Immediate implant placement protocol which includes (a) and (b) careful and atraumatic tooth extraction; (c) flapless implant placement in the ideal prosthetically driven position; (d) placement of a slow resorbing bone replacement graft in the buccal gap; (e) placement of a collagen-based soft tissue substitute to enhance the buccal soft tissue volume; (f) placement of an immediate provisional restoration; (g–i) clinical, radiographical and aesthetic outcome
From a surgical point of view, the attainment of a correct 3D implant positioning with good primary stability in sufficient apical bone availability is an essential factor for achieving successful outcomes with immediate implants. Additional stability may be obtained by anchoring the implant in the bony structures of the alveolar walls or inter-radicular septa, although an excessive buccal positioning of the implant must always be avoided as well as the choice of implants that are too congruent with the socket anatomy. An adequate gap dimension (>2 mm) between the implant and the inner surface of the buccal bone plate crestally is fundamental to allow for satisfactory bone healing [23]. In an apico-coronal dimension, it is important that the final position of the implant shoulder is placed at least 1 mm apical to the buccal ridge, in order to compensate for the expected vertical resorption [24].
6.4 Early Implants
It is rather frequent that the integrity of the socket bone walls is affected by the underlying pathology that justified the tooth extraction or by the possible trauma to the bone during the extraction procedure. In these situations, where mainly the buccal bone wall is totally or partially absent, the immediate implant protocol should not be indicated, and the early implant placement protocol (type 2) has been proposed. This treatment modality reduces the risk for postsurgical complications since it allows for primary healing after tooth extraction. This proposed healing time (4–6 weeks) usually allows for soft tissue healing but at the same time permits the anchorage of the implant in the socket walls before they become fully resorbed. Since the bucal bone wall is either partially or totally absent, there is a need for regenerative interventions in conjunction with implant placement. The presence of adequate soft tissue usually allows for primary closure, thus reducing the risks of infeccion and soft tissue dehiscence. This is especially relevant in the presence of thin biotypes, where significant hard and soft tissue resorptive changes usually occur [25].

Early implant placement protocol which includes (a) soft tissue healing 4 weeks after tooth extraction; (b) alveolar bone healing 4 weeks after tooth extraction; (c) implant placement with resulting dehiscence defect; (d) placement of an autogenous bone graft over the exposed implant threads; (e) over-contouring the buccal bone crest with a slow resorbing bone replacement graft; (f) placement of a bioabsorbable collagen membrane fixed with titanium tags; (g) bone regenerative outcome 4 months after; (h) placement of a provisional restoration; (i, j) radiographical and aesthetic outcome
6.5 Ridge Preservation

Socket preservation protocol which includes (a, b) careful and atraumatic tooth extraction; (c) placement of a slow resorbing bone replacement graft inside the socket; (d) placement of a collagen-based soft tissue substitute to seal the socket opening; (e) healing at 2 weeks; (f) healing at 4 months; (g, h) placement of a dental implant in the ideal prosthetically driven position; (i) placement of a provisional restoration; (j) placement of the final restoration
In humans, the application of regenerative biomaterials, such as bone autografts, allografts, guided tissue regeneration procedures, xenografts and, most recently, growth factors, has also been evaluated with varying degrees of success to maintain the anatomical dimensions of the alveolar ridge after tooth extraction. Systematic reviews have evaluated the efficacy of these interventions reporting that socket preservation techniques do not prevent the physiological resorptive bone processes after tooth extraction, although they may significantly reduce the resulting bone dimensional changes [29]. The results from the meta-analysis demonstrated statistically significant higher alveolar bone crest preservation in both height and width in the interventions for ridge preservation when compared with the healing of the untreated control socket. The results of the meta-regression analysis showed that the surgical procedures attaining primary closure of the socket opening (by advancing flaps of using barrier membranes or soft tissue autografts) demonstrated a significantly lesser horizontal and vertical resorption of the socket [30]. These results suggest the importance of achieving full closure and first intention healing, thus avoiding the contamination and disturbance of the healing of the biomaterial or regenerative technology.
