Introduction
The objective of this research was to analyze the effect of orthodontic treatment with maxillary permanent first molar extraction on the occlusal stress distribution and displacement in the human skull.
Methods
A 3-dimensional finite element model was constructed on the basis of a computed tomography scan, and it was used as the pretreatment model. The software used for geometric modeling was Solid Works (Dassault Systèmes, Paris, France). For the extraction model, the maxillary permanent first molar was removed, followed by a repositioning of the anterior and posterior segments to create a space closure model. Stress distribution was evaluated under the simulation of 1000 N for occlusal forces and 400 N for masseter muscle force.
Results
The highest von Mises stress was observed at the zygomatic process of the temporal bone across all 3 models (25 MPa), whereas stress at the pterygomaxillary suture area was almost 50% less. However, the stress in the pterygomaxillary suture area was lowest in the extraction model (18%) and space closure (30%). Stress in the zygomatic process of the frontal bone and frontal process of the maxilla increased from pretreatment to extraction model followed by space closure model.
Conclusions
The occlusal forces were transferred through maxillonasal, maxillozygomatic, and maxillopterygoid stress trajectories. The mesial displacement of the molars may weaken the role of maxillopterygoid stress trajectory while strengthening the role of maxillonasal stress trajectory.
Highlights
- •
Finite element analysis is an advanced and precise tool in biomechanical research.
- •
The pattern of occlusal load distribution reflects the functional morphology.
- •
And prevents local bone damage caused by excess masticatory load and orthodontic forces.
- •
Mesial displacements of the molars weaken the role of the pterygoid stress trajectory.
- •
Dehiscence and fenestration can occur if the bone does not adapt physiologically to the local stress.
The occlusal forces during mastication are transferred through the teeth, periodontal ligaments (PDLs), and the alveolar bone to the supporting structures of the skull. Theoretical fundaments of biomechanics explain the skull architecture on the basis of bone pillars in the maxilla and trajectories of stress dissipations in the mandible.
According to Sicher, the masticatory forces are dissipated from the alveolar process to the 3 enhanced bone pillars in the maxilla, located at each antimere and bypassed by nasal and orbital cavities. The canine and zygomatic pillars (ZPs) are horizontally connected along with the supra and infraorbital edges, which act as beams that resist the mechanical stresses. The pterygoid pillar is an enhanced bone arched toward the skull base and hard palate, connecting the pillar systems on each side of the skull. The nature of strain and stress distribution helps in better understanding of diagnosis and treatment of stomatognathic diseases and reconstruction of masticatory function.
It is generally accepted that the maximal occlusal force is higher in molars than incisors. There is an inherent relationship between key ridge, infrazygomatic crest, and the position of the first molar.
Recent dental health surveys in the United Kingdom have shown an increase in the incidence of decay associated with the first molar, and it also significantly display enamel hypoplasia. , As a general rule, the compensating extraction of a maxillary first permanent molar has been recommended when extraction of the mandibular first permanent molar is required. This is to avoid supraeruption of an unopposed maxillary first permanent molar, which can prevent desirable mesial movement associated with the erupting mandibular second permanent molar and other occlusal interferences, whereas balancing extraction of sound first permanent molar has been recommended to preserve arch symmetry. ,
In certain occlusions, when space is not required to reduce overjet or relieve crowding, it may be beneficial to extract the first molar, which favors mesial eruption of the second molar into extraction space.
In the finite element study by Cattaneo et al, the forward and backward displacements of the maxillary molars from the infrazygomatic crest affected the pattern of load transfer through the maxillary complex. Thus, it becomes important to determine the areas of stress distribution in patients with missing or extracted maxillary first molars.
The finite element analysis (FEA) is the most advanced and precise tool in biomechanical research. It is the ultimate method for modeling complex structures and analyzing their mechanical properties. It allows establishing the location, magnitude, and direction of an applied force, as it may also assign stress points that can be theoretically measured. Furthermore, it does not affect the physical properties of the analyzed materials.
However, to date, there have been no studies dealing with the stress distribution and displacement after orthodontic treatment with maxillary first permanent molar extraction. The purpose of this study is to evaluate and analyze the effect of orthodontic treatment with maxillary permanent first molar extraction on the occlusal stress distribution in the skull by FEA.
