Abstract
Objective
Rechargeable calcium phosphate (CaP) composites were developed recently. However, none of the rechargeable CaP composites was antibacterial. The objectives of this study were to develop the first rechargeable CaP composite that was antibacterial, and to investigate the effects of adding dimethylaminohexadecyl methacrylate (DMAHDM) into rechargeable CaP composite on ion rechargeability and re-release as well as biofilm properties.
Methods
DMAHDM was synthesized via a Menschutkin reaction. Nanoparticles of amorphous calcium phosphate (NACP) were synthesized using a spray-drying technique. The resin contained ethoxylated bisphenol A dimethacrylate (EBPADMA) and pyromellitic glycerol dimethacrylate (PMGDM). Two composites were fabricated: rechargeable NACP composite, and rechargeable NACP-DMAHDM composite. Mechanical properties and ion release and recharge were measured. A dental plaque microcosm biofilm model using saliva was tested.
Results
Flexural strength and elastic modulus of rechargeable NACP and NACP-DMAHDM composites matched commercial control composite (p > 0.1). NACP-DMAHDM inhibited biofilm metabolic activity and lactic acid, and reduced biofilm colony-forming units (CFU) by 3–4 log. NACP and NACP-DMAHDM showed similar Ca and P ion recharge and re-release (p > 0.1). Therefore, adding DMAHDM did not compromise the ion rechargeability. One recharge yielded continuous release for 42 d. The release was maintained at the same level with increasing number of recharge cycles, indicating long-term ion release and remineralization capability.
Conclusions
The first CaP rechargeable and antibacterial composite was developed. Adding DMAHDM into the rechargeable NACP composite did not adversely affect the Ca and P ion release and recharge, and the composite had much less biofilm growth and lactic acid production, with CFU reduction by 3–4 log.
Clinical significance
This novel CaP rechargeable composite with long-term remineralization and antibacterial properties is promising for tooth restorations to inhibit caries.
1
Introduction
Dental diseases are among the most prevalent chronic diseases in humans. Dental diseases create substantial public healthcare and economic burdens and affect the oral and general health and the quality of life [ ]. Dental composites are popular materials to restore carious teeth due to their esthetics and direct-filling capabilities. Significant improvements in composites have resulted in the surge of their use [ ]. However, composite restorations have limited lifetime due to several drawbacks, including polymerization shrinkage/stress formation, fracture, relatively low abrasion and wear resistance, and higher marginal leakage [ ]. Marginal leakage can provide pathways for bacteria to invade; these bacteria can produce acids that lead to demineralization and secondary caries. Indeed, secondary caries is a primary reason for restoration failures [ ], and the treatment of the failed restorations accounts for 50–70% of all operative procedures performed [ ]. Furthermore, the treatment of secondary caries usually results in additional loss of tooth structures and may weaken the affected tooth [ ]. Consequently, there is a substantial need to develop a new generation of composites with the ability to combat secondary caries.
Therefore, efforts were made to formulate dental resins that possessed antibacterial and remineralization abilities [ ]. These methods involved the incorporation of antimicrobial agents into resin composites and bonding agents [ ]. Quaternary ammonium methacrylates (QAMs) were synthesized and copolymerized in resins for antibacterial functions [ ]. Resins containing 12-methacryloyloxydodecylpyridinium bromide (MDPB) was shown to have a potent antibacterial activity [ ]. The antibacterial effectiveness of quaternary ammonium compounds was found to be greater with the increase of the alkyl chain length (CL) of the ammonium group [ ]. Recently, resins containing dimethylaminohexadecyl methacrylate (DMAHDM) with a chain length of 16 was shown to be strongly antibacterial [ ]. The antibacterial effects of composite and adhesive containing DMAHDM were investigated, demonstrating strong antibacterial activities against cariogenic, endodontic and periodontal pathogens [ ].
Another method for caries-inhibition involved remineralization using resins that release calcium (Ca) and phosphate (P) ions. The incorporation of nanoparticles of amorphous calcium phosphate (NACP) into composite enabled the release of Ca and P ions especially during an acidic cariogenic challenge [ ]. The ion release helps to prevent demineralization and facilitates remineralization [ ]. Furthermore, the NACP composite had the ability to be recharged with Ca and P ions to have long-term ion release [ ]. One study demonstrated the repeated recharge of Ca and P ions for a composite; however, the rechargeable NACP composite was not antibacterial [ ]. Another study incorporated protein-repellant and antibacterial agents into a rechargeable composite; however, there was no report on the effect of antibacterial agent on the rechargeability, and there was no evidence if the antibacterial composite had Ca and P ion recharge and re-release capability [ ]. To date, there has been no report on the effect of incorporation of DMAHDM into the rechargeable NACP composite on ion recharge and re-release properties.
