Introduction
The purpose of this research was to demonstrate the potential of the optical coherence tomography technique on the evaluation of changes and damages in the enamel structure caused by debonding and cleanup procedures.
Methods
Twin metal and ceramic brackets were bonded to the labial enamel surfaces of 120 incisors; the brackets were debonded 24 hours later. The anterior surfaces of the teeth were scanned, and optical coherence tomography images were captured in 2-dimensional and 3-dimensional modes before and after debonding and cleanup. A commercial spectral domain optical coherence tomography system with a 5-μm axial spatial resolution was used. The system is based on the Michelson interferometer setup. It is connected to a preconfigured personal computer, and the images are captured with a scanner probe that controls the light beam position at the sample. A total of 960 images were evaluated.
Results
The analysis of the 2-dimensional and 3-dimensional images obtained with optical coherence tomography allows observation and evaluation of adhesive remnants, enamel damage, and superficial aspects of enamel from different methods of adhesive remnant removal. The 2-dimensional optical coherence tomography analysis allows in-depth observation of the adhesive remnant layer.
Conclusions
Optical coherence tomography can be a powerful tool for academic and clinical applications for the evaluation of debonding procedures.
The development of adhesive systems led to the creation of effective methods for bonding orthodontic brackets on enamel surfaces. Although this procedure facilitates assembly of orthodontic appliances, there is a dilemma in its use; the adhesion should be strong enough to prevent bond failure during treatment, but also weak enough so that enamel damage would be nonexistent or minimal during bracket removal after treatment. Diedrich estimated that 150 to 160 μm of enamel loss could occur after debonding. A major concern is to prevent cohesive failures in the enamel during debonding and at the same time to obtain tooth surfaces without adhesive. The procedures of bonding and removal of brackets from the enamel surfaces have a potential risk for cracks, scarring, scratches, or loss of enamel. Moreover, the retention of resin tags is an irreversible alteration of the enamel.
To reduce the rate of irreversible enamel surface damage, several methods of debonding have been suggested. These include conventional methods, in which pliers are used; an ultrasonic method; and electrothermal methods that involve transmission of heat to the adhesive through the bracket. Although all 3 methods have been used successfully to debond brackets, the use of pliers to apply shear or tensile force is perhaps the most convenient method. Alterations in bracket structure and development of debonding instruments and techniques have been made, yet enamel damage during debonding, mainly of ceramic brackets, continues to be a matter of concern for clinicians.
Debondings are mostly examined by optical and scanning electronic microscopes. Scanning, tunneling, and atomic force microscopy techniques have also been explored for dental surface analyses. However, these analyze only the surface. Optical coherence tomography is a well-established, low-coherence interferometric technique that performs high-resolution, ultrafast, noninvasive, and cross-sectional tomographic imaging of tissue microstructures. It can be seen as analogous to ultrasound techniques, in the sense that the analyzed reflected wave from the tissue carries the structural information of the biologic sample. Different from ultrasound equipment, optical coherence tomography uses light instead of sound waves. With the use of broadband sources, such as those generated by ultrashort laser pulses or superluminescent diodes, optical coherence tomography images of biologic tissue can achieve spatial axial resolution of a few micrometers.
Optical coherence tomography systems have been widely explored on ophthalmic applications. Several ophthalmoscopic optical coherence tomography systems are commercially available. Optical coherence tomography has also been used in many other medical fields: eg, dermatology, endoscopy, cardiology, gynecology, oncology, otolaryngology, and urology. One can find many uses for optical coherence tomography in dentistry: anatomic characterization, caries diagnosis, evaluation of restorations and dental materials, marginal adaptations of composite restorations, characterization of periodontal structures, evaluation of the integrity of dental sealants, qualitative markers of plaque bacteria around orthodontic brackets, evaluation of early responses of the periodontal ligament under orthodontic forces, and early detection of oral cancers. However, to the best of our knowledge, no study of optical coherence tomography analysis has been performed on the enamel structure after debonding. By using optical coherence tomography, we can analyze images of resin remnants and enamel surfaces before and after debonding in 2 and 3 dimensions, opening up the possibility for quantitative studies.