6.6 Bone Regeneration of Residual Alveolar Ridges
Alteration of the anatomy of the residual alveolar ridge may be the consequence of the underlying pathology that caused tooth loss (trauma, chronic/acute infections or severe periodontal disease). Even in the absence of overt pathological conditions, the loss of mechanical function following tooth extraction or tooth loss will cause severe atrophic changes in the residual alveolar ridge, which may limit the availability for adequate implant placement. In fact, bone availability is the main prerequisite for safe and predictable implant placement, since an adequate amount of bone is required for attaining the functional stability of the implant needed to achieve osseointegration. Moreover, the goal of achieving adequate aesthetic outcomes requires the optimal three-dimensional position of the implant following a planned prosthetic reconstruction. The frequent presence of residual ridge deficiencies, both in horizontal and vertical dimensions, demands the indication of bone augmentation procedures, either concomitant with implant placement or as a staged intervention.
These crestal changes may be different depending on the region of the affected jaw and, similarly, the functional and aesthetic demands of the patient may vary, what advocates for an individual assessment of the possible need of bone augmentation procedures. The available bone crest, therefore, must be carefully evaluated clinically and mainly by means of a three-dimensional radiographic evaluation with the use of modern CBCT (cone beam computed tomography) technologies.
-
Class 1 defect, when the bone deficiency affected predominantly the horizontal dimension
-
Class 2 defect, when the bone deficiency affected predominantly the vertical dimension
-
Class 3 defect, when the bone deficiency affected both the vertical and horizontal dimensions
A careful diagnosis of the residual alveolar ridge is also fundamental for the selection of the appropriate regenerative strategy and technology, although any bone augmentation therapy must be based in a set of fundamental biological principles of wound healing, including the promotion of primary wound closure, the enhancing of cell delivery and neo-angiogenesis by attaining initial wound stability and avoiding contamination of the regenerative process.
Primary closure is, therefore, primordial to assure an undisturbed environment for healing and must be assured by appropriate flap management and suturing. This requires an adequate amount of soft tissue present before the regenerative surgery [32]. The resulting flap must cover the regenerated area, and, when sutured, it should be relatively passive and tension-free. Proper cell proliferation and differentiation are also fundamental in the wound healing process to provide the neo-angiogenesis and the differentiation of the osteogenic cells needed for regenerating the bone. The main sources of osteogenic cells include the periosteum and endosteum from the walls of the bone defects, as well as the bone marrow. These cells include osteoblasts and undifferentiated mesenchymal cells, which can be differentiated into osteoblasts in the presence of the adequate signalling molecules, nutrients and growth factors. This process requires adequate blood supply for providing not only oxygen and nutrients but also as a source of mesenchymal cells. To promote these early healing events, perforations of the cortical plate have been recommended to facilitate cell migration [33]. Another key factor that affects the wound healing is the stability of the blood clot. This is important since the clot promotes the formation of granulation tissue, which subsequently will develop into bone [34]. Moreover, the clot contains a myriad of cytokines (e.g. interleukin-1 (IL-1), IL-8, tumour necrosis factor), growth factors (e.g. platelet-derived growth factor (PDGF), insulin-like growth factor-1 (IGF-1), fibroblast growth factor-2 (FGF-2)) and signalling molecules that aid in recruiting cells to promote angiogenesis and bone regeneration. In most clinical situations, this clot stability can only be assured when physical space is provided by means of a scaffold and by exclusion of the soft tissue cells from the mucosal. This is usually assured by the placement of a barrier membrane between the bone replacement graft serving as scaffold and the inner surface of the flap.