Accordingly, the objectives of this study were to: (1) develop the first Ca and P ion rechargeable and antibacterial composite; and (2) investigate the effects of NACP and DMAHDM on mechanical properties, biofilm response, and ion release, recharge and re-release. It was hypothesized that: (1) Adding DMAHDM into rechargeable NACP composite would have good mechanical properties matching those without DMAHDM; (2) Adding DMAHDM into rechargeable NACP composite would not compromise the Ca and P ion release and recharge; and (3) The rechargeable NACP-DMAHDM composite would have much less biofilm growth and lactic acid production than the rechargeable composite without DMAHDM.
2
Material and methods
2.1
Formulation of composites
The resin consisted of ethoxylated bisphenol A dimethacrylate (EBPADMA) (Esstech, Essington, PA, USA) and pyromellitic glycerol dimethacrylate (PMGDM) (Esstech, Essington, PA, USA) with each at 49.5% (by mass), and 0.2% camphorquinone and 0.8% ethyl 4-N,N-diethylaminobenzoate for light-cure activation [ ]. This resin is referred to as EBPM.
A modified Menschutkin reaction was used to synthesize DMAHDM in which a tertiary amine group was reacted with an organ-halide [ ]. Briefly, 10 mmol of 2-(dimethylamino)ethyl methacrylate (DMAEMA, Sigma-Aldrich, St. Louis, MO, USA) and 10 mmol of 1-bromohexadecane (BHD, TCI America, Portland, OR, USA) were combined with 3 g of ethanol in a 20 mL scintillation vial. The vial was stirred at 70 °C to react for 24 h. The solvent was then removed via evaporation, yielding DMAHDM as a clear, colorless, and viscous liquid [ ]. DMAHDM was mixed with EBPM at a mass fraction of DMAHDM/(EBPM + DMAHDM) = 10%, according to a previous study [ ]. This resin is referred to as EBPM-DMAHDM.
A spray-drying technique was used to synthesize NACP [ ]. Briefly, calcium carbonate and dicalcium phosphate anhydrous were dissolved in acetic acid to produce Ca and P with concentrations of 8 mmol/L and 5.333 mmol/L, respectively, thus yielding a Ca/P molar ratio of 1.5, the same as that for ACP [Ca 3 (PO 4 ) 2 ]. This solution was sprayed into a heated chamber of the spray-drying machine. An electrostatic precipitator was used to collect the dry NACP. This produced NACP with a mean particle size of 116 nm [ ]. In addition, silanized barium boroaluminosilicate glass particles with a median size of 1.4 μm (Caulk/Dentsply, Milford, DE, USA) were used as a co-filler for mechanical reinforcement. These fillers were incorporated into the resin at a total filler mass fraction of 70%, including 20% NACP and 50% glass, which were readily mixed to produce a cohesive composite paste. Two resins were used: EBPM, and EBPM-DMAHDM. For the EBPM-DMAHDM composite, the filler level was 70%, the resin matrix was 30% and the resin contained 10% DMAHDM; hence, the final DMAHDM mass fraction in the composite was 3%.
In addition, a commercial composite (Heliomolar, Ivoclar, Ontario, Canada) was used as comparative control. It contained 66.7% of nanofillers of 40–200 nm of silica and ytterbium-trifluoride with fluoride-release. According to the manufacturer, Heliomolar is indicated for Class I, II, III, IV and V restorations. Therefore, three composites were tested:
- (1.)
Heliomolar (referred to as commercial control composite);
- (2.)
Rechargeable NACP composite: 20% NACP + 50% glass + 30% EBPM;
- (3.)
Rechargeable antibacterial NACP-DMAHDM composite: 20% NACP + 50% glass + 3% DMAHDM + 27% EBPM.
2.2
Mechanical properties
Each composite paste was placed into a stainless steel mold of 2 × 2 × 25 mm, covered with Mylar strips and light-cured (Triad 2000, Dentsply, York, PA, USA) for 1 min on each open side. The irradiance of the Triad 2000 machine was previously calculated to be 65 mW/cm 2 at the specimen position [ ]. Specimens were stored dry at 37 °C for 24 h. Flexural strength and elastic modulus were measured using a three-point flexural test with a 10 mm span at a crosshead-speed of 1 mm/min on a computer-controlled Universal Testing Machine (5500R, MTS, Cary, NC, USA), by following the ISO guidelines [ ]. Flexural strength was calculated by: S = 3Pmax/L(2bh 2 ), where Pmax is the fracture load, L is the span, b is specimen width and h is thickness. Elastic modulus was calculated by: E = (P/d)(L 3 /[4bh 3 ]), where load P divided by displacement d is the slope in the linear elastic region [ ].
