Abstract
Objectives
Dental luting cements are widely used to bond indirect restorations to teeth. Microcracks often lead to cement failures. The objectives of this study were to develop the first self-healing luting cement, and investigate dentin bond strength, mechanical properties, crack-healing, and self-healing durability in water-aging for 6 months.
Methods
Microcapsules of poly(urea-formaldehyde) (PUF) shells with triethylene glycol dimethacrylate (TEGDMA) as healing liquid were synthesized. Cement contained bisphenol A glycidyl dimethacrylate, TEGDMA, 4-methacryloyloxyethyl trimellitic and glass fillers. Microcapsules were added at 0%, 2.5%, 5%, 7.5%, 8.5%, 9.5% and 10%. Dentin shear bond strength was measured using extracted human teeth. Flexural strength and elastic modulus were measured. Single edge V-notched beams were used to measure fracture toughness (K IC ) and self-healing. Specimens were water-aged at 37 °C for 6 months and then tested for self-healing durability.
Results
Adding 7.5% microcapsules into cement achieved effective self-healing, without adverse effects on dentin bond strength and virgin mechanical properties (p > 0.1). Excellent self-healing of 68%-77% recovery was obtained. Six months of water-aging did not decrease the self-healing efficiency, compared to 1 d (p > 0.1), indicating that the self-healing property did not degrade in water-aging.
Conclusions
A self-healing dental luting cement was developed for the first time. It contained fine microcapsules and exhibited an excellent self-healing efficiency, even after being immersed in water for 6 months.
Clinical significance
The self-healing cement is promising for cementing crowns and bridges and other adhesive cement applications, to heal cracks and increase restoration longevity.
1
Introduction
Dental luting cements play an important role in dentistry to attach indirect restorations including inlays, crowns and bridges to the prepared teeth . Success of indirect restorative procedures depends partly on the cementation technique used to create a critical link between the fixed prostheses and their supporting tooth structures . Although the clinical performance has been improved rapidly, dental cements still have relatively poor physical properties compared to bulk restorative filling materials and are prone to fractures . At the restoration-tooth cemented interfaces or margins, the bond longevity is mainly affected by microcracks induced by polymerization shrinkage, repetitive dynamic mechanical loading, water sorption and thermal fatigue . These microcracks could coalesce under cyclic stresses and eventually cause cement fracture, which is a main reason for the failure of cemented restorations . Therefore, extensive efforts have been undertaken to improve the properties of dental luting agents to increase the clinical service lives. They include modifying the compositions and optimizing the handling properties . However, there have been few reports on inhibiting microcrack propagation to protect the cement’s integrity. An innovative strategy is to develop self-healing materials by incorporating microcapsules . These microcapsules have an intact polymeric shell encapsulating a healing liquid inside. When such a material cracks due to stress, the microcapsules inside the material would be ruptured by the crack. They would then release the healing liquid which flows into the crack planes via capillary action, exposing the healing liquid to the catalyst embedded in the polymer matrix. This causes self-healing due to the polymerization of the healing agent, which fills and bonds the crack together . Dicyclopentadiene (DCPD) was encapsulated in a poly (urea-formaldehyde) (PUF) shell to form microcapsules . Grubb’s catalyst was used in an epoxy matrix to trigger the polymerization of the released DCPD, which achieved an ideal self-healing efficiency of 75% . These DCPD-containing microcapsules were also incorporated in dental resin-based materials . The self-healing resin composite achieved 57% recovery of the original fracture toughness . The incorporation of microcapsules did not decrease the mechanical properties of dental resins . Another study developed polyurethane (PU) shell-based triethylene glycol dimethacrylate (TEGDMA) containing nanocapsules for addition in dental adhesive, but that study did not mention the use of a catalyst and did not report any self-healing data . There has been no further report on the use of DCPD and Grubb’s catalyst in dental materials, likely because the DCPD toxicity, Grubb’s catalyst toxicity, availability and high cost remain as challenges .
