Abstract
Objectives
White spot lesions due to biofilm acid-induced enamel demineralization are prevalent in orthodontic treatments. The aim of this study was to develop a novel bioactive multifunctional cement with protein-repellent, antibacterial and remineralizing capabilities, and investigate the effects on enamel hardness and lesion depth in vitro for the first time.
Materials and methods
2-Methacryloyloxyethyl phosphorylcholine (MPC), dimethylaminohexadecyl methacrylate (DMAHDM), and nanoparticles of amorphous calcium phosphate (NACP) were incorporated into a resin-modified glass ionomer (RMGI). Extracted human premolars had brackets bonded via four groups: (1) Transbond XT (TB), (2) RMGI (GC Ortho LC), (3) RMGI + MPC + DMAHDM, (4) RMGI + MPC + DMAHDM + NACP. Demineralization was induced via a dental plaque microcosm biofilm model. Samples were tested using polarized light microscopy (PLM) for lesion depth. Enamel hardness was tested for different groups.
Results
Incorporating MPC, DMAHDM and NACP did not affect enamel bond strength. “RMGI + MPC + DMAHDM + NACP” group had the least lesion depth in enamel ( p < 0.05). Groups with NACP had the highest enamel hardness ( p < 0.05). Mineral loss (ΔS) in enamel for NACP group was about one third that for RMGI control. “RMGI + MPC + DMAHDM” had greater effect on demineralization-inhibition, compared to RMGI and TB controls. “RMGI + MPC + DMAHDM + NACP” was more effective in protecting enamel prisms from dissolution by biofilm acids, compared to RMGI and TB control groups.
Conclusion
The Novel “RMGI + MPC + DMAHDM + NACP” cement substantially reduced enamel demineralization adjacent to orthodontic brackets, yielding much less lesion depth and greater enamel hardness under biofilm acid attacks than commercial controls. The clinical significance is that the novel multi-agent (RMGI + MPC + DMAHDM + NACP) method is promising for a wide range of preventive and restorative applications to combat caries.
1
Introduction
Orthodontic treatments are increasingly more popular especially among the young people; however, the fixed appliances often cause white spot lesions (WSLs) on enamel surfaces due to demineralization . Clinically, chalky spots manifestation of enamel demineralization around appliances can be detected as early as on the first return visit, representing the formation of initial smooth surface caries caused by biofilm acids. A high incidence of WSLs has been observed in younger patients with poor oral hygiene during treatment. WSLs and enamel caries can cause dissatisfaction compromising the hard-earned therapeutic outcomes among patients, their parents, and attending orthodontists . Biofilms are more inclined to aggregate on the surfaces of irregular structures such as brackets, bands and bonding agents. Cariogenic genus inside the biofilms, especially Streptococcus mutans , devote themselves to producing acid, thereby lowering the local pH and resulting the release of calcium and phosphate ions out of enamel .
Various approaches of fluoride (F − ) preventive applications, including mouth-rinses, fluoridated dentifrices, topical use of gels and varnishes, have been used to decrease enamel demineralization and WSLs, but with inconsistent results . Almost all of these strategies need good long-term patient compliance or periodic use to be reliable . Resin-modified glass ionomer cements (RMGIs), possessing acceptable bonding strengths, have been widely used as orthodontic cements due to their outstanding F − release . Although recent studies have suggested that RMGIs could be beneficial in preventing demineralization , enamel WSLs are still prevalent after the treatments. The initial fluoride release is short-lived and its concentration in the oral cavity is extensively low due to salivary clearance and swallowing . Moreover, RMGIs were reported to gather more cariogenic biofilms than other adhesives due to their relatively rough surfaces . Therefore, a new bioactive orthodontic cement that can alleviate subsurface enamel demineralization adjacent to brackets, and without increasing its necessity for better patient compliance, is needed.
Once placed in the oral environment, an acquired pellicle containing absorbed salivary proteins attach to appliances, adhesives and neighboring tooth enamel . These proteins serve as receptors for, and are the prerequisite of, early bacterial colonization and subsequent biofilm formation . Therefore, it would be beneficial to develop a protein-repellent material. It has been demonstrated that dental materials with hydrophilic surfaces are resistant to protein adsorption and retention . 2-Metha-cryloyloxyethyl phosphorylcholine (MPC) is a methacrylate monomer having a phospholipid polar group in the side chain, and could repel protein adsorption . Recently, MPC was incorporated into RMGIs yielding protein-repellent effects . Another approach to prevent enamel demineralization is to develop antibacterial materials. Quaternary ammonium methacrylates (QAMs) were synthesized and incorporated into bonding agent . Dimethylaminohexadecyl methacrylate (DMAHDM) with an alkyl chain length of 16 was incorporated into composite resins, bonding adhesives and RMGIs, showing a potent antibacterial ability without compromising the mechanical properties of the resin .