2.3
Saliva collection and dental plaque microcosm biofilm model
Circular Teflon molds (9 mm in diameter and 2 mm in thickness) were used to fabricate composite disks for biofilm tests. Specimens were covered with Mylar strips and photo-cured in the same manner as described above. The cured composite disk was immersed in 200 mL of distilled water and magnetically stirred at 100 rpm for 1 h to remove any uncured monomers, following a previous study [ ]. Sterilization of the composite disks was performed with ethylene oxide (AnproleneAN 74i, Andersen, Haw River, NC, USA). Then the specimens were de-gassed for 7 days following the manufacturer’s instructions, prior to bacterial experiments.
The dental microcosm biofilm model was approved by the University of Maryland Baltimore Institutional Review Board. This model used human saliva as inoculum and has the advantage of providing the complexity and heterogeneity of the microorganisms that are available in the dental plaque complex [ ]. An equal volume of saliva was collected from ten healthy donors having natural dentition without active caries, and not having used antibiotics within the past 3 months. The donors were instructed to avoid tooth brushing for 24 h and abstained from food and drink intake for 2 h prior to saliva donation. Then the saliva from the ten donors was combined and diluted in sterile glycerol to a concentration of 70%, and stored at −80 °C for subsequent use [ ].
The saliva-glycerol solution was added, with 1:50 final dilution, into a McBain artificial saliva growth medium as inoculum. This medium contained mucin (Type II, porcine, gastric) at a concentration of 2.5 g/L; bacteriological peptone, 2.0 g/L; tryptone, 2.0 g/L; yeast extract, 1.0 g/L; NaCl, 0.35 g/L, KCl, 0.2 g/L; CaCl 2 , 0.2 g/L; cysteine hydrochloride, 0.1 g/L; hemin, 0.001 g/L; vitamin K 1 , 0.0002 g/L, at pH 7 [ ]. 2% sucrose was added to this medium. Then, 1.5 mL of the inoculum was added to each well of 24-well plates containing a composite disk on the well bottom. The samples were incubated at 37 °C in 5% CO 2 for 8 h to grow the biofilms on the composite disk surface. Then, the disks were transferred to new 24-well plates with fresh medium and incubated for 16 h. Then, the disks were transferred to new 24-well plates with fresh medium and incubated for another 24 h. This totaled 48 h of culture, which was previously shown to form relatively mature dental plaque microcosm biofilms on dental resins [ ].
2.4
Live/dead staining of biofilms
Composite disks with 2-day biofilms were washed with phosphate buffered saline (PBS) and stained using the BacLight live/dead kit (Molecular Probes, Eugene, OR, USA) [ ]. A mixture of 2.5 μM SYTO 9 and 2.5 μM propidium iodide was used to stain each sample for 15 min. Live bacteria were stained with SYTO9 to produce a green fluorescence. Bacteria with compromised membranes were stained with propidium iodide to produce a red fluorescence. The stained disks were examined using an inverted epifluorescence microscope (Eclipse TE2000-S, Nikon, Melville, NY, USA). Six disks were evaluated for each group. Three randomly chosen fields of view were photographed from each disk, yielding 18 images for each composite.
2.5
MTT assay of metabolic activity of biofilms
Separate composite disks with 2-day biofilms were prepared. A colorimetric assay was used that measures the enzymatic reduction of MTT (3-[4,5-dimethylthiazol-2-yl]-2,5-diphenyltetrazolium bromide), a yellow tetrazole, to formazan [ ]. Disks with 2-day biofilms were transferred to a new 24-well plate, and 1 mL of tetrazolium dye was added to each well and incubated at 37 °C in 5% CO 2 for 1 h. Then the disks were transferred to a new 24-well plate, and 1 mL of dimethyl sulfoxide (DMSO) was added to dissolve the formazan crystals. The samples were incubated for 20 min with gentle stirring in the dark. Then 200 mL of the DMSO solution from each well was collected, and its absorbance was measured via a microplate reader at 540 nm (SpectraMax M5, Molecular Devices, Sunnyvale, CA, USA). A higher absorbance is related to a higher formazan concentration, which indicates a higher metabolic activity in the biofilm on the disk [ ].
2.6
Lactic acid production by biofilms
Disks with 2-day biofilms were transferred to 24-well plates containing buffered-peptone water (BPW) plus 0.2% sucrose. The 24-well plate was incubated for 3 h to allow the biofilms to produce acid. The lactate concentrations in the BPW solutions were determined using an enzymatic (lactate dehydrogenase) method, as described in previous studies [ ]. BPW solutions were collected from each well and the absorbance was measured at 340 nm (optical density OD 340 ) using the microplate reader (SpectraMax M5, Molecular Devices, Sunnyvale, CA, USA). Standard curves were prepared using a lactic acid standard (Supelco, Bellefonte, PA, USA) as described previously [ ].