More recently, novel self-healing poly(urea-formaldehyde) (PUF) microcapsules containing polymerizable TEGDMA and N,N -dihydroxyethyl- p -toluidine (DHEPT) as healing liquid with no cellular cytotoxicity were synthesized and incorporated into dental resins . These microcapsules were successfully incorporated into antibacterial dental composite containing nanoparticles of amorphous calcium phosphate (NACP) to obtain triple benefits of self-healing, antibacterial, and remineralization capabilities . The long-term self-healing efficiency of this novel self-healing dental composite was also investigated, and six months of water-aging did not decrease the self-healing efficiency, compared to that at 1 day . However, to date, there has been no report on self-healing dental luting cements.
Accordingly, the objectives of this study were to: (1) develop the first self-healing dental luting cement; (2) investigate the dentin bond strength, mechanical properties and healing efficiency; and (3) assess the long-term healing effect after water-aging for up to 6 months. The following hypotheses were tested: (1) Incorporation of self-healing microcapsules into dental luting cement would not compromise the dentin bond strength; (2) Incorporation of the microcapsules would endow the cement with the ability to self-heal cracks and regain load-bearing capability; (3) Water-aging for 6 months would not reduce the self-healing efficiency of the novel cement compared to that at 1 day.
2
Materials and methods
2.1
Synthesis of self-healing microcapsules
Microcapsules were synthesized via in situ polymerization of formaldehyde and urea as previously described . Briefly, DHEPT (Sigma-Aldrich, St. Louis, MO) at 1% (all mass fractions) was added to TEGDMA monomer (Esstech, Essington, PA). At room temperature, 50 mL of distilled water and 13 mL of a 2.5% aqueous solution of ethylene-maleic anhydride (EMA) copolymer (Sigma-Aldrich) were mixed in a 250 mL round-bottom glass flask . The flask was suspended in a water bath on a hotplate (Isotemp, Fisher Scientific, Pittsburg, PA). The EMA solution was used as a surfactant to form an “oil-in-water” emulsion (“oil” being the TEGDMA-DHEPT monomer). Under agitation by a magnetic stir bar (diameter = 7.8 mm, length = 50 mm, Fisher Scientific) at 300 rpm, the shell-forming material urea (1.25 g), ammonium chloride (0.125 g) and resorcinol (0.125 g) (Sigma-Aldrich) were added into the solution. Resorcinol was added in the reaction of shell formation to enhance the rigidity of the shell . The pH was adjusted to 3.5 via the drop-wise addition of 1 M sodium hydroxide solution. Then, the agitation rate was increased to 900 rpm, and 30 mL of the TEGDMA-DHEPT liquid was added into the flask. A stabilized emulsion of fine TEGDMA-DHEPT droplets was formed after 10 min of agitation. Then, 3.15 g of a 37% aqueous solution of formaldehyde (Sigma-Aldrich) was added, and the flask was sealed with aluminum foil to prevent evaporation. The temperature of the water bath was raised to 55 °C and the shell material was isothermally polymerized for 4 h (h) under continuous agitation . In this process, ammonium chloride catalyzed the reaction of urea with formaldehyde to form PUF at the oil-water interface to develop the shell . The microcapsules thus obtained were rinsed with water and acetone, vacuum-filtered, and air-dried for 24 h. The microcapsules were examined by optical microscope (TE2000-S, Nikon, Japan), and their sizes were measured with an image analysis software (Nis-Elements BR2.30, Nikon). In addition, the microcapsules were examined with scanning electronic microscopy (SEM, Quanta 200, FEI, Hillsboro, OR).
2.2
Development of self-healing dental luting cements
Bisphenol A glycidyl dimethacrylate (BisGMA) and TEGDMA (Esstech) were mixed at a mass ratio of 1:1 (termed BT). Then, 1% (all mass fractions) photo-initiator of phenyl-bis (2,4,6-trimethylbenzoyl) phosphine oxide (BAPO, Sigma-Aldrich) and 1% BPO were mixed into BT, which formed the resin matrix for paste A (termed BT part A). For paste B, 0.5% DHEPT was mixed into BT (termed BT part B). This formed a dual-cure system where BAPO was for light-cure and BPO was for chemical-cure. Paste A contained BPO as the initiator, and paste B contained DHEPT as the catalyst. For mechanical reinforcement, barium boroaluminosilicate glass particles with a median particle size of 1.45 μm (Caulk/Dentsply, Milford, DE) were silanized with 4% 3-methacryloxypropyltrimethoxysilane and 2% n -propylamine . For bonding capability, an acidic functional adhesive monomer 4-methacryloyloxyethyl trimellitic anhydride (4-META, Sigma-Aldrich) was added to the resin in addition to glass fillers to form the cement. Paste A contained 40% BT part A + 5% 4-META + 55% glass fillers. Paste B contained 40% BT part B + 5% 4-META + 55% glass fillers.