The rationale behind any crestal bone augmentation procedure is not only to establish sufficient bone availability for the safe and predictable placement of a dental implant but also to provide adequate bone thickness around the installed implant. In fact, the influence of bone thickness around an implant has been evaluated during the second-stage surgery, demonstrating that thicknesses ≤1.5 were frequently associated with bone loss and dehiscence lesions (exposed implant surface), while as the bone thickness approached 1.8–2 mm, the occurrence of dehiscence decreased significantly [35]. Although the ‘adequate’ bone thickness may vary depending on the macroscopic and microscopic implant configurations, as well as in the clinical indication, it is generally agreed that at least 2 mm of the bone on the buccal side of the implant are needed to achieve long-term stability of peri-implant soft tissues and hence to attain adequate aesthetic outcomes [36].
In bone augmentation procedures of the residual alveolar crest, the treatment strategy may include the placement of an implant and the regeneration during the same surgical intervention (one-step procedure) or the delay of implant placement until enough bone volume has been augmented (staged procedure). The one-step procedure is indicated in class 1 defects, when there is enough vertical bone for placing an implant with appropriate primary stability and in the proper prosthetically driven position (lateral bone augmentation). In class 2 and 3 defects, depending on the amount of vertical augmentation needed, the staged procedure is usually indicated.
Different biomaterials have been tested as bone replacement grafts and barrier membranes in bone regenerative interventions in alveolar ridges.
As bone replacement grafts, natural and/or synthetic biomaterials have been developed, investigated and used in bone augmentation procedures. Autogenous bone grafts (autografts) have been historically the gold standard in alveolar bone regenerative therapies due to their well-documented osteoconductive, osteoinductive and osteogenic properties [37]. Their major limitation, however, is the morbidity and complications associated with their harvesting, which depends on the volume needed depending on the size of the defect. Furthermore, autografts suffer fast resorption, which requires early implant placement to provide functional loading to the regenerated bone, and even with early implant placement, significant resorption occurs. Particulate bone autografts are usually harvested from intra-oral sites and used in combination with barrier membranes following the principles of guided bone regeneration . Mono-cortical block autografts are indicated in large crestal defects, where there is a need for vertical bone augmentation, since their compressive properties allow for excellent space maintenance. These autogenous grafts may be harvested from intra- or extra-oral sites. Common intra-oral donor sites are the mandibular chin or the ascending ramus area, whereas common extra-oral donor sites are the iliac crest or the calotte. They may be used alone or in combination with barrier membranes, and they require fixation to the recipient crestal site with mini-screws to avoid micro-movements during healing. Their main disadvantage is the morbidity associated with their harvesting, and similar to particulate autografts, their resorption rate is high.
To overcome these shortcomings, natural or synthetic bone substitutes, either used alone or in combination with bone autografts, have become the standard biomaterials in bone augmentation procedures. These bone substitutes must fulfil the following requirements for their use in bone regeneration in periodontology and oral and maxillofacial surgery: biocompatibility, osteoconductivity, adequate mechanical support to provide the volume for the regenerated bone, biodegradability and being replaced by the patient’s own bone, although recent studies have suggested that a slow degradation or even non-resorption may be advantageous for the maintenance of the augmented volume.
Depending on their origin, bone substitutes have been classified in three groups: allografts, from human origin; xenografts, from another animal species, usually bovine or equine; and alloplasts, which are synthetically produced. Allografts are bone grafts harvested from cadaver donors and processed by freezing or demineralizing and then sterilized and supplied by specially licenced tissue banks as bone particles or blocks. Allografts include fresh-frozen bone (FFB), freeze-dried bone (FDB) and demineralized freeze-dried bone (DFDB). Their main limitation is the potential risk of cross-infection or immunologic reactions due to their protein content, what cannot exclude the possibility of disease transmission. Nevertheless, there are no reported cases from the use of demineralized freeze-dried bone allografts (DFDBA) for dental purposes in over one million cases over 25 years [37]. DFDBA have shown osteoconductive as well as osteoinductive properties, due to the release of bone morphogenetic proteins (BMPs) during the demineralization process. Clinically, these allografts are usually combined with barrier membranes following the principles of guided bone regeneration.