2.7
Colony-forming unit (CFU) counts of biofilms on composites
with 2-day biofilms were transferred into tubes with 2 mL of CPW, and the biofilms were harvested by sonication and vortex (Analog Vortex Mixer, Fisher Scientific, Waltham, MA, USA) [ ]. Three different types of agar plates were evaluated. First, tryptic soy blood agar culture plates were used to determine the total microorganisms [ ]. Second, mitis salivarius agar (MSA) culture plates containing 15% sucrose were used to determine total streptococci [ ]. This MSA agar contains agents such as crystal violet, potassium tellurite and trypan blue, which inhibit most Gram-negative bacilli and most Gram-positive bacteria except streptococci, thus enabling the growth of streptococci [ ]. Third, MSA agar culture plates with 0.2 units of bacitracin per mL were used to determine the mutans streptococci [ ]. The bacterial suspensions were serially diluted and transferred into the three agar plates to evaluate the microorganism colonies. The agar plates were incubated at 37 °C in 5% CO 2 for 48 h. The number of colonies was counted and used, along with the dilution factor, to calculate the CFU on each specimen [ ].
2.8
Measurement of Ca and P ion release from NACP
A sodium chloride (NaCl) solution (133 mmol/L) was buffered to pH 4 with 50 mmol/L lactic acid to measure ion release, simulating a cariogenic condition [ ]. Three specimens of approximately 2 × 2 × 12 mm were immersed in 50 mL of solution to yield a specimen volume/solution of 2.9 mm 3 /mL. This was similar to a specimen volume per solution of about 3.0 mm 3 /mL in previous studies. The concentrations of Ca and P ions released from the specimens were measured at 1, 3, 5, 7, 14, 21, 28, 35, 42, 49, 56, 63 and 70 days [ ]. At each time, aliquots of 0.5 mL were removed and replaced by fresh solution [ ]. The aliquots were analyzed for Ca and P ion concentrations via a spectrophotometric method (DMS-80 UV–vis, Varian, Palo Alto, CA, USA) using known standards and calibration curves [ ]. In addition, the pH of each solution was checked and adjusted to pH 4 using 50 mM lactic acid for standardization. Only groups 2 and 3 were tested for Ca and P ion release; group 1 was not included because the commercial composite did not contain Ca and P ions. Six specimens were tested for each group (n = 6) [ ]. The measured ion releases are referred to as “initial release” to differentiate from the recharge and re-release below.
2.9
Ca and P ion recharge and re-release
After completion of the ion release for 70 days, the ion measurement indicated that the ion concentration had plateaued and there was no additional ion release. Then, these specimens were removed, rinsed with water for 5 min, and then immersed in a fresh 50 mL NaCl solution at pH 4. Their ion release was further measured for 7 days to ensure the ion release from these specimens was exhausted [ ]. These exhausted specimens were then used for the recharge test. The Ca ion recharge solution consisted of 20 mmol/L CaCl 2 and 50 mmol/L HEPES buffer. The P ion recharge solution consisted of 12 mmol/L KHPO 4 and 50 mmol/L HEPES buffer. The solutions were adjusted to pH 7 by the use of 1 mol/L KOH [ ]. Three specimens of approximately 2 × 2 × 12 mm were immersed in 5 mL of the calcium or phosphate solution and gently stirred for 1 min on a mixing machine (Analog Vortex Mixer, Fisher Scientific, Waltham, MA, USA) at a power level of 3 to simulate the action of using a mouthwash. Then the specimens were rinsed with distilled water for 1 min to remove any ion deposits on specimen surfaces. Following a previous study [ ], the recharge was repeated three times at 9:00 am, 1:00 pm and 5:00 pm for one day, for a total of 3 min of recharge. The next day, the measurement was started for ion re-release. The recharged specimens were transferred into a 50 mL NaCl solution at pH 4 to measure the Ca and P ion re-releases at 1, 2, 3, 5, 9, 11 and 14 days [ ]; this constituted one cycle of recharge and re-release. Then the specimens were recharged again for 3 min and the ion re-release was measured for 14 days, as cycle 2. Three cycles were tested in this study. Furthermore, after the third cycle, the specimens without further recharge were placed in 50 mL of fresh NaCl solution at pH 4 and the measurement of Ca and P ion re-release was continued for an additional 28 days, total of 42 days. The purpose of this was to determine how long the specimens could further release Ca and P ions without any additional recharge treatment [ ].