Furthermore, the BPO in paste A served as the initiator to react with the DHEPT in the healing liquid in the microcapsules to trigger the self-healing polymerization. The following microcapsule mass fractions were incorporated into the final cement (when an equal amount of A and B were mixed): 0% (experimental control), 2.5%, 5%, 7.5%, 8.5%, 9.5% and 10%. Clearfil™ SA cement (Kuraray, Tokyo, Japan) was used as a commercial control. Clearfil™ SA cement also had two parts, containing BisGMA, TEGDMA, and silanated colloidal silica particles as fillers. Furthermore, paste A contained acidic functional adhesive monomer of 10-Methacryloyloxydecyl dihydrogen phosphate (MDP), photo-initiator, BPO and initiator. Paste B contained accelerators, according to the manufacturer.
2.3
Dentin shear bond strength test
Extracted caries-free human third molars were stored in 0.01% thymol solution. Flat mid-coronal dentin surfaces were prepared by cutting off the tips of molar crowns with a 150 μm-thick diamond saw (Isomet, Buehler, Lake Bluff, IL). Each tooth was embedded in a poly-carbonate holder (Bosworth, Skokie, IL) and ground perpendicularly to the longitudinal axis on 320-grit silicon carbide paper until the occlusal enamel was completely removed. A double-stick tape with a central hole of a diameter of 3 mm was used to cover the moist dentin surface, and a stainless-steel iris with a central opening (diameter = 4 mm, thickness = 1.5 mm) was placed against the tape, exposing the 3 mm hole. Each mixed cement paste was placed to fill the opening in the iris. The bonded specimens were light-cured for 60 s (Triad 2000, Dentsply, York, PA). After 1 h, all the specimens were stored in water at 37 °C for 24 h. To measure the dentin shear bond strength, a chisel connected with a computer-controlled Universal Testing Machine (MTS, Eden Prairie, MN) was aligned to be parallel to the cement-dentin interface. A load was applied at a rate of 0.5 mm/min until the bond failed. Dentin shear bond strength = 4P/(πd 2 ), where P is the failure load, and d is the diameter of central hole of the tape .
2.4
Flexural test
Each cement paste was placed into a mold of 2 × 2 x 25 mm and covered with Mylar strips. Specimens were photo-cured (Triad 2000, Dentsply, York, PA) for 1 min on each open side of the mold. After 1 h, the specimens were demolded and stored in water at 37 °C for 24 h. A computer-controlled Universal Testing Machine (5500R, MTS, Cary, NC) was used to fracture the specimens in three-point flexure with a span of 10 mm and a crosshead speed of 1 mm/min . Flexural strength was measured as: S = 3P max L/(2bh 2 ), where P max is the load-at-failure, L is span, b is width and h is thickness. Elastic modulus was measured as: E = (P/d)(L 3 /[4bh 3 ]), where load P divided by displacement d is the slope in the linear elastic region of the load-displacement curve .
2.5
Fracture toughness K IC test and measurement of self-healing efficiency
K IC was measured via a single edge V-notched beam (SEVNB) method . A notch with a depth of approximately 500 μm was machined into the 2 × 2 x 25 mm bar using a diamond blade with a 150 μm thickness (Buehler, Lake Bluff, IL). Then, a 3-μm diamond paste (Buehler, city, IL) was placed into the notch tip, and a new sharp razor blade was used to cut the notch further to a total depth of about 700–800 μm . This method yielded a relatively fresh sharp notch tip . The notch length was measured using an optical microscope on both sides of the specimen and then the notch length was averaged for each specimen. The notched specimens were tested in the same three-point fixture with the notch on the tensile side, and the loading pin was aligned with the notch under a 3 × magnifier. K IC was calculated following an established SEVNB method . This yielded the original virgin K IC of the cement, K IC-virgin .