Another key approach is calcium phosphate remineralization. Supersaturating levels of calcium (Ca) and phosphate (P) ions around enamel are necessary for inhibiting demineralization and promoting remineralization. Nanoparticles of amorphous calcium phosphate (NACP) were synthesized . Acid challenges could be quickly neutralized via a NACP-containing resin . Significant remineralization effect on enamel of human molars under the NACP composite in vitro was observed after demineralization treatment . Recently, NACP were incorporated into a RMGI which released Ca and P ions . However, to date, there has been no report on the development of an orthodontic cement containing MPC, DMAHDM and NACP that can effectively reduce or prevent enamel demineralization near orthodontic brackets under oral biofilm acid attacks.
The objective of this study is to investigate the demineralization preventive effect of novel multi-functional orthodontic cements around brackets in vitro. Extracted premolars were collected and analogous oral acid environment with mixed human saliva was imitated. The brackets were bonded with modified RMGI-based cements containing MPC, DMAHDM and NACP fillers, in comparison with the controls. It was hypothesized that: (1) The novel multi-functional cement with MPC, DMAHDM and NACP would successfully reduce enamel subsurface lesions adjacent to brackets under acid attack; (2) The effect of demineralization prevention by novel cement containing bioactive agents would be significantly better than that without the bioactive agents; (3) The novel cement would yield significantly greater enamel hardness when compared with a commercial RMGI control.
2
Materials and methods
2.1
Incorporation of MPC into RMGI
An RMGI cement (GC Ortho LC, Fuji, Aichi-ken, Japan) consisted of fluoroaluminosilicate glass particles, hydroxyethyl methacrylate (HEMA) monomer and aqueous polyalkenoic acid. A powder/liquid mass ratio of 2.5/1 was used according to the manufacturer. MPC was obtained commercially (Sigma-Aldrich, St. Louis, MO) which was synthesized via an approach by Ishihara et al. . The MPC powder was mixed into RMGI at a MPC/(RMGI + MPC) mass fraction of 3%, following a previous study .
2.2
Incorporation of DMAHDM into RMGI
DMAHDM with an alkyl chain length of 16 was synthesized via a modified Menschutkin reaction where a tertiary amine group was reacted with an organo-halide . A benefit of this reaction is that the reaction products are generated at quantitative amounts and require minimal purification . Briefly, 10 mmol of 2-(dimethylamino) ethyl methacrylate (DMAEMA, Sigma-Aldrich) and 10 mmol of 1-bromohexadecane (BHD, TCI America, Portland, OR) were combined with 3 g of ethanol in a 20 mL scintillation vial. The vial was stirred at 70 °C for 24 h. The solvent was then removed via evaporation, yielding DMAHDM as a clear and viscous liquid . The DMAHDM was mixed with RMGI at a DMAHDM/(RMGI + DMAHDM) mass fraction of 1.5%, following a previous study .
2.3
Incorporation of NACP into RMGI
NACP were synthesized via a spray-drying technique as described previously . Briefly, calcium carbonate and dicalcium phosphate anhydrous were dissolved into an acetic acid solution to achieve final Ca and P ionic concentrations of 8 mmol/L and 5.333 mmol/L, which led to Ca/P molar mass ratio was 1.5, the same as that for ACP. This solution was sprayed into a heated chamber apparatus, and the dried particles were collected via an electrostatic precipitator (AirQuality, Minneapolis, MN), producing NACP with a mean particle size of 116 nm measured before .
