Abstract
This study was designed to investigate whether bone regeneration by implantation of octacalcium phosphate and porcine atelocollagen composite (OCP/Col) would be enhanced if mechanical stress to the implanted OCP/Col were alleviated. OCP/Col discs were implanted into an arc-shaped mandibular defect in male adult beagle dogs divided into untreated, OCP/Col, and OCP/Col/Mesh groups. In the OCP/Col/Mesh group, mechanical stress towards the implanted OCP/Col was alleviated by a titanium mesh. Bone regeneration in the three groups was compared after 6 months. Macroscopically, the alveolus in the OCP/Col/Mesh group was augmented vertically more than in the other two groups. Morphometric analysis by micro-CT showed the bone volume in the OCP/Col/Mesh group was significantly greater than in the other two groups. The augmented alveolus in the OCP/Col/Mesh group consisted of outer cortical and inner cancellous structure. Histologically, the OCP/Col/Mesh-treated alveolus was augmented by matured bone tissue along the inside of the titanium mesh. The implanted OCP/Col in the OCP/Col/Mesh and OCP/Col groups had almost disappeared. These results indicated that vertical bone regeneration by OCP/Col was efficient and successful when the mechanical stress to the implanted OCP/Col was alleviated. OCP/Col should be a useful bone substitute with active structural reconstitution.
Achieving bone regeneration for bone defects is a crucial issue in oral and orthopaedic surgery. Many cases require bone regeneration, for example, reconstruction of bone defect after extirpation of jaw tumours and cysts, bone grafting for alveolar cleft, and preprosthetic surgery of sinus lifting for bone deficiency. Autogenous iliac bone grafting is the most popular bone regenerative technique for large bone defects that cannot be expected to exhibit self-restoration. This technique has several drawbacks, such as limited quantity of harvested bone and excessive surgical intervention into healthy tissue, as well as low availability for general dental practitioners. Therefore, many bone substitutes have been developed as alternatives to autogenous bone grafting, and hydroxyapatite (HA) and β-tricalcium phosphate (β-TCP) have been applied in clinical contexts. Autogenous bone grafting is still the gold standard because HA and β-TCP did not accomplish bone regeneration, in contrast to autogenous bone grafting.
Interstitial bone material consists of organic matrices, such as collagen, and minerals whose prototype is considered to be HA. Octacalcium phosphate (Ca 8 H 2 (PO 4 ) 6 ·5H 2 O; OCP) is considered to be a direct precursor of HA, and the transition of OCP to HA is likely to be spontaneous and irreversible under physiological conditions. The osteoconductive property of synthetic granular OCP was first seen in the subperiosteal implantation of mouse calvaria, and the implanted OCP was converted to the apatitic phase. As OCP was capable of inducing osteoblastic cell differentiation in a manner comparable to HA, the implanted granules of OCP are resorbed and replaced by newly formed bone to a greater extent than those of β-TCP and HA.
Even though OCP possesses many desirable properties as a bone substitute, it cannot be moulded using sintering processes, unlike bioceramics such as HA or β-TCP, because of its intrinsic crystal structure. When considering clinical use, it is easy to assume the limited availability of granular OCP as well as that of granular HA or β-TCP. To resolve these disadvantages, a composite sponge constructed of synthetic OCP and porcine atelocollagen (OCP/Col) was developed. The authors’ previous studies reported that OCP/Col significantly enhanced bone regeneration more than the implantation of OCP alone, β-TCP collagen composite (β-TCP/Col), or HA collagen composite (HA/Col), if implanted into a critical-sized calvarial defect rat model. The efficacy of bone regeneration by OCP/Col was confirmed in various canine bone defect models.
It has been accepted that the three main components for bone regeneration are stem cells or osteoprogenitors, an appropriate biological scaffold, and signal molecules, and the importance of mechanical stress in bone regeneration has been advocated. Bone is a dynamic tissue that constantly undergoes remodelling even once growth and modelling of the skeleton have been completed, and the cells responsible for bone remodelling are known as osteocytes, osteoblasts, and osteoclasts. Osteocytes have the ability to communicate with osteoblasts through an extensive network of cellular processes, and this network is thought to sense and respond to local mechanical stress.
Although implantation of OCP/Col showed prominent bone regenerative properties at a site with minimal stress, limited bone regeneration with stimulation of osteoclastic resorption was demonstrated at mechanically stressed bone defects. The bone regeneration by OCP/Col would be recovered if the mechanical stress were alleviated by the use of support material. It has been reported that the alveolar defects of adult dog were augmented when a micro-titanium mesh was used to alleviate mechanical stress. The present study was designed to investigate whether bone regeneration by implantation of OCP/Col would be enhanced if mechanical stress to the implant were alleviated. With the goal of clinical use, a critical-sized mandibular defect was prepared for an adult dog, and discs of OCP/Col with titanium mesh that alleviates mechanical stress were implanted into the defect. After 6 months of implantation, bone regeneration by OCP/Col with and without titanium mesh was compared.