In order to test the self-healing efficiency, immediately following specimen fracture, the two halves of the specimen were placed back into the mold to ensure a good contact of the two fractured planes. This would be similar to the case of the cement in a tooth-restoration interface, where the cracked cement would stay in the same position to allow the released healing liquid to heal the crack. Since fracture ruptured the microcapsules, the released TEGDMA-DHEPT from the microcapsules would react with the BPO in the matrix. This would cause the polymerization of the released liquid to heal and bond the two cracked planes into one cohesive specimen. This healed sample was incubated in a humidor at 37 °C for 24 h. The notch length was again measured and made sure that it was the same as the virgin notch length of the same specimen. Then the healed bar was tested again using the same flexural method to measure the K IC . This yielded the healed value, K IC-healed . The self-healing efficiency (η) was assessed: η = K IC-healed /K IC-virgin , following a previous study .
The fractured surfaces of selected self-healed specimens were examined via metallographic microscope (BX51 M, Olympus, Japan). In addition, the fractured and unhealed surfaces were rinsed with ethyl alcohol immediately after fracture to remove the released healing liquid, and then the surfaces were also examined via the metallographic microscope.
2.6
Water-aging of self-healing cement specimens
The self-healing cement with 7.5% microcapsules had a flexural strength similar to that with 0% microcapsules, while having a high self-healing efficiency. The cement with 8.5%-10% microcapsules had a relatively lower flexural strength. Therefore, the cement containing 7.5% microcapsules was selected for water-aging test for 6 months. The cured specimens of 2 × 2 × 25 mm were immersed in distilled water at 37 °C for 1 day and 1, 2, 3 and 6 months. For each time period, six specimens were immersed in 200 mL of water in a sealed polyethylene container. The water was changed once every week. After each water-aging time period, the K IC-virgin was measured . Then the two broken halves of each specimen were allowed to heal as described above, and the K IC-healed was measured. This yielded the self-healing efficiency η vs. water-aging time for the cement .
2.7
Statistical analysis
One-way and two-way analyses-of-variance (ANOVA) were performed to detect the significant effects of the variables. Tukey’s multiple comparison was used to compare the data at p of 0.05.
2
Materials and methods
2.1
Synthesis of self-healing microcapsules
Microcapsules were synthesized via in situ polymerization of formaldehyde and urea as previously described . Briefly, DHEPT (Sigma-Aldrich, St. Louis, MO) at 1% (all mass fractions) was added to TEGDMA monomer (Esstech, Essington, PA). At room temperature, 50 mL of distilled water and 13 mL of a 2.5% aqueous solution of ethylene-maleic anhydride (EMA) copolymer (Sigma-Aldrich) were mixed in a 250 mL round-bottom glass flask . The flask was suspended in a water bath on a hotplate (Isotemp, Fisher Scientific, Pittsburg, PA). The EMA solution was used as a surfactant to form an “oil-in-water” emulsion (“oil” being the TEGDMA-DHEPT monomer). Under agitation by a magnetic stir bar (diameter = 7.8 mm, length = 50 mm, Fisher Scientific) at 300 rpm, the shell-forming material urea (1.25 g), ammonium chloride (0.125 g) and resorcinol (0.125 g) (Sigma-Aldrich) were added into the solution. Resorcinol was added in the reaction of shell formation to enhance the rigidity of the shell . The pH was adjusted to 3.5 via the drop-wise addition of 1 M sodium hydroxide solution. Then, the agitation rate was increased to 900 rpm, and 30 mL of the TEGDMA-DHEPT liquid was added into the flask. A stabilized emulsion of fine TEGDMA-DHEPT droplets was formed after 10 min of agitation. Then, 3.15 g of a 37% aqueous solution of formaldehyde (Sigma-Aldrich) was added, and the flask was sealed with aluminum foil to prevent evaporation. The temperature of the water bath was raised to 55 °C and the shell material was isothermally polymerized for 4 h (h) under continuous agitation . In this process, ammonium chloride catalyzed the reaction of urea with formaldehyde to form PUF at the oil-water interface to develop the shell . The microcapsules thus obtained were rinsed with water and acetone, vacuum-filtered, and air-dried for 24 h. The microcapsules were examined by optical microscope (TE2000-S, Nikon, Japan), and their sizes were measured with an image analysis software (Nis-Elements BR2.30, Nikon). In addition, the microcapsules were examined with scanning electronic microscopy (SEM, Quanta 200, FEI, Hillsboro, OR).