2.10
Statistical analysis
One-way and two-way analyses of variance (ANOVA) and Tukey’s multiple comparison tests were performed to detect the significant effects of the variables in the mechanical properties and antibacterial effects, and t -test was performed for the Ca and P initial ion release and recharge and re-release. All the statistical analyses were performed by SPSS 22.0 software (SPSS, Chicago, IL, USA) at an alpha of 0.05.
2
Material and methods
2.1
Formulation of composites
The resin consisted of ethoxylated bisphenol A dimethacrylate (EBPADMA) (Esstech, Essington, PA, USA) and pyromellitic glycerol dimethacrylate (PMGDM) (Esstech, Essington, PA, USA) with each at 49.5% (by mass), and 0.2% camphorquinone and 0.8% ethyl 4-N,N-diethylaminobenzoate for light-cure activation [ ]. This resin is referred to as EBPM.
A modified Menschutkin reaction was used to synthesize DMAHDM in which a tertiary amine group was reacted with an organ-halide [ ]. Briefly, 10 mmol of 2-(dimethylamino)ethyl methacrylate (DMAEMA, Sigma-Aldrich, St. Louis, MO, USA) and 10 mmol of 1-bromohexadecane (BHD, TCI America, Portland, OR, USA) were combined with 3 g of ethanol in a 20 mL scintillation vial. The vial was stirred at 70 °C to react for 24 h. The solvent was then removed via evaporation, yielding DMAHDM as a clear, colorless, and viscous liquid [ ]. DMAHDM was mixed with EBPM at a mass fraction of DMAHDM/(EBPM + DMAHDM) = 10%, according to a previous study [ ]. This resin is referred to as EBPM-DMAHDM.
A spray-drying technique was used to synthesize NACP [ ]. Briefly, calcium carbonate and dicalcium phosphate anhydrous were dissolved in acetic acid to produce Ca and P with concentrations of 8 mmol/L and 5.333 mmol/L, respectively, thus yielding a Ca/P molar ratio of 1.5, the same as that for ACP [Ca 3 (PO 4 ) 2 ]. This solution was sprayed into a heated chamber of the spray-drying machine. An electrostatic precipitator was used to collect the dry NACP. This produced NACP with a mean particle size of 116 nm [ ]. In addition, silanized barium boroaluminosilicate glass particles with a median size of 1.4 μm (Caulk/Dentsply, Milford, DE, USA) were used as a co-filler for mechanical reinforcement. These fillers were incorporated into the resin at a total filler mass fraction of 70%, including 20% NACP and 50% glass, which were readily mixed to produce a cohesive composite paste. Two resins were used: EBPM, and EBPM-DMAHDM. For the EBPM-DMAHDM composite, the filler level was 70%, the resin matrix was 30% and the resin contained 10% DMAHDM; hence, the final DMAHDM mass fraction in the composite was 3%.
In addition, a commercial composite (Heliomolar, Ivoclar, Ontario, Canada) was used as comparative control. It contained 66.7% of nanofillers of 40–200 nm of silica and ytterbium-trifluoride with fluoride-release. According to the manufacturer, Heliomolar is indicated for Class I, II, III, IV and V restorations. Therefore, three composites were tested:
- (1.)
Heliomolar (referred to as commercial control composite);
- (2.)
Rechargeable NACP composite: 20% NACP + 50% glass + 30% EBPM;
- (3.)
Rechargeable antibacterial NACP-DMAHDM composite: 20% NACP + 50% glass + 3% DMAHDM + 27% EBPM.
2.2
Mechanical properties
Each composite paste was placed into a stainless steel mold of 2 × 2 × 25 mm, covered with Mylar strips and light-cured (Triad 2000, Dentsply, York, PA, USA) for 1 min on each open side. The irradiance of the Triad 2000 machine was previously calculated to be 65 mW/cm 2 at the specimen position [ ]. Specimens were stored dry at 37 °C for 24 h. Flexural strength and elastic modulus were measured using a three-point flexural test with a 10 mm span at a crosshead-speed of 1 mm/min on a computer-controlled Universal Testing Machine (5500R, MTS, Cary, NC, USA), by following the ISO guidelines [ ]. Flexural strength was calculated by: S = 3Pmax/L(2bh 2 ), where Pmax is the fracture load, L is the span, b is specimen width and h is thickness. Elastic modulus was calculated by: E = (P/d)(L 3 /[4bh 3 ]), where load P divided by displacement d is the slope in the linear elastic region [ ].