Materials and methods
Preparation of OCP and OCP/Col discs
Preparation of the discs of OCP and OCP/Col has been described previously. Briefly, OCP was prepared by direct precipitation, and collagen was prepared from NMP collagen PS (Nippon Meat Packers, Tsukuba, Ibaraki, Japan), a lyophilized powder of pepsin-digested atelocollagen isolated from porcine dermis. The sieved granules (particle size 300–500 μm) of OCP were added to the concentrated collagen and mixed, and the weight percentage of OCP in OCP/Col became 77%. The OCP/Col mixture was lyophilized, and the disc was moulded (9 mm diameter, 1 mm thick). The moulded OCP/Col underwent dehydrothermal treatment (150 °C, 24 h) in a vacuum drying oven and was then sterilized using gamma-ray irradiation (5 kGy). Under standardized conditions (60 kV, 10 mA, 11 s), the OCP/Col discs before implantation showed no radiopacity.
Animals and implantation procedures
17-Month-old, male beagle dogs ( n = 9; NARC Co., Chiba, Japan) were used. The principles of laboratory animal care were followed as well as national laws, and all procedures were approved by the Animal Research Committee of Tohoku University (21-Dentistry Animal-23).
General anaesthesia was administered with intravenous sodium pentobarbital (0.5 ml/kg), followed by intramuscular atropine sulfate (0.5 mg) and ketamine hydrochloride (20 mg/kg). After disinfection of the oral cavity, local anaesthesia (2% lidocaine with 1/80,000 epinephrine) was injected. The preparation of left mandibular defect was as follows: at first, left lower second and third premolars were extracted and a buccal intracrevicular incision was made from the centre of the first premolar to the fourth premolar. A vertical incision was made from the marginal gingiva of the fourth premolar towards the inferior border of the mandible, and the mucoperiosteum of the first to fourth premolars of the mandible was ablated in circumference. An arc-shaped defect (about 10 mm radius) was made from the interalveolar septum of the first and second to the third and fourth premolars. The middle point of 20 mm of the alveolar crest between the extracted second and third premolars was point M. The point 10 mm away from point M, which was on a perpendicular line vertically towards the mandibular border from point M was defined the bottom of the defect ( Figs. 1a and 2a ). After preparation of the defect, radiographs were taken to ensure that there were no remaining teeth in the defect, and the mucoperiosteal flap was advanced to cover the defect.


The experimental animals were divided into three groups, and each group included three samples. Group 1 was untreated ( Figs. 1a and 2a ); nothing was implanted into the prepared mandibular defect. Group 2 was the OCP/Col group ( Figs. 1b and 2b ); as many OCP/Col discs as possible were implanted into the prepared mandibular defect, the average was 14.7. Group 3 was the OCP/Col/Mesh group ( Figs. 1c and 2c ); the remaining mandible was surrounded by titanium mesh (0.1 mm thick, hole spacing 0.75 mm × 1.3 mm, Flexgitter 1.0; Synthes ® , Tokyo, Japan) to alleviate mechanical stress on the implants, and the mesh was fixed to the mandible using two titanium mini-screws, as many OCP/Col discs as possible were implanted into the prepared mandibular defect, the average was 12.7. Finally, all of the experimental defects were closed watertight ( Fig. 2 d). To prevent infection, flomoxef sodium was used via an intravenous drip during the operation, and cefcapene pivoxil hydrochloride hydrate was administered orally for 3 days postsurgically. A soft diet was fed to rest the operative wounds during the experimental period.
Tissue preparation and radiography
Dental radiographs were taken by dental radiography (SANKO X-ray MFG or MAX-DC70) with instant film for occlusal radiography (Hanshin Technical Laboratory, Ltd., Nishinomiya, Hyogo, Japan) under standardized conditions (50 kV, 10 mA, 0.8–1.0 s or 60 kV, 10 mA, 0.1 s) immediately after the operation and every month thereafter to confirm the condition of the treated defect. 6 months after implant surgery, the dogs were killed by intravenous injection of an overdose of sodium pentobarbital. The mandible and surrounding tissues were resected and fixed with 10% formalin neutral buffer solution, pH 7.4.