2.2
Development of self-healing dental luting cements
Bisphenol A glycidyl dimethacrylate (BisGMA) and TEGDMA (Esstech) were mixed at a mass ratio of 1:1 (termed BT). Then, 1% (all mass fractions) photo-initiator of phenyl-bis (2,4,6-trimethylbenzoyl) phosphine oxide (BAPO, Sigma-Aldrich) and 1% BPO were mixed into BT, which formed the resin matrix for paste A (termed BT part A). For paste B, 0.5% DHEPT was mixed into BT (termed BT part B). This formed a dual-cure system where BAPO was for light-cure and BPO was for chemical-cure. Paste A contained BPO as the initiator, and paste B contained DHEPT as the catalyst. For mechanical reinforcement, barium boroaluminosilicate glass particles with a median particle size of 1.45 μm (Caulk/Dentsply, Milford, DE) were silanized with 4% 3-methacryloxypropyltrimethoxysilane and 2% n -propylamine . For bonding capability, an acidic functional adhesive monomer 4-methacryloyloxyethyl trimellitic anhydride (4-META, Sigma-Aldrich) was added to the resin in addition to glass fillers to form the cement. Paste A contained 40% BT part A + 5% 4-META + 55% glass fillers. Paste B contained 40% BT part B + 5% 4-META + 55% glass fillers.
Furthermore, the BPO in paste A served as the initiator to react with the DHEPT in the healing liquid in the microcapsules to trigger the self-healing polymerization. The following microcapsule mass fractions were incorporated into the final cement (when an equal amount of A and B were mixed): 0% (experimental control), 2.5%, 5%, 7.5%, 8.5%, 9.5% and 10%. Clearfil™ SA cement (Kuraray, Tokyo, Japan) was used as a commercial control. Clearfil™ SA cement also had two parts, containing BisGMA, TEGDMA, and silanated colloidal silica particles as fillers. Furthermore, paste A contained acidic functional adhesive monomer of 10-Methacryloyloxydecyl dihydrogen phosphate (MDP), photo-initiator, BPO and initiator. Paste B contained accelerators, according to the manufacturer.
2.3
Dentin shear bond strength test
Extracted caries-free human third molars were stored in 0.01% thymol solution. Flat mid-coronal dentin surfaces were prepared by cutting off the tips of molar crowns with a 150 μm-thick diamond saw (Isomet, Buehler, Lake Bluff, IL). Each tooth was embedded in a poly-carbonate holder (Bosworth, Skokie, IL) and ground perpendicularly to the longitudinal axis on 320-grit silicon carbide paper until the occlusal enamel was completely removed. A double-stick tape with a central hole of a diameter of 3 mm was used to cover the moist dentin surface, and a stainless-steel iris with a central opening (diameter = 4 mm, thickness = 1.5 mm) was placed against the tape, exposing the 3 mm hole. Each mixed cement paste was placed to fill the opening in the iris. The bonded specimens were light-cured for 60 s (Triad 2000, Dentsply, York, PA). After 1 h, all the specimens were stored in water at 37 °C for 24 h. To measure the dentin shear bond strength, a chisel connected with a computer-controlled Universal Testing Machine (MTS, Eden Prairie, MN) was aligned to be parallel to the cement-dentin interface. A load was applied at a rate of 0.5 mm/min until the bond failed. Dentin shear bond strength = 4P/(πd 2 ), where P is the failure load, and d is the diameter of central hole of the tape .