Therefore, the purpose of this in-vitro study was to demonstrate the use of optical coherence tomography for the characterization of enamel structure after debonding. Moreover, we report an in-depth analysis of adhesive remnants after the final cleanup procedures.
Material and methods
One hundred twenty extracted incisors acquired from a tooth bank (Pontifícia Universidade Católica do Paraná, Curitiba, Paraná, Brazil) and free from apparent caries, macroscopic cracks, abrasions, and staining (assessed by visual and optical coherence tomography examinations) were selected and stored in 0.1% thymol solution. The teeth were randomly divided into 4 groups ( n = 30) according to the bonded brackets and the pliers used for debonding ( Table ). Each group was equally subdivided into 2 subgroups ( n = 15); for each subgroup, a different procedure was used for residual resin removal (low-speed or high-speed tungsten carbide finishing bur). This experimental procedure was submitted to the ethical committee of the Pontifícia Universidade Católica do Paraná and carried out in accordance with its guidelines in research for human research.
Group | Bracket | Pliers |
---|---|---|
1 | Metallic | Side cutter |
2 | Ceramic | Side cutter |
3 | Metallic | Anterior bracket removal |
4 | Ceramic | Anterior bracket removal |
Before the bonding procedure, the anterior surface of each tooth was examined with optical coherence tomography (Ganymede; Thorlabs, Newton, NJ) in 3-dimensional (3D) and 2-dimensional (2D) modes to obtain the initial images of the intact enamel. Then, for the bonding of the orthodontics appliances, metallic brackets, edgewise standard (American Orthodontics, Sheboygan, Wis), and ceramic brackets, edgewise standard (Morelli, Sorocaba, São Paulo, Brazil), were used. Enamel surfaces were etched with 37% phosphoric acid (Condac 37; FGM, Joinville, Santa Catarina, Brazil) for 20 seconds, rinsed with water for 10 seconds, and air dried. The bonding procedure was performed with Transbond Plus Color Change Adhesive (3M Unitek, Monrovia, Calif) according to the manufacturer’s instructions. The primer was used at the enamel surface, and the adhesive resin was applied on the base of the bracket. The brackets were positioned on the labial surfaces of the incisors, and a dynamometer pressure of 400 g was exerted on the bracket before curing the adhesive to standardize the thickness of the adhesive layer under the bracket. The adhesive was light cured for 20 seconds on the incisal and cervical sides of the bracket with an Ortholux LED curing light (3M Unitek). After bonding, the samples were stored in distilled water for 24 hours.
Debonding was carried out 24 hours after bonding by using side cutter pliers (model i-552; Rocky Mountain Orthodontics, Denver, Colo) and anterior bracket removal pliers (model E-346; Pyramid Orthodontics, Corte Madera, Calif). Although variations in the use of these instruments are possible, debonding was performed with a standardized procedure as follows.
The side cutter was placed diagonally at the bracket base, at the cervical and incisal parts. The bracket was removed by gentle squeezing of the pliers and an additional clockwise rotational movement.
The anterior bracket removal pliers was applied by gripping below the bracket wings at the bracket-enamel interface. By closing and downward tipping of the pliers, a rotational axis was created at the apical bracket margin, thereby releasing the bracket.
After bracket removal, the teeth were again examined by optical coherence tomography, and 3D volumetric images were made (2 images for each tooth). The volumetric images’ scales were 10 × 8 mm for maxillary central incisors and 8 × 6 mm for maxillary lateral incisors, for a broad view of samples. For a more detailed view, a volumetric image, with a scale of 5 × 5 mm, was made for all teeth.
Adhesive removal was carried out with 2 types of burs: a high-speed tungsten carbide finishing bur (model CF375R; Beijing Smart Technology, Beijing, China) and a low-speed tungsten carbide finishing bur (model CB27204; Beijing Smart Technology). The cleanup was performed by 1 operator (J.C.B.L.F.). After that, satisfactory removal of the remnant adhesive and restoration of the enamel surface were verified by optical coherence tomography.