Micro-CT examination
The morphological and quantitative image analysis of newly formed bone was performed using micro-computed tomography (CT) (Scan Xmate-E090; Comscantecno Co., Ltd., Kanagawa, Japan) under standardized conditions (90 kV, 110 μA). In the three-dimensional analysis, the newly formed bone area was analyzed after sampling using bone mineral density (BMD) standards, established as over 300 mg/cm 3 (between 300 mg/cm 3 and 1500 mg/cm 3 ), and the extracted range of bone was defined between 300 mg/cm 3 and 1100 mg/cm 3 .
An area of interest was defined and the bone volume in the area of interest was quantified as follows ( Fig. 1 d). The upper border defined as a curved surface was the line from the distal cement–enamel junction of the first premolar (A) to the mesial cement–enamel junction of the fourth premolar (B). The lower border was defined as the inferior mandibular border. The mesial border defined as a curved surface was the line from A through the root apex of the canine tooth (C) towards the inferior mandibular border, and the intersection was defined as E. The distal border was the tangential line from B through the mesial root border of the fourth premolar (D) towards the inferior mandibular border, and the intersection was defined as F. An area enclosed by A, E, F, and B was defined as the area of interest, then the bone volume was obtained by integrating the area of interest from the buccal to the lingual side. The bone volume in the operated site was measured using a morphometric programme of TRI/three-dimensional BON (Ratoc System Engineering, Osaka, Japan). The height and width of the treated mandible were measured and statistically analyzed. The measured plane was the frontal plane at the centre between A and B, which was vertical to the inferior border of the mandibular.
Statistical analysis
Statistical analysis was performed for all of the micro-CT data using Excel v.X. (Microsoft Co., Redmond, WA, USA). All values are reported as the means ± standard error (SE). The χ 2 test was applied to test whether each group had a normal distribution, and Bartlett’s test was used to examine for homogeneity of variances across samples. One-way analysis of variance (ANOVA) or the Kruskal–Wallis test was used to compare the means among groups. Statistical significance was accepted at P < 0.05. If significant differences of mean values were detected, Tukey–Kramer or Scheffe multiple comparison analysis was used as a post hoc test.
Contact microradiogram and histology
The specimens were fixed in 70% ethanol, stained with Villanueva bone stain, dehydrated in graded ethanol, and embedded in methyl methacrylate. They were sectioned coronally using a low-speed saw machine (Isomet 5000; Buehler, Lake Bluff, IL, USA) with a diamond-wafering blade. The sectioned wafers were mounted on plastic slides and were ground and polished until they were 200–300 μm thick. Contact microradiography of undecalcified sections was carried out with a microradiography unit (Softex CMR Unit; Softex, Tokyo, Japan) for 60 s exposures, at 20 kV and 5 mA. After radiographs were taken, the polished wafers were ground and polished to 20–30 μm thickness and observed with a photomicroscope (Leica DFC300 FX, Leica Microsystems Japan, Tokyo, Japan).
Results
Macroscopic analysis
In the OCP/Col/Mesh group, the operated site was apparently augmented and bone-like hard tissue was palpated at the treated site; exposure of titanium mesh on the treated alveolar ridge was confirmed in two of three animals. In these cases, the alveolar ridge between the first and fourth premolars became thinner and frayed 1 month after operation. Although the area of exposed mesh was slightly increased with time, infectious symptoms such as swelling and pus discharge were hardly seen, and no sequestration was observed. In OCP/Col and untreated groups, the operated site was arc-shaped, and the atrophied alveolus was covered by healthy oral mucosa after 6 months of operation. No inflammatory and infectious symptoms were observed in either experimental group.
Chronological radiographic examination
Immediately after operation, the radiopaque bottom of the defect was similarly positioned in every group, and radiopacity in the OCP/Col implanted area was not clear without mesh-like radiopacity that corresponded to titanium mesh ( Fig. 3 ). In the OCP/Col/Mesh group, a foggy radiopaque figure was observed in the implanted area at 2 months after operation, but it was distant from the top of the titanium mesh. At 6 months, the treated area was augmented with radiopacity that was similar to original bone. In the OCP/Col group, the radiopaque bottom of the defect was increased about 3–4 mm 2 months after operation, and a foggy radiopaque figure was observed at the OCP/Col implanted area. Subsequently, it increased and came to resemble original bone, whereas the bottom of the defect was stationary. In the untreated group, the radiopaque bottom of the defect was increased about 2–3 mm, immediately to 2 months after the operation; thereafter, no significant change was observed.

