Abstract
Resin bonding to dentin requires the use of self-etching primers or acid etching to decalcify the surface and expose a layer of collagen fibrils of the dentin matrix. Acid-etching reduces the stiffness of demineralized dentin from approximately 19 GPa–1 MPa, requiring that it floats in water to prevent it from collapsing during bonding procedures. Several publications show that crosslinking agents like gluteraladehyde, carbodiimide or grape seed extract can stiffen collagen and improve resin–dentin bond strength.
Objective
The objective was to assess a new approach for evaluating the changes in stiffness of decalcified dentin by polar solvents and a collagen cross-linker.
Methods
Fully demineralized dentin beams and sections of etched coronal dentin were subjected to indentation loading using a cylindrical flat indenter in water, and after treatment with ethanol or ethyl-3-(3-dimethylaminopropyl) carbodiimide (EDC). The stiffness was measured as a function of strain and as a function of loading rate from 1 to 50 μm/s.
Results
At a strain of 0.25% the elastic modulus of the fully demineralized dentin was approximately 0.20 MPa. It increased to over 0.90 MPa at strains of 1%. Exposure to ethanol caused an increase in elastic modulus of up to four times. Increasing the loading rate from 1 to 50 μm/s caused an increase in the apparent modulus of up to three times in both water and ethanol. EDC treatment caused increases in the stiffness in fully demineralized samples and in acid-etched demineralized dentin surfaces in situ .
Significance
Changes in the mechanical behavior of demineralized collagen matrices can be measured effectively under hydration via indentation with cylindrical flat indenters. This approach can be used for quantifying the effects of bonding treatments on the properties of decalcified dentin after acid etching, as well as to follow the loss of stiffness over time due to enzymatic degradation.
1
Introduction
Mineralized dentin contains about 50% mineral by volume. If it is acid-etched with phosphoric acid in preparation for resin–dentin bonding, approximately the top 10 μm of mineralized dentin is completely demineralized . After rinsing off the excess acid, the rinse water extracts the solubilized mineral and leaves the insoluble collagen fibrils floating in approximately 70 vol% water. The goal in adhesive dentistry is to replace that volume of water with adhesive resin monomers. However, the monomer concentration is low (3–4 mol/L) when compared to the water concentration (56 mol/L). Consequently, it is impossible for dentists to remove all of the water using solvated comonomer mixtures in the relatively short period (60–90 s) that most dentists generally allot for monomer infiltration.
The presence of excess residual water in demineralized dentin is problematic. Application of bonding blends that contain dimethacrylates like BisGMA, TEGDMA or UDMA that are insoluble in water, undergo phase changes when they encounter that residual water . Consequently, some collagen fibrils remain infiltrated by water instead of monomers . These collagen fibrils also contain bound endogenous proteases (MMPs and cathepsins) that become uncovered and activated by acid-etching . They are hydrolases that use the residual water to slowly destroy the collagen fibrils, which are critical to anchor the adhesive and resin composite to the underlying mineralized dentin.
If the water is removed from demineralized dentin, there is a rapid, spontaneous development of new interpeptide hydrogen bonds between adjacent collagen peptides. Formation of these bonds increases collagen stiffness and causes shrinkage . Both changes are rapidly reversible if the collagen is rehydrated because the Hoy’s solubility parameter describing the hydrogen bonding cohesive forces ( δ h ) is 40 (J/cm 2 ) 1/2 , which is much greater than that of collagen peptides 14.8 (J/cm 2 ) 1/2 . Water preferentially H-bonds to collagen peptides and prevents interpeptide H-bonds from forming, which is how water plasticizes collagen and synthetic polymers .
Solvents like acetone and ethanol produce reversible interpeptide hydrogen bonds, which also cause reversible increases in matrix stiffness . However, proanthocyanidin agents like Grape Seed Extract (GSE) and tannic acid produce covalent cross-links and irreversible increases in matrix stiffness and strength . Such cross-linking may also make the dentin too stiff to undergo complete shrinkage if the matrix is inadvertently air-dried. The increase in stiffness of the demineralized dentin reflects the degree of interfibrillar cross-linking achieved within the collagen matrix . Consequently, measurements of the changes in matrix stiffness provide a quantitative means for assessing the degree of cross-linking and the treatment’s effectiveness . Recently, Liu et al. showed that addition of 2 wt% GSE to 20 vol% phosphoric acid produced an acid-etched dentin matrix that was apparently stabilized against the action of microbial collagenase within 15–20 s. Nevertheless, they did not measure the increase in stiffness of the demineralized matrix caused by the GSE/phosphoric acid mixture. That would require an approach capable of measuring the stiffness of the etched layer of dentin in situ with adequate sensitivity.