The optical coherence tomography images were made to evaluate the surface of the intact enamel before bonding, after bracket removal, and after removal of any residual resin. A commercial spectral optical coherence tomography system (Ganymede) produced cross-sectional and volumetric optical images ( Fig 1 ). The system is based on the Michelson interferometer setup. It is connected to a preconfigured personal computer, and the images are obtained with a scanner probe. The base unit contains the superluminescent diode light source. The wavelength of the superluminescent diode is centered at 930 nm, with spectral width of 100 nm. With an A-scan rate of 29 kHz, this system can produce 29 frames per second with 512 lines per frame and an axial resolution of 5 μm.
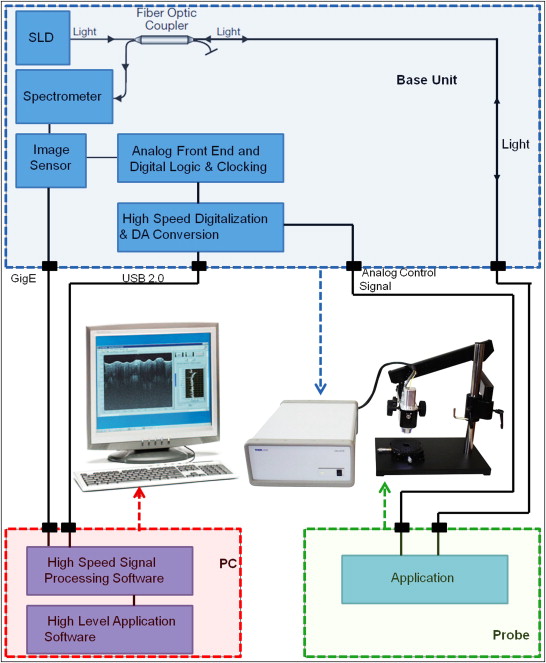
The optical coherence tomography device used in this study produces 2D and 3D images. The 2D scan is obtained by the combination of depth-resolved backscatter signal intensity profiles along the section of interest (A-scans) on the sample. These images allow depth evaluation in the structure of the sample. The optical coherence tomography system produces 3D images by combining several 2D scans of the area of interest, allowing analysis of the surface structure of the sample and in-depth analysis of the scanned structures.
Results
For the evaluation of the samples, the optical coherence tomography images were obtained before bonding, after debonding, and after removal of the remnant adhesive. Images were obtained in both 2D (longitudinal section) and 3D imaging modes.
The initial evaluation of enamel surface structure, examined by optical coherence tomography before the bonding procedure, showed the typical anatomy of this structure. Most surfaces showed the characteristic perikymata and imbrications when using the 3D imaging mode ( Fig 2 , A and B ). Moreover, the 2D imaging mode also permitted measurements of incipient smooth-surface caries lesions and identification of microcavities related to the demineralization process ( Fig 2 , C and D ). These inadequate samples were not used for debonding.
The optical coherence tomography images showed the typical separation of the bracket-resin-enamel interfaces after bracket removal with the side cutter and the anterior bracket removal pliers. Separation occurred mostly at the bracket-adhesive interface but also at the adhesive-enamel interface, leaving varying amounts of resin on the tooth surfaces ( Fig 3 ). Optical coherence tomography also allowed us to verify fractures of bracketing and enamel ruptures after debonding.