2.3
Saliva collection and dental plaque microcosm biofilm model
Circular Teflon molds (9 mm in diameter and 2 mm in thickness) were used to fabricate composite disks for biofilm tests. Specimens were covered with Mylar strips and photo-cured in the same manner as described above. The cured composite disk was immersed in 200 mL of distilled water and magnetically stirred at 100 rpm for 1 h to remove any uncured monomers, following a previous study [ ]. Sterilization of the composite disks was performed with ethylene oxide (AnproleneAN 74i, Andersen, Haw River, NC, USA). Then the specimens were de-gassed for 7 days following the manufacturer’s instructions, prior to bacterial experiments.
The dental microcosm biofilm model was approved by the University of Maryland Baltimore Institutional Review Board. This model used human saliva as inoculum and has the advantage of providing the complexity and heterogeneity of the microorganisms that are available in the dental plaque complex [ ]. An equal volume of saliva was collected from ten healthy donors having natural dentition without active caries, and not having used antibiotics within the past 3 months. The donors were instructed to avoid tooth brushing for 24 h and abstained from food and drink intake for 2 h prior to saliva donation. Then the saliva from the ten donors was combined and diluted in sterile glycerol to a concentration of 70%, and stored at −80 °C for subsequent use [ ].
The saliva-glycerol solution was added, with 1:50 final dilution, into a McBain artificial saliva growth medium as inoculum. This medium contained mucin (Type II, porcine, gastric) at a concentration of 2.5 g/L; bacteriological peptone, 2.0 g/L; tryptone, 2.0 g/L; yeast extract, 1.0 g/L; NaCl, 0.35 g/L, KCl, 0.2 g/L; CaCl 2 , 0.2 g/L; cysteine hydrochloride, 0.1 g/L; hemin, 0.001 g/L; vitamin K 1 , 0.0002 g/L, at pH 7 [ ]. 2% sucrose was added to this medium. Then, 1.5 mL of the inoculum was added to each well of 24-well plates containing a composite disk on the well bottom. The samples were incubated at 37 °C in 5% CO 2 for 8 h to grow the biofilms on the composite disk surface. Then, the disks were transferred to new 24-well plates with fresh medium and incubated for 16 h. Then, the disks were transferred to new 24-well plates with fresh medium and incubated for another 24 h. This totaled 48 h of culture, which was previously shown to form relatively mature dental plaque microcosm biofilms on dental resins [ ].
2.4
Live/dead staining of biofilms
Composite disks with 2-day biofilms were washed with phosphate buffered saline (PBS) and stained using the BacLight live/dead kit (Molecular Probes, Eugene, OR, USA) [ ]. A mixture of 2.5 μM SYTO 9 and 2.5 μM propidium iodide was used to stain each sample for 15 min. Live bacteria were stained with SYTO9 to produce a green fluorescence. Bacteria with compromised membranes were stained with propidium iodide to produce a red fluorescence. The stained disks were examined using an inverted epifluorescence microscope (Eclipse TE2000-S, Nikon, Melville, NY, USA). Six disks were evaluated for each group. Three randomly chosen fields of view were photographed from each disk, yielding 18 images for each composite.
2.5
MTT assay of metabolic activity of biofilms
Separate composite disks with 2-day biofilms were prepared. A colorimetric assay was used that measures the enzymatic reduction of MTT (3-[4,5-dimethylthiazol-2-yl]-2,5-diphenyltetrazolium bromide), a yellow tetrazole, to formazan [ ]. Disks with 2-day biofilms were transferred to a new 24-well plate, and 1 mL of tetrazolium dye was added to each well and incubated at 37 °C in 5% CO 2 for 1 h. Then the disks were transferred to a new 24-well plate, and 1 mL of dimethyl sulfoxide (DMSO) was added to dissolve the formazan crystals. The samples were incubated for 20 min with gentle stirring in the dark. Then 200 mL of the DMSO solution from each well was collected, and its absorbance was measured via a microplate reader at 540 nm (SpectraMax M5, Molecular Devices, Sunnyvale, CA, USA). A higher absorbance is related to a higher formazan concentration, which indicates a higher metabolic activity in the biofilm on the disk [ ].
2.6
Lactic acid production by biofilms
Disks with 2-day biofilms were transferred to 24-well plates containing buffered-peptone water (BPW) plus 0.2% sucrose. The 24-well plate was incubated for 3 h to allow the biofilms to produce acid. The lactate concentrations in the BPW solutions were determined using an enzymatic (lactate dehydrogenase) method, as described in previous studies [ ]. BPW solutions were collected from each well and the absorbance was measured at 340 nm (optical density OD 340 ) using the microplate reader (SpectraMax M5, Molecular Devices, Sunnyvale, CA, USA). Standard curves were prepared using a lactic acid standard (Supelco, Bellefonte, PA, USA) as described previously [ ].