The changes in stiffness of demineralized dentin after application of cross-linkers or other treatments reflects the degree of intermolecular bonding achieved. Thus, quantifying the changes in matrix stiffness is important. The stiffness of demineralized dentin collagen matrices has been measured using atomic force microscopy and flexure methods . While AFM is a potent method for evaluating the stiffness of collagen, it is generally applied to evaluate single fibrils and not the matrix as a continuum . Quantifying the degree of collagen cross-linking requires measures at the meso-scale, not at the fibril level. Existing flexure and tensile testing methods are macro-scale and not capable of measuring the small changes in stiffness of a 1–10 μm layer of demineralized dentin matrix in situ that results from application of polar solvents. Therefore, the purpose of this investigation was to examine a new approach for evaluating the stiffness of demineralized dentin collagen matrices. Here we attempt to perform macroscopic “indentations” with a cylindrical flat indenter to measure reversible and irreversible changes in stiffness of demineralized dentin collagen matrices resulting from application of various clinically-relevant treatments. The null hypothesis of the investigation is that macroscopic indentations are not capable of quantifying the stiffness of decalcified layers of dentin resulting from acid etching in situ .
2
Materials and methods
2.1
Dentin specimens
Human third molars were obtained from the Oral Surgery Clinics of the Georgia Regents University with signed informed consent using a document approved by the GRU Human Assurance Committee. The teeth came from young patients (18–24 yrs of age) and were examined at receipt under light microscopy for signs of caries or cracks. Those teeth without signs of carious lesions or mechanical degradation were stored under refrigeration (4 °C) until ready for use.
The occlusal enamel and superficial dentin were removed using an Isomet saw (Buehler Ltd., Lake Bluff, IL, USA) at right angles to the long axis of the tooth. A second, parallel section was made to create a disk of mid-coronal dentin of either 1.0 or 2.0 mm thick thickness (±0.1 mm). The 2 mm thick disks were further sectioned to prepare beams approximately 3 mm × 2 mm × 5 mm. Three beams and three disks were prepared, all from different teeth. After preparation the samples were stored in 0.9% NaCl containing 0.02% sodium azide to prevent microbial growth.
2.2
Dentin demineralization
To create completely demineralized dentin, single beams or dentin disks were suspended in 10 ml of 10 wt% phosphoric acid (PA) in screw top tubes. Multiple tubes were tumbled at room temperature for 16 h to completely demineralize the specimens after . At the end of demineralization the enamel periphery had been completely removed from the coronal disks. Completely demineralized dentin disks have a modulus of elasticity of ≤4 MPa . Partially demineralized dentin disks have higher values of stiffness (1000–12,000 MPa). The fact that none of our experimental stiffness values reached 4 MPa indicates they were all completely demineralized.
An additional group of 1 mm thick mineralized dentin disks ( N = 5) were prepared and etched using 37% phosphoric acid (Scotchbond, 3M ESPE) to achieve a layer of demineralized dentin of approximately 10 μm. The depth of demineralization was measured using Confocal Laser Scanning Microscopy (CLSM) as described in Scheffel et al. . Specifically, flat dentin surfaces were acid-etched with 37% phosphoric acid for 15 s, rinsed with water for 10 s and then immersed in 1% (w/v) Fluorescein Isothiocyanate (FITC) in DMSO and 1% (w/v) xylenol orange (XO) in water for 5 h. After rinsing with water, the stained disks were examined by CLSM. Exposed collagen fluoresced a green color while underlying mineralized dentin was red, as described in Scheffel et al. . The average thickness of the demineralized layer was 8 μm in Scheffel et al. , but was 10–15 μm in our current study. Many of the teeth had deep occlusal grooves that carried enamel deep into the dentin. When the section is made deep enough to avoid all traces of enamel, the dentin surface is sometimes in deep dentin that contains more tubules than more superficial dentin, allowing etchants to penetrate more deeply into the dentin matrix and increasing the thickness of the demineralized matrix. After completion of the period of etching the samples were rinsed with excess water and then maintained in the 0.9% NaCl solution at room temperature for approximately 1 h.
2.3
EDC treatment
For the 1 mm thick disks of completely demineralized dentin, the stiffness was initially evaluated in the solution of 0.9% NaCl. The samples were then subjected to a cross-linking treatment using an experimental solution of 0.5 M ethyl-3-(3-dimethylaminopropyl) carbodiimide (EDC) in water for 60 s. The dentin stiffness was then measured by indentation as the samples were maintained within a droplet of the EDC treatment solution. Similarly, for the fully mineralized dentin disks that were subjected to acid etching of the surface, the stiffness of the etched layer of dentin collagen was evaluated in situ under hydration with the 0.9% NaCl solution. Then the samples were subjected to 0.5 M EDC crosslinking treatment for 30 s and evaluated again.