Adding NACP powder into the RMGI paste significantly increased the paste viscosity. Therefore, to maintain a viscosity similar to that of the commercial RMGI, photo-activated resin monomers were added together with NACP into RMGI. The monomers consisted of bisphenol A glycidyl dimethacrylate (BisGMA) and triethylene glycol dimethacrylate (TEGDMA) (Esstech, Essington, PA) at a 1:1 mass ratio, with 0.2% camphorquinone and 0.8% ethyl 4-N,Ndimathylaminobenzoate. The BisGMA-TEGDMA resin (referred to as BT) at a BT/(final cement) mass fraction of 15%, as well as NACP at a NACP/(final cement) mass fraction of 20% was mixed to form the BT-NACP paste. Meanwhile, MPC at a MPC/(final cement) mass fraction of 3% and DMAHDM at a DMAHDM/(final cement) mass fraction of 1.5% were mixed into the powder of the RMGI to form the RMGI-MPC-DMAHDM blend. Then, this blend was mixed with the BT-NACP paste to form the final RMGI + MPC + DMAHDM + NACP cement. The percentages of each component were selected following a previous study . This yielded a modified RMGI paste containing BT and NACP that had an acceptable viscosity for the final paste and good enamel bond strength.
2.4
Preparation of orthodontic bracket-enamel bonded samples
Nighty-six freshly extracted, caries-free premolars for orthodontic reasons were collected and randomly assigned to four groups shown in Table 1 (n = 24 per group). The use of extracted human teeth has been approved by the Ethical Committee of Beijing Stomatological Hospital and the Institutional Review Board of University of Maryland in Baltimore. The selection criteria for teeth included intact buccal enamel without WSLs, and without visible cracks or enamel irregularities. Teeth were cleaned and stored in 0.1% thymol solution at 4 °C before use within 1 month. For each group, twenty-four teeth were randomly divided into two groups of 12 teeth each. One group was used for the shear bond strength test, and the other group for the demineralization experiment. The four types of cements are listed below:
- (1)
Transbond XT control (referred to as TB control);
- (2)
RMGI (GC Ortho LC, Fuji);
- (3)
95.5% RMGI + 3% MPC + 1.5% DMAHDM (referred to as “RMGI + MPC + DMAHDM”);
- (4)
60.5% RMGI + 3% MPC + 1.5% DMAHDM + 20% NACP + 15% BisGMA-TEGDMA (referred to as “RMGI + MPC + DMAHDM + NACP”).
Group | F − | Protein-repellent | Antimicrobial | Ca-P remineralization | |
---|---|---|---|---|---|
I | Transbond XT (TB control) | − | − | − | − |
II | RMGI | + | − | − | − |
III | RMGI + MPC + DMAHDM | + | + | + | − |
IV | RMGI + MPC + DMAHDM + NACP | + | + | + | + |
According to the manufacturer, Transbond XT (3 M Unitek, Saint Paul, MS, USA) contained silane-treated quartz, bisphenol-A-diglycidyl ether dimethacrylate, bisphenol-A-bis (2-hydroxyethyl) dimethacrylate, and silane-treated silica. For enamel shear bond test, each tooth was embedded in a self-curing acrylic resin (New Century Dental Materials, Shanghai, China) perpendicularly to the buccal axis of the crown, in order that the buccal surface would be parallel to the force during testing ( Fig. 1 A). Enamel was etched for 30 s with 37% phosphoric acid gel (3 M Unitek). The tooth was dried with air stream until a chalky appearance was observed . Standard straight-wire premolar brackets (OPK-A, Tomy, Fukushima-ken, Japan) were bonded according to manufacturers’ instructions and literature for Transbond control and GC control . The bonding procedure for the two novel groups was the same as that for GC control. To make the cement thickness consistent for each sample, a 3.0 N force was applied vertically on the bracket for 5 s via an FGP-0.5 gauge (SHIMPO, Japan). The samples were light-cured for a total 40 s from mesial and distal sides at a 45° angle and a distance of 2 mm to the enamel surface (Elipar™ S10 LED, 3 M Unitek, Saint Paul, MS, USA).

For demineralization test, the roots were cut off via a water-cooled diamond bur. Coronal pulp was removed, then the pulp cavities were sealed by wax. To prevent the surrounding enamel from being etched, a piece of tape with a rectangular cut-out window of the size of the bracket base was used. The etching and bracket bonding procedures were the same as the samples in the shear bond test. Then, each bracket-enamel bonded sample was painted with an acid-resistant nail varnish around the bracket, leaving a 1 mm space of exposed enamel ( Fig. 1 B). All the samples were disinfected with ethylene oxide and de-gassed for 3 d (Anprolene AN 74i, Andersen, Haw River, NC).