2.7
Colony-forming unit (CFU) counts of biofilms on composites
with 2-day biofilms were transferred into tubes with 2 mL of CPW, and the biofilms were harvested by sonication and vortex (Analog Vortex Mixer, Fisher Scientific, Waltham, MA, USA) [ ]. Three different types of agar plates were evaluated. First, tryptic soy blood agar culture plates were used to determine the total microorganisms [ ]. Second, mitis salivarius agar (MSA) culture plates containing 15% sucrose were used to determine total streptococci [ ]. This MSA agar contains agents such as crystal violet, potassium tellurite and trypan blue, which inhibit most Gram-negative bacilli and most Gram-positive bacteria except streptococci, thus enabling the growth of streptococci [ ]. Third, MSA agar culture plates with 0.2 units of bacitracin per mL were used to determine the mutans streptococci [ ]. The bacterial suspensions were serially diluted and transferred into the three agar plates to evaluate the microorganism colonies. The agar plates were incubated at 37 °C in 5% CO 2 for 48 h. The number of colonies was counted and used, along with the dilution factor, to calculate the CFU on each specimen [ ].
2.8
Measurement of Ca and P ion release from NACP
A sodium chloride (NaCl) solution (133 mmol/L) was buffered to pH 4 with 50 mmol/L lactic acid to measure ion release, simulating a cariogenic condition [ ]. Three specimens of approximately 2 × 2 × 12 mm were immersed in 50 mL of solution to yield a specimen volume/solution of 2.9 mm 3 /mL. This was similar to a specimen volume per solution of about 3.0 mm 3 /mL in previous studies. The concentrations of Ca and P ions released from the specimens were measured at 1, 3, 5, 7, 14, 21, 28, 35, 42, 49, 56, 63 and 70 days [ ]. At each time, aliquots of 0.5 mL were removed and replaced by fresh solution [ ]. The aliquots were analyzed for Ca and P ion concentrations via a spectrophotometric method (DMS-80 UV–vis, Varian, Palo Alto, CA, USA) using known standards and calibration curves [ ]. In addition, the pH of each solution was checked and adjusted to pH 4 using 50 mM lactic acid for standardization. Only groups 2 and 3 were tested for Ca and P ion release; group 1 was not included because the commercial composite did not contain Ca and P ions. Six specimens were tested for each group (n = 6) [ ]. The measured ion releases are referred to as “initial release” to differentiate from the recharge and re-release below.
2.9
Ca and P ion recharge and re-release
After completion of the ion release for 70 days, the ion measurement indicated that the ion concentration had plateaued and there was no additional ion release. Then, these specimens were removed, rinsed with water for 5 min, and then immersed in a fresh 50 mL NaCl solution at pH 4. Their ion release was further measured for 7 days to ensure the ion release from these specimens was exhausted [ ]. These exhausted specimens were then used for the recharge test. The Ca ion recharge solution consisted of 20 mmol/L CaCl 2 and 50 mmol/L HEPES buffer. The P ion recharge solution consisted of 12 mmol/L KHPO 4 and 50 mmol/L HEPES buffer. The solutions were adjusted to pH 7 by the use of 1 mol/L KOH [ ]. Three specimens of approximately 2 × 2 × 12 mm were immersed in 5 mL of the calcium or phosphate solution and gently stirred for 1 min on a mixing machine (Analog Vortex Mixer, Fisher Scientific, Waltham, MA, USA) at a power level of 3 to simulate the action of using a mouthwash. Then the specimens were rinsed with distilled water for 1 min to remove any ion deposits on specimen surfaces. Following a previous study [ ], the recharge was repeated three times at 9:00 am, 1:00 pm and 5:00 pm for one day, for a total of 3 min of recharge. The next day, the measurement was started for ion re-release. The recharged specimens were transferred into a 50 mL NaCl solution at pH 4 to measure the Ca and P ion re-releases at 1, 2, 3, 5, 9, 11 and 14 days [ ]; this constituted one cycle of recharge and re-release. Then the specimens were recharged again for 3 min and the ion re-release was measured for 14 days, as cycle 2. Three cycles were tested in this study. Furthermore, after the third cycle, the specimens without further recharge were placed in 50 mL of fresh NaCl solution at pH 4 and the measurement of Ca and P ion re-release was continued for an additional 28 days, total of 42 days. The purpose of this was to determine how long the specimens could further release Ca and P ions without any additional recharge treatment [ ].
2.10
Statistical analysis
One-way and two-way analyses of variance (ANOVA) and Tukey’s multiple comparison tests were performed to detect the significant effects of the variables in the mechanical properties and antibacterial effects, and t -test was performed for the Ca and P initial ion release and recharge and re-release. All the statistical analyses were performed by SPSS 22.0 software (SPSS, Chicago, IL, USA) at an alpha of 0.05.
