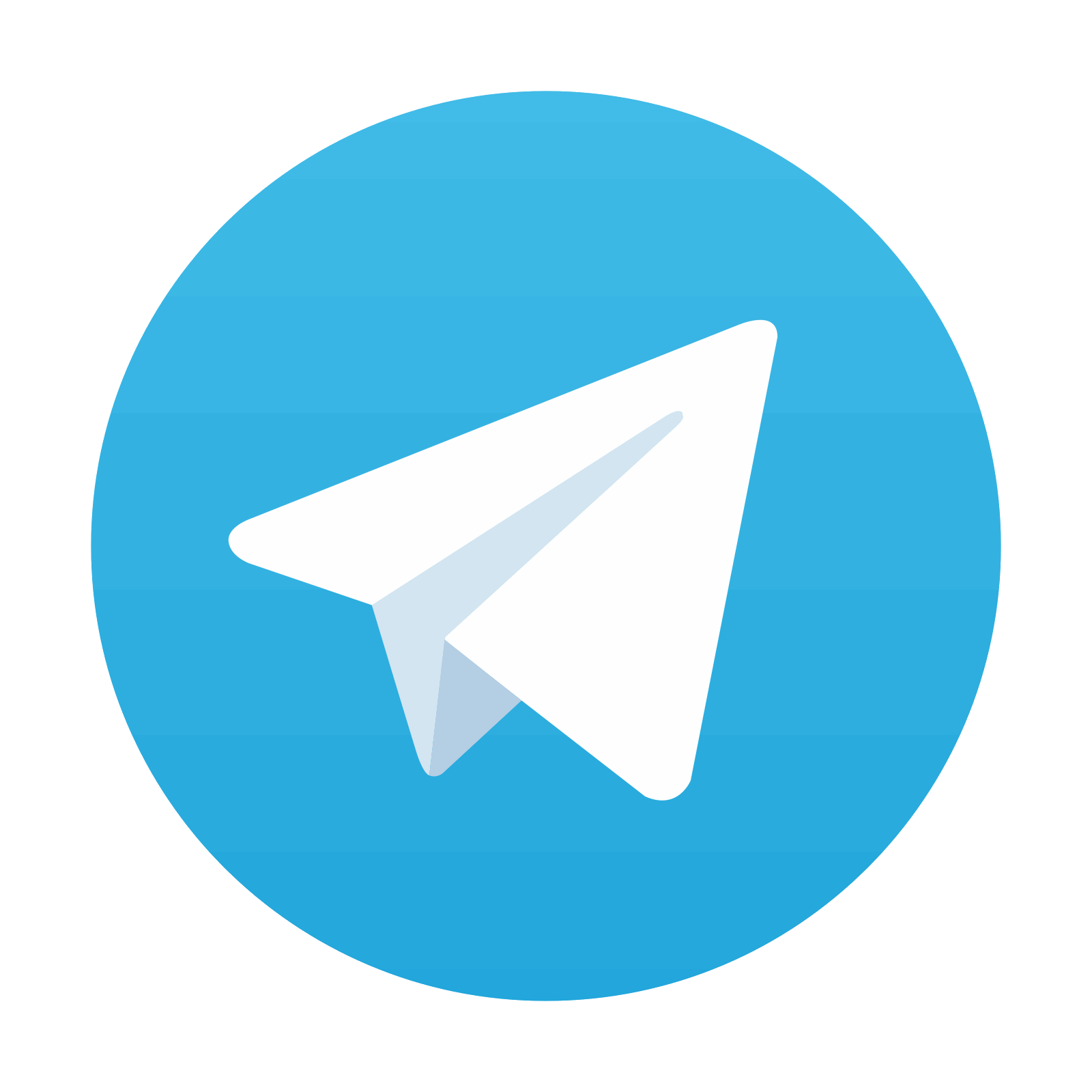
Stay updated, free dental videos. Join our Telegram channel

VIDEdental - Online dental courses
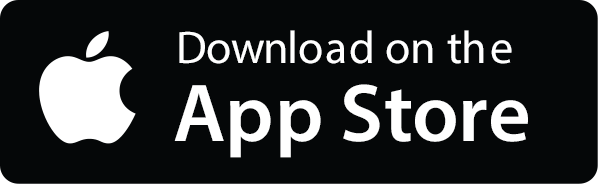
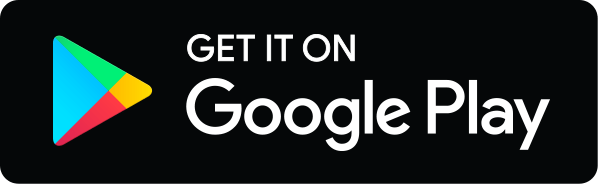