2.4
Flexural test
Each cement paste was placed into a mold of 2 × 2 x 25 mm and covered with Mylar strips. Specimens were photo-cured (Triad 2000, Dentsply, York, PA) for 1 min on each open side of the mold. After 1 h, the specimens were demolded and stored in water at 37 °C for 24 h. A computer-controlled Universal Testing Machine (5500R, MTS, Cary, NC) was used to fracture the specimens in three-point flexure with a span of 10 mm and a crosshead speed of 1 mm/min . Flexural strength was measured as: S = 3P max L/(2bh 2 ), where P max is the load-at-failure, L is span, b is width and h is thickness. Elastic modulus was measured as: E = (P/d)(L 3 /[4bh 3 ]), where load P divided by displacement d is the slope in the linear elastic region of the load-displacement curve .
2.5
Fracture toughness K IC test and measurement of self-healing efficiency
K IC was measured via a single edge V-notched beam (SEVNB) method . A notch with a depth of approximately 500 μm was machined into the 2 × 2 x 25 mm bar using a diamond blade with a 150 μm thickness (Buehler, Lake Bluff, IL). Then, a 3-μm diamond paste (Buehler, city, IL) was placed into the notch tip, and a new sharp razor blade was used to cut the notch further to a total depth of about 700–800 μm . This method yielded a relatively fresh sharp notch tip . The notch length was measured using an optical microscope on both sides of the specimen and then the notch length was averaged for each specimen. The notched specimens were tested in the same three-point fixture with the notch on the tensile side, and the loading pin was aligned with the notch under a 3 × magnifier. K IC was calculated following an established SEVNB method . This yielded the original virgin K IC of the cement, K IC-virgin .
In order to test the self-healing efficiency, immediately following specimen fracture, the two halves of the specimen were placed back into the mold to ensure a good contact of the two fractured planes. This would be similar to the case of the cement in a tooth-restoration interface, where the cracked cement would stay in the same position to allow the released healing liquid to heal the crack. Since fracture ruptured the microcapsules, the released TEGDMA-DHEPT from the microcapsules would react with the BPO in the matrix. This would cause the polymerization of the released liquid to heal and bond the two cracked planes into one cohesive specimen. This healed sample was incubated in a humidor at 37 °C for 24 h. The notch length was again measured and made sure that it was the same as the virgin notch length of the same specimen. Then the healed bar was tested again using the same flexural method to measure the K IC . This yielded the healed value, K IC-healed . The self-healing efficiency (η) was assessed: η = K IC-healed /K IC-virgin , following a previous study .
The fractured surfaces of selected self-healed specimens were examined via metallographic microscope (BX51 M, Olympus, Japan). In addition, the fractured and unhealed surfaces were rinsed with ethyl alcohol immediately after fracture to remove the released healing liquid, and then the surfaces were also examined via the metallographic microscope.
2.6
Water-aging of self-healing cement specimens
The self-healing cement with 7.5% microcapsules had a flexural strength similar to that with 0% microcapsules, while having a high self-healing efficiency. The cement with 8.5%-10% microcapsules had a relatively lower flexural strength. Therefore, the cement containing 7.5% microcapsules was selected for water-aging test for 6 months. The cured specimens of 2 × 2 × 25 mm were immersed in distilled water at 37 °C for 1 day and 1, 2, 3 and 6 months. For each time period, six specimens were immersed in 200 mL of water in a sealed polyethylene container. The water was changed once every week. After each water-aging time period, the K IC-virgin was measured . Then the two broken halves of each specimen were allowed to heal as described above, and the K IC-healed was measured. This yielded the self-healing efficiency η vs. water-aging time for the cement .
2.7
Statistical analysis
One-way and two-way analyses-of-variance (ANOVA) were performed to detect the significant effects of the variables. Tukey’s multiple comparison was used to compare the data at p of 0.05.