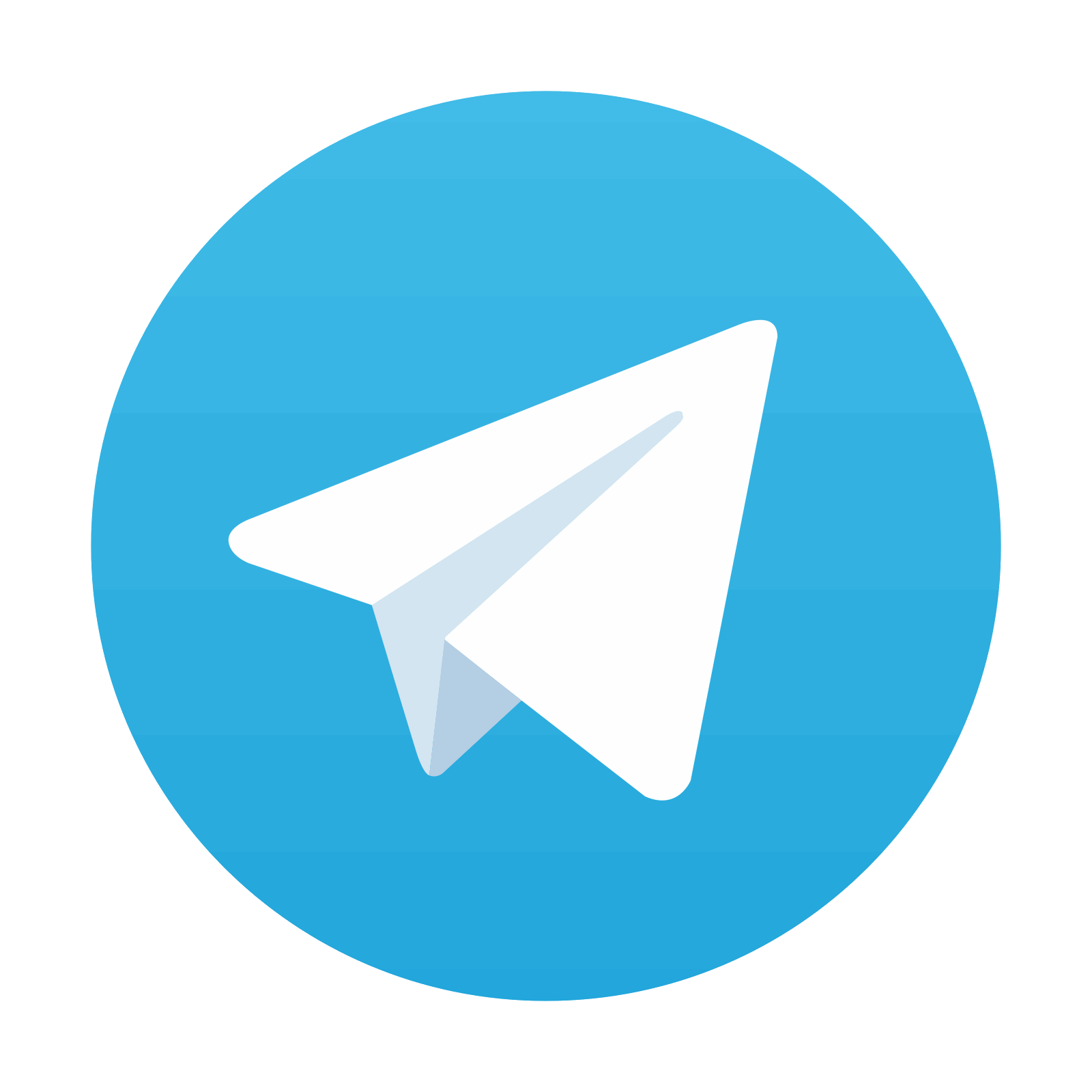
Stay updated, free dental videos. Join our Telegram channel

VIDEdental - Online dental courses
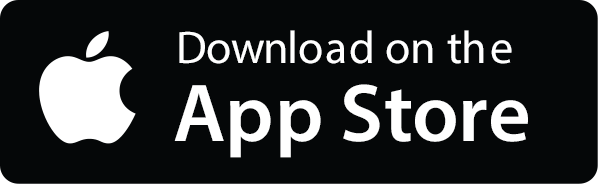
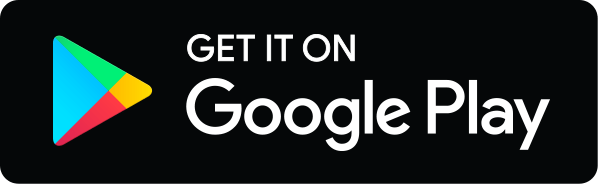
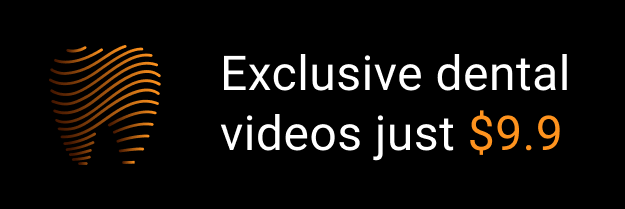