Xenografts are graft biomaterials of animal origin, mainly bovine and equine. These graft materials are usually de-proteinized by means of a chemical or low-heat process. This technique preserves the original bone architecture and the inorganic mineral bone composition but removes the organic component, thus preventing any possibility of immunogenic reactions. Anorganic bovine bone grafts (ABBG) and de-proteinized bovine bone mineral (DBBM) have shown good biocompatibility and osteoconductivity in preclinical studies when used following the principles of GBR, as porous particulate granules combined with a bioabsorbable collagen barrier membrane [38]. Moreover, these xenogeneic grafts have shown a very slow resorption rate what assures their long-term stability.
Alloplastic bone substitutes are synthetic grafts that include different combinations of calcium phosphates, bioactive glasses and polymers fabricated under different manufacturing and sintering conditions, hence resulting in different physical properties and resorption rates. Hydroxyapatite (HA) constitutes the main mineral component of the natural bone, and it is the least soluble of the naturally occurring calcium phosphate salts, what provides an osteoconductive scaffolding function, being highly resistant to physiologic resorption [39]. In contrast, tricalcium phosphate (TCP) is characterized by rapid resorption and replacement by host tissue during the early phases of healing.
Similar to bone replacement grafts, different types of biomaterials have been tested as barrier membranes for guided bone regeneration (GBR) . Their specific composition falls into two broad categories: non-absorbable and bioabsorbable. Non-absorbable e-PTFE membranes have been frequently used in bone regeneration clinical applications, mainly with reinforced titanium strips, thus providing space-making capacity for increased bone augmentation. However, the need of a second surgical intervention for their removal and the frequent occurrence of postoperative complications, mainly early membrane exposure, have limited their clinical use, resulting in the much broader use of biodegradable membranes. These bioabsorbable membranes must, however, ensure that the process of membrane resorption or biodegradation does not lead to tissue reactions that may affect the outcome of bone regeneration. Bioabsorbable membranes are either natural (xenogeneic collagen type I or III), which undergo resorption by enzymatic degradation, or made of synthetic polymers, which, when inserted in an aqueous environment, undergo enzymatic degradation by hydrolysis [40]. With bioabsorbable membranes, the barrier function duration is variable, since membrane degradation depends on many factors, such as the membrane composition, pH, temperature, the polymer crystallization degree and the cross-linking in collagen membranes, and, therefore, these resorptive processes may vary and interfere with wound healing and, hence, with the bone regenerative outcome [41]. Moreover, due to the lack of stiffness and space-making properties of bioabsorbable membranes, they will collapse into the bone defect or onto the implant threads under the tension of the flap, and thus, the space available for bone regeneration will be occluded. Since crestal defects are usually non-contained defects, the use of a scaffold with either particulate or a bone block replacement graft is a prerequisite for both lateral and vertical bone augmentation procedures [42]. The achievement of primary barrier fixation when the GBR technique is utilized to correct osseous defects is also necessary mainly in large defects, as the percentage of postoperative complications increases when barrier fixation is lacking [43].