Material and methods
A 3-dimensional (3D) FEA model of a human skull was produced on the basis of computed tomography (CT) scan data of a healthy 15-year-old male patient with no craniofacial anomaly. The CT scan was taken for study with a 3-mm scan increment and a 1-mm slice thickness. The model had a set of all permanent dentition with normally erupted teeth and maxillary permanent first molar with a poor prognosis on the right side. The protocols of this study were approved by the ethics committee of the institute (IEC/2017-18/02). The CT scan was read into image processing software (Mimics, version 14.0; Materialise, Leuven, Belgium). The images were segmented by thresholding to obtain the right skull and maxillary teeth. The 3D reconstruction of these parts was then formed with a surface triangularization technique ( Fig 1 ). This reconstruction formed the basis for the so-called pretreatment model. The triangularized shapes of the bone were subsequently read into image processing software to manipulate the molar position. The 3D models were assumed to have maxillary teeth, PDLs (0.25-mm thick), and cortical and cancellous bone thickness of 1-mm thick. CT scan images of the maxilla, including the skull, are made with a top cutting plane. The physical model of brackets was scanned at Advance CAD Technologies Pvt Ltd (Pune, India), using REXCAN III 3D white light scanner (Solutionix, Seoul, South Korea). The computer-aided designing model with proper details about the brackets and teeth were obtained. The obtained computer-aided designing model was used to construct the geometric model of the teeth with the bonded brackets in Geomagic Design X software (3D Systems Manufacturing, Rock Hill, SC).

The software used for geometric modeling and to import individual CT scan sections and traced was Solid Works (Dassault Systèmes, Paris, France). Individual CT scan sections were imported into the Solid Works software and traced, taking care not to disturb the anatomy of the region. These blocks were imported into HyperWorks software (version 13.0; Altair Engineering, Troy, Mich).
The study aimed to determine the occlusal stress distribution and displacement in the human skull after permanent maxillary first molar extraction. For the boundary conditions, the movement was restricted in the top cutting plane.
The von Mises stress and the normal stress were evaluated under the simulation of 1000 N for the occlusal force on the maxillary right teeth and the simulation of 400 N for the masseter muscle force, which originated at the inferior part of the zygomatic arch and inserted into the lateral surface of the ramus of the mandible, before and after extraction of maxillary permanent first molar ( Table I ). To produce the extraction model, the permanent maxillary right first molar was removed. Then the anterior segment was repositioned back by 4.1 mm and the posterior segment forward by 6.9 mm on the sagittal plane ( Fig 2 ). Buccolingual minor repositioning and rotation of the anterior and posterior dental segments were done to maintain the continuous curvature of the dental arch. The stress was compared at 4 points within the main stress trajectories: the frontal process of the maxilla (FM), the zygomatic process of the frontal bone (ZF), the zygomatic process of the temporal bone (ZT), and the pterygomaxillary suture area (PM).
Force application point | Model | ||
---|---|---|---|
Pretreatment | Extraction | Space closure | |
Central incisor | 20 | 20 | 20 |
Lateral incisor | 20 | 20 | 20 |
Canine | 20 | 20 | 20 |
First premolar | 60 | 120 | 120 |
Second premolar | 60 | 140 | 140 |
First molar | 370 | – | – |
Second molar | 450 | 680 | 680 |
Total occlusal force | 1000 | 1000 | 1000 |
Masseter muscle | 400 | 400 | 400 |

The linear FEA method has been used, and all the materials were assumed to be isotropic, homogenous, and linearly elastic. The total number of nodes and elements in the pretreatment model were 207,148 and 1,072,185 for teeth, 98,532 and 145,986 for a PDL, 138,225 and 680,737 for alveolar bone and skull bone. In the case of the extraction model, a total number of nodes and elements were 167,902 and 869,724 for teeth, 92,654 and 134,864 for a PDL, 138,225 and 680,737 for alveolar bone and skull bone, 11,802 and 45,587 for the bracket, 2653 and 980 for archwire whereas in space closure model, 167,902 and 869,724 for teeth, 92,654 and 134,864 for a PDL, 147,231 and 694,632 for alveolar bone and skull bone. The space closure model had a higher number of nodes and elements than the other 2 models. After extraction of the first molar, as the second molar moved anteriorly, these geometric changes in the position of the second molars led to the reconstruction of the space closure model with highly refined mesh. The precision of the FEA calculation increases as highly refined meshes of the model are used. The highly refined mesh is required in critical areas such as areas with a higher stress concentration and zones of complex and highly curved geometric shapes within the model. Hence, a mesh convergence study was recommended. The space closure model was reanalyzed using a 10-noded tetrahedral element to ensure convergence in the pattern of stress; therefore, discretization error can be well controlled to get more accurate results with a reasonable model dimension. The material properties of the bone and teeth in the model were defined according to experimental data from previous studies. , ( Table II ).