2.4
Measurement of stiffness
Measurement of the stiffness was performed using a commercial universal testing machine (BOSE, Model Electroforce 3100, Eden Prairie, MN, USA). The system was equipped with a special load cell with full scale range of 2.5 N and sensitivity of 0.01%. This system has a resolution in displacement control of 1 μm and a resolution in displacement measurement of 0.1 μm. Loading of the demineralized dentin was performed in indentation mode (compression) as shown in Fig. 1 using a specially designed stainless steel cylindrical indenter with 1.9 mm diameter and flat face. The demineralized dentin specimens ( Fig. 1 a) were placed on a loading platform of UHMW polyethylene with the pulp side down after Walton et al. . The specimen was covered within a droplet of 0.9% NaCl containing 0.02% sodium azide to maintain the hydration of the specimen during the indentation process.
To achieve loading of the demineralized samples, the indenter was lowered within the droplet until developing a preload of 2 mN. For the beams, the point of contact was centered within the 3 mm beam width and along the 5 mm length. At this point, the system was converted to displacement control actuation and loading was conducted in displacement control to a maximum displacement of 20 μm. Loading was conducted at displacement rates of 1, 10 or 50 μm/s. The acquisition of the load and displacement responses was monitored over the range of displacement. Each sample was loaded in three different locations over the surface area. After evaluation in water, the samples were placed in a bath of ethanol and maintained with agitation for a period of 30 s. Thereafter, the samples were placed on the UHMW polyethylene stage covered with a droplet of ethanol. The samples were loaded as previously described using the BOSE system at one of the three displacement rates. Following completion of the ethanol treatment and loading, the samples were returned to the 0.9% NaCl solution and maintained at room temperature. After approximately 15 min the samples were evaluated again within a droplet of the NaCl solution using the aforementioned universal testing system and loading approach. Contact loading of the demineralized disk was conducted in exactly the same manner before and after EDC treatment.
For the etched mineralized dentin samples, contact loading was performed to evaluate the stiffness of the thin demineralized layer in situ ( Fig. 1 b). Due to the limited depth of the demineralized layer (ca. 10–15 μm) and range of the load cell (2.5 N), displacement control loading was conducted at a rate of 1 μm/s to a maximum displacement of 10 μm. Following completion of the tests, the samples of water-saturated demineralized dentin were subjected to cross-linking using the EDC treatment for 30 s and loaded again via cylindrical indentation in the EDC solution. Three indentations were performed on each sample.