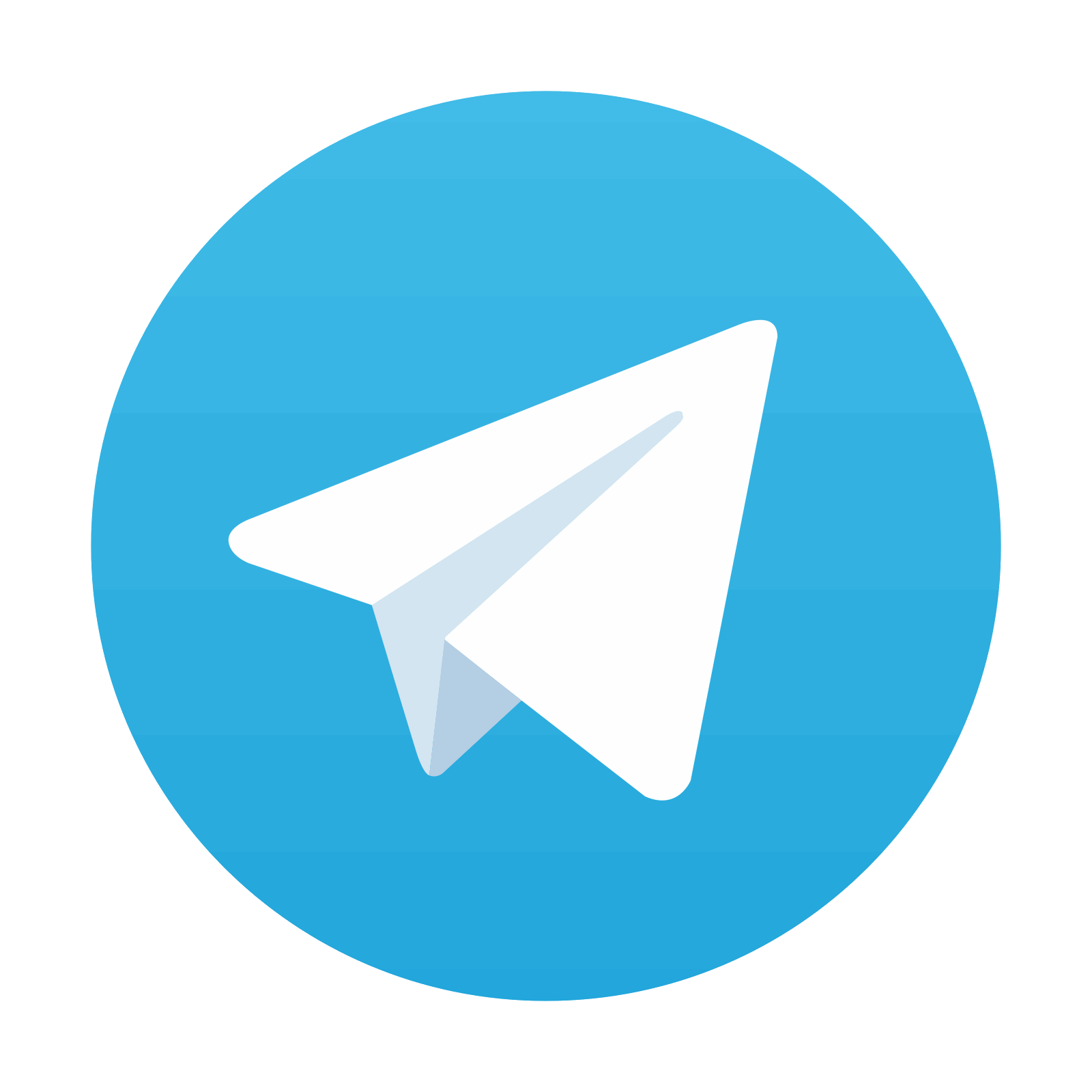
Stay updated, free dental videos. Join our Telegram channel

VIDEdental - Online dental courses
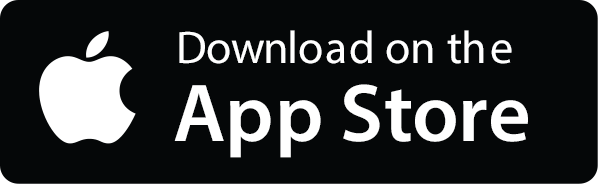
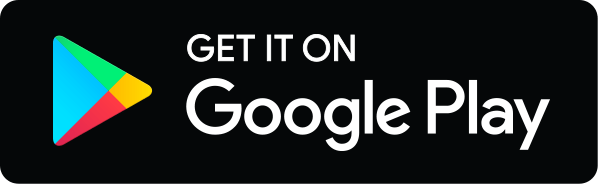
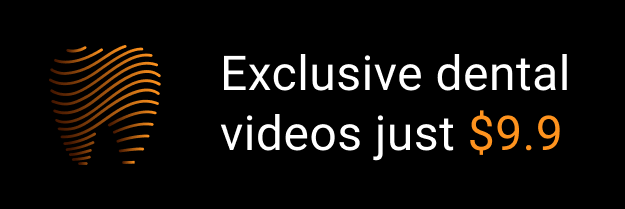