Material | Young’s modulus (MPa) | Poisson’s ratio |
---|---|---|
Teeth | 20,000 | 0.3 |
Cortical bone | 13,700 | 0.3 |
Trabecular bone | 1370 | 0.3 |
Stainless steel archwire | 200,000 | 0.3 |
PDL | 0.13 | 0.45 |
Brackets | 193,000 | 0.35 |
Buccal tube with traction arm | 200,000 | 0.3 |
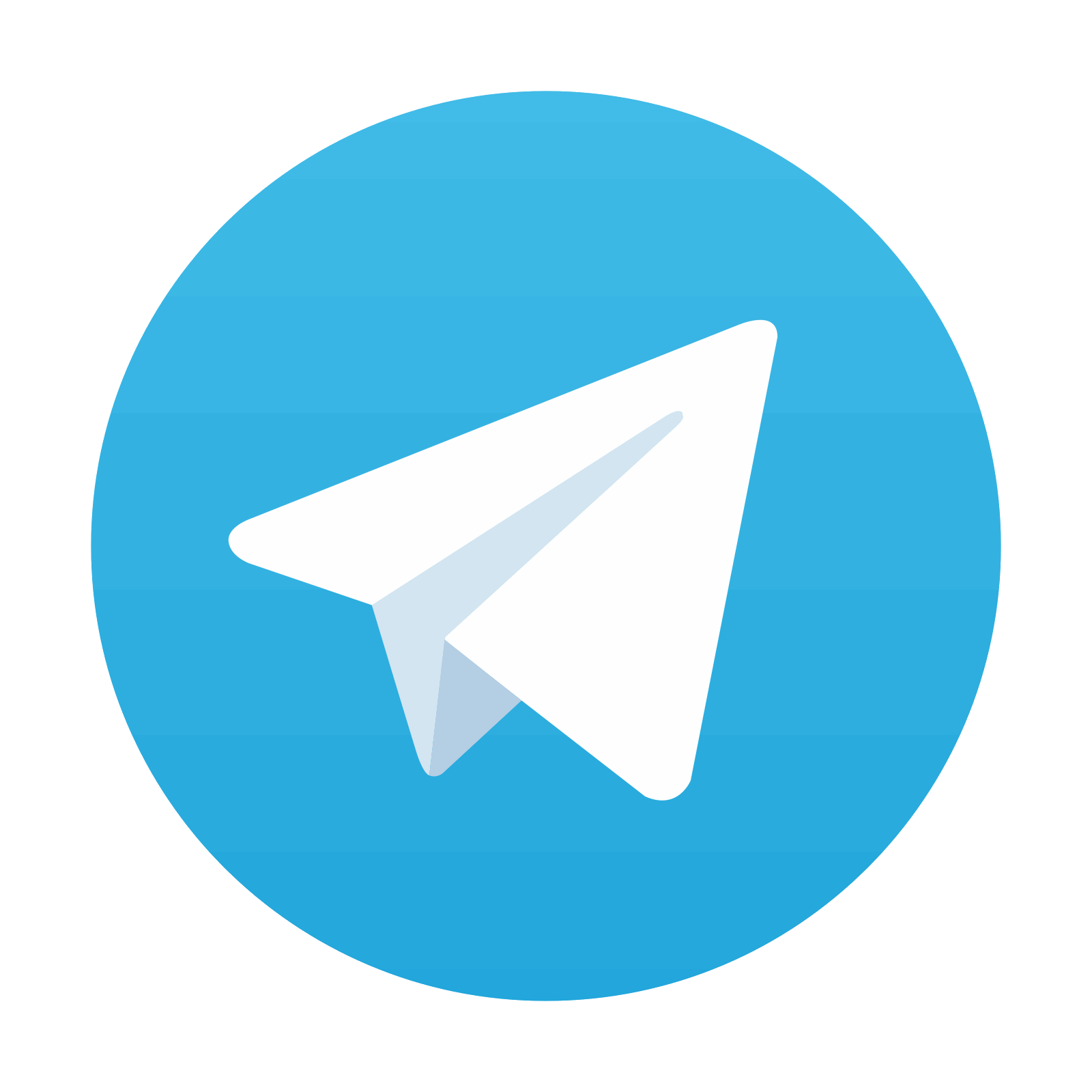
Stay updated, free dental videos. Join our Telegram channel

VIDEdental - Online dental courses
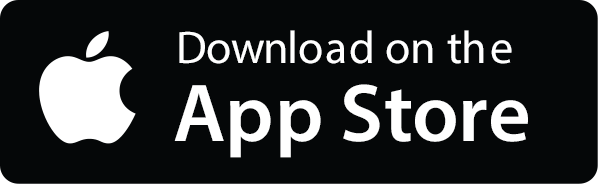
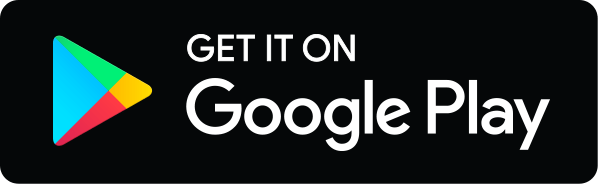
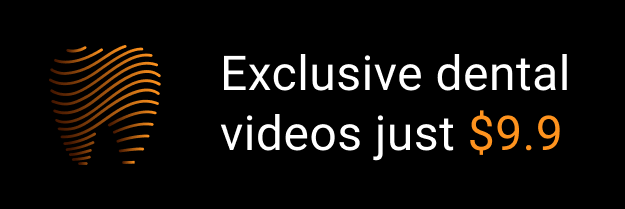