For both sample configurations the load–displacement curves were used in evaluating the indentation stiffness of the demineralized dentin. These quantities were then used to estimate the elastic modulus at various strains of interest. Under cylindrical indentation, the contact pressure (Pr) can be described as a function of penetration depth ( h ) using Sneddon’s solution for indentation of an elastic half-space by a flat-ended cylindrical punch according to
P r = 2 E h π a ( 1 − ν 2 )
where E , a and ν are the elastic modulus, indenter radius and Poisson’s ratio, respectively. Expanding the contact pressure in terms of the contact force ( P ) and area, the apparent elastic modulus can be estimated in terms of the indentation stiffness ( k = P / h ) according to
E = k ⋅ ( 1 − ν 2 ) 2 a
In the case of a thin demineralized layer of collagen developed by etching of mineralized dentin, the elastic modulus of the demineralized section is substantially lower than that of the mineralized portion. For small displacements that are limited to the demineralized layer thickness, the reaction force is attributed to the demineralized collagen layer only. Thus, the elastic response can be evaluated using Eq. (2) . However, the thin layer of hydrated collagen is restrained by the fully mineralized dentin foundation through the collagen fibril anchors. Hayes et al. developed a model for indentation of layered substrates to be applied in assessing the elastic modulus of articular cartilage, which is described by
E = k ⋅ ( 1 − ν 2 ) 2 a ⋅ β a / h , ν
where the term <SPAN role=presentation tabIndex=0 id=MathJax-Element-4-Frame class=MathJax style="POSITION: relative" data-mathml='βa/h,ν’>β(a/h,ν)βa/h,ν
β a / h , ν
corresponds to a corrective term that accounts for the restraint of the compressible material. In considering the dimensions of a thin layer of demineralized dentin that results from acid etching, it could be considered a thin film. Yang derived an expression for indentation of a compressible elastic thin film by cylindrical flat punch, where the elastic modulus is given by
E = k 1 + ν 1 − 2 ν t 1 − ν π a 2
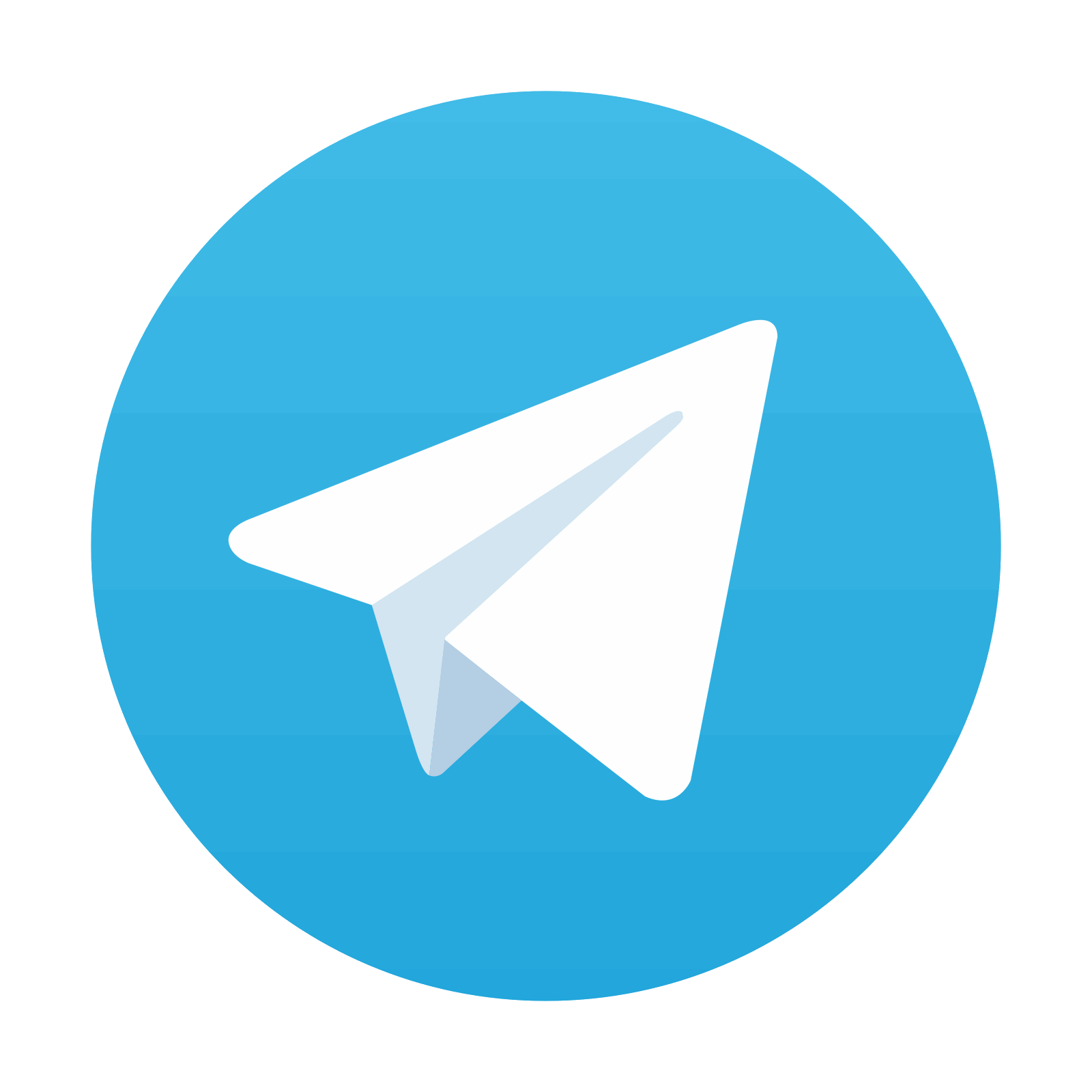
Stay updated, free dental videos. Join our Telegram channel

VIDEdental - Online dental courses
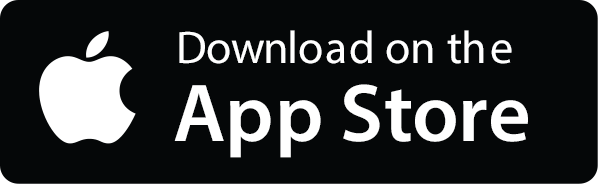
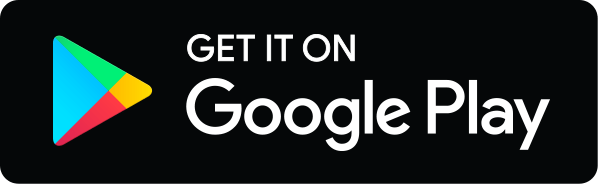