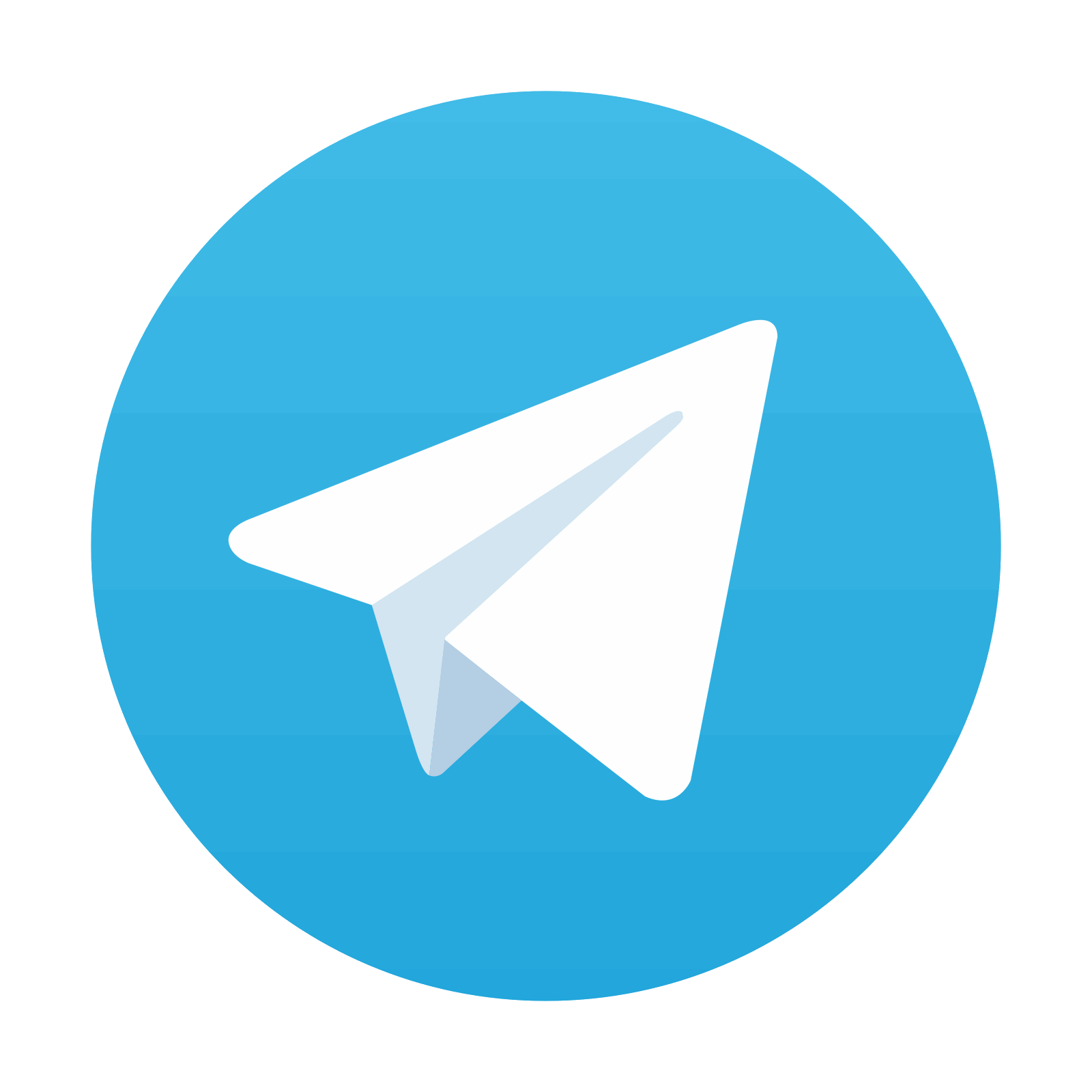
Stay updated, free dental videos. Join our Telegram channel

VIDEdental - Online dental courses
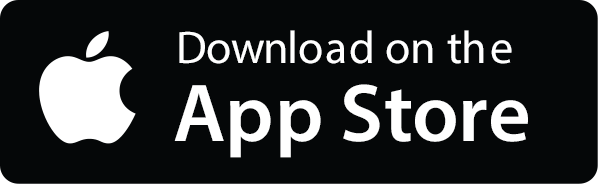
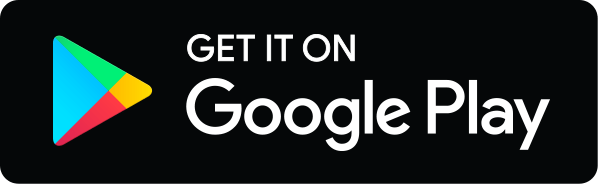