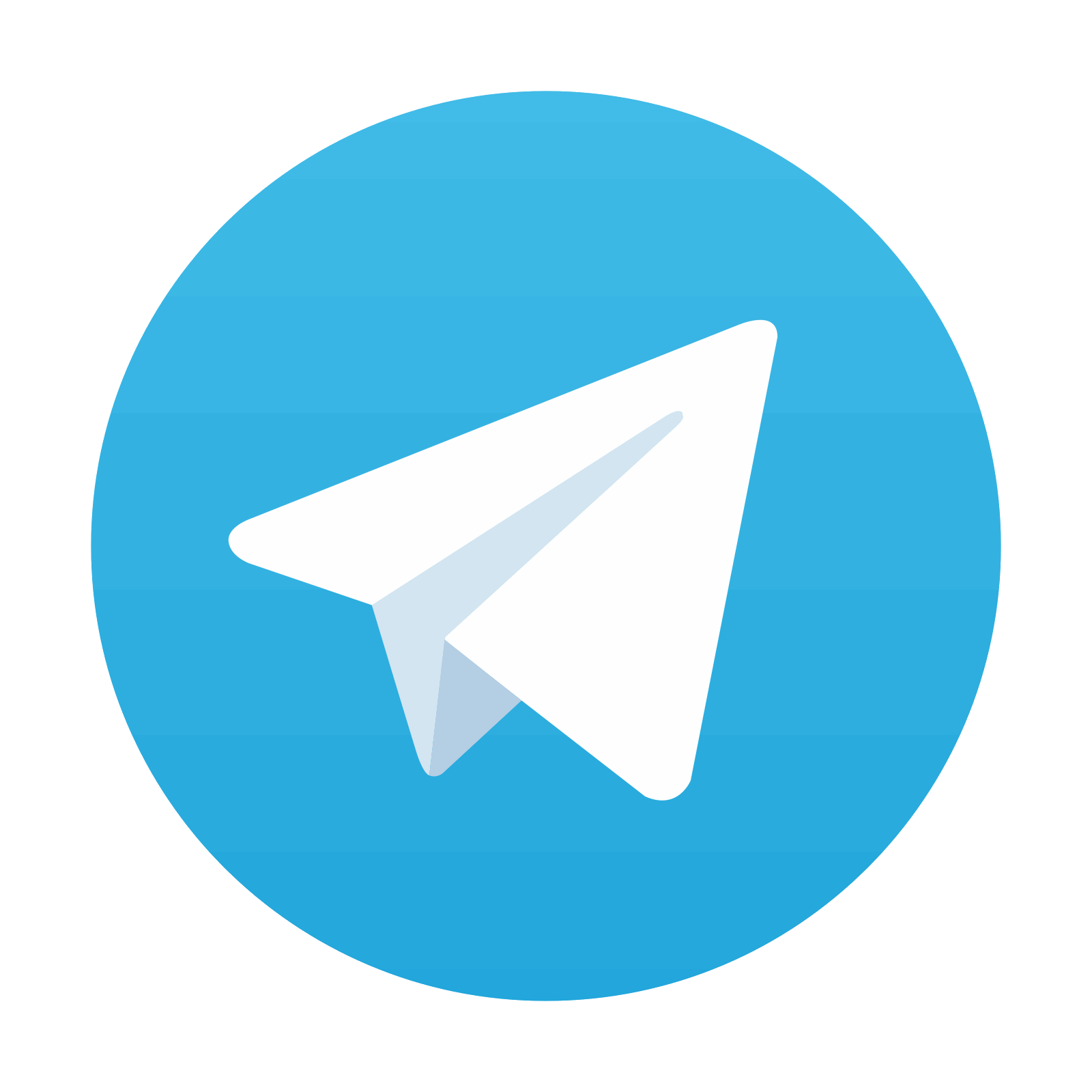
Stay updated, free dental videos. Join our Telegram channel

VIDEdental - Online dental courses
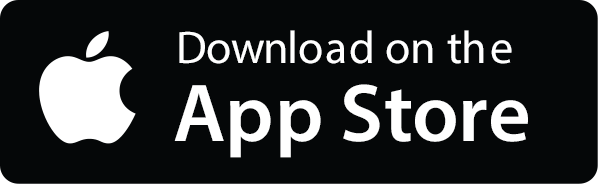
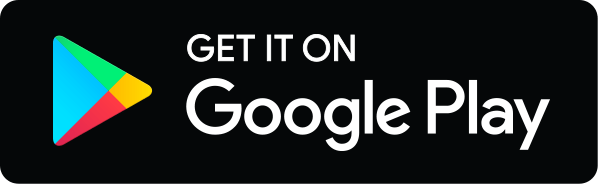