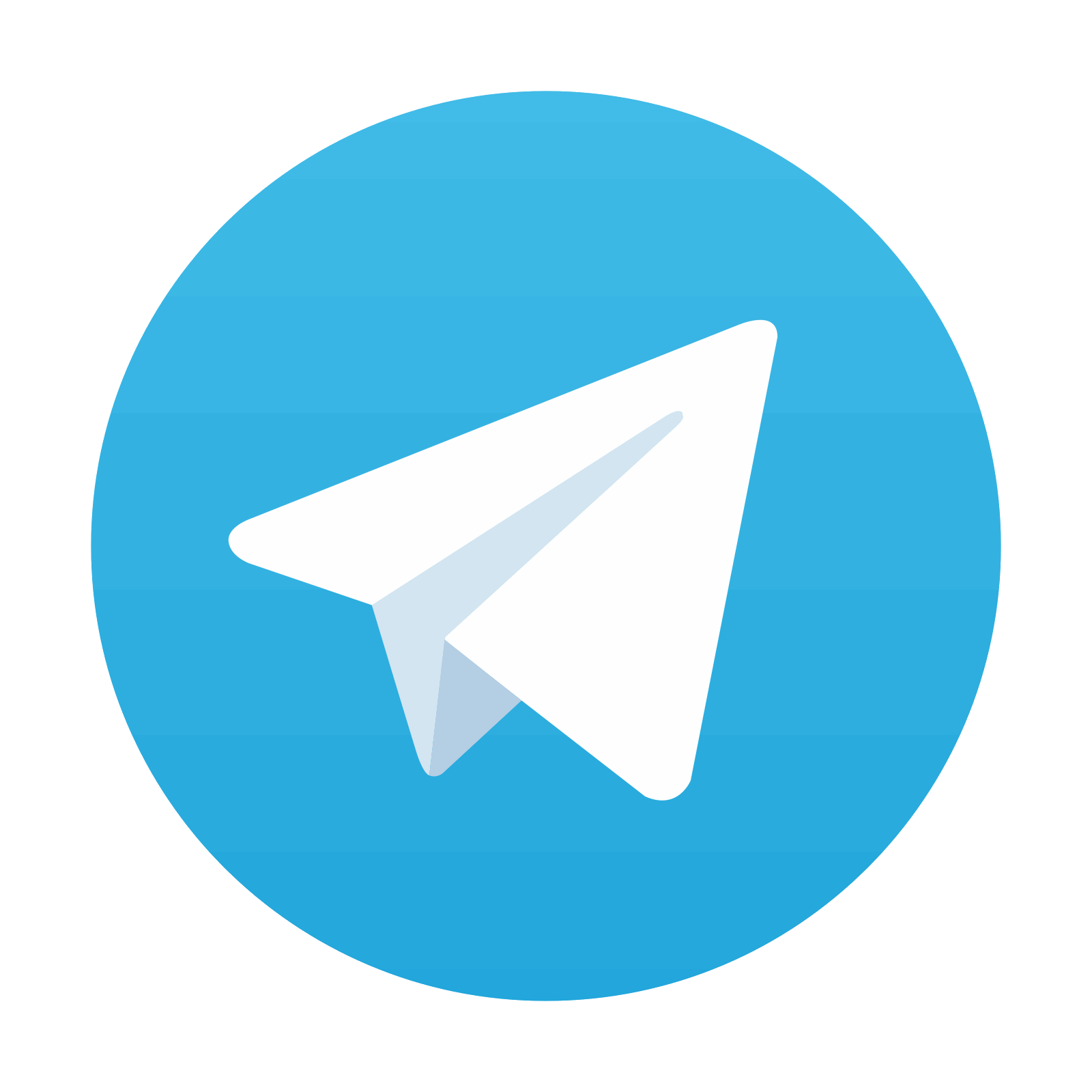
Stay updated, free dental videos. Join our Telegram channel

VIDEdental - Online dental courses
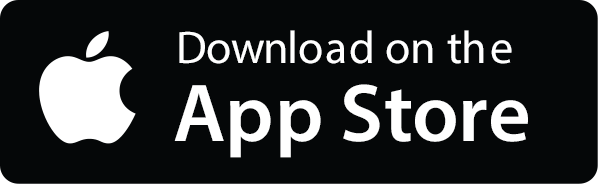
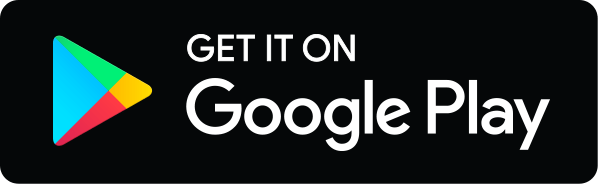