2.5
Enamel shear bond strength (SBS) test
The bracket-bonded samples of each group (n = 12) were stored in distilled water at 37 °C for 24 h, and then they were used for shear bond testing. A chisel shear tester was connected to a computer-controlled Universal Testing Machine (AG-IS 500N, SHIMADZU Corporation, Japan). Each embedded sample was fixed on the working stage, and the chisel tip was carefully adjusted and positioned on the upper part of the bracket base ( Fig. 1 A). An occlusal-gingival load was applied at a displacement rate of 0.5 mm/min until the bracket bond failed. The enamel shear bond strength (SBS) = the debonding force/bracket contact surface area, following previous studies .
2.6
Dental plaque microcosm biofilm model for enamel demineralization
Human saliva and sucrose were used to establish an in-vitro dental plaque microcosm biofilm model . This model was confirmed to have the capability to produce acidic pH due to polymicrobial biofilm growth and acid production . Saliva was collected from ten healthy adult donors having natural dentition without active caries or periopathology, and without the application of antibiotics within the last 3 months. The donors did not carry out any procedures of oral hygiene for 24 h and abstained from food and drink intake for 2 h prior to donating saliva. An identical volume of saliva from each donor was blended to form the saliva sample. The saliva was then diluted in sterile glycerol to a concentration of 70%, and stored at −80 °C before use .
The culture medium contained mucin (type II, porcine, gastric) at a concentration of 2.5 g/L; bacteriological peptone, 2.0 g/L; tryptone, 2.0 g/L; yeast extract, 1.0 g/L; NaCl, 0.35 g/L; KCl, 0.2 g/L; CaCl 2 , 0.2 g/L; cysteine hydrochloride, 0.1 g/L; hemin, 0.001 g/L; vitamin K1, 0.0002 g/L, at pH 7. In each well of 24-well flat-bottomed plates, 1.5 mL of medium with the saliva-glycerol mixture (1:50 of volume) and 0.2% sucrose was added as inoculum. To ensure biofilm standardized growth, the inoculum was first incubated anaerobically at 37 °C for 8 h, then refreshed and incubated for another 16 h, for a total of 24 h. A previous study showed that an acidic environment could be obtained by biofilm metabolism after 24 h incubation . Then, each prepared crown sample was placed into a well with medium for continuous 14 d demineralization, while the medium was changed every 24 h ( Fig. 1 C).
2.7
Polarized-light microscopy (PLM)
After the aforementioned biofilm acid challenge for 14 days, the demineralized samples were taken out and rinsed with deionized water. Each sample was buccolingually sectioned into two equivalent parts using a diamond rim saw ( Fig. 1 D). They were randomly divided into two groups. Group A was used for PLM. Group B was used for Section 2.8 . Samples in group A were dehydrated by gradient ethanol solutions (at 90%, 95% and 100%), and the varnish was rinsed off with acetone. Each sample was embedded and then segmented parallel to the buccal-lingual axis using a hard-tissue cutting system (E300CP, EXAKT, Germany) into a cross-sectional slice and polished with 800- to 4000-grit abrasive papers (Hermes, Hamburg, Germany) using a micro-grinding system (E400CS, EXAKT, Germany). Finally, an 80 to 100 μm thickness slice was derived for lesion depth evaluation using a polarized-light microscope (Axio Scope A1, Zeiss, Germany). Regions of demineralization at 25× magnification were centered and photographed ( Fig. 1 E). For each slice, the lesion depth was measured at three distance ranges, from 50 to 150 μm, 150 to 250 μm, and 250 to 350 μm, respectively, to the margin of the adhesive. The three ranges were marked with the digital segment template lines of isometric widths by Image Pro Plus 6.0 (Media Cybernetic, Silver Spring, MD) software. The average lesion depth for each range was measured as the area of the body of the lesion divided by the width of the template line.
2.8
Cross-sectional hardness testing
Each sample of group B was stick to a glass slide via a thin layer of self-curing resin (Technovit 4000, Heraeus Kulzer GmbH, Germany) using a parallel carrier sheet pressing device (E401, EXAKT, Germany). The cross-sectional surfaces were serially polished to 4000-grit. This process was to ensure that the tested surface was parallel to the platform of the hardness tester (FM-700, Future-Tech, Kawasaki, Japan). A Knoop indenter was used to measure the hardness KHN at a force of 25 g and a dwell time of 5 s. On each sample, three lanes of 24 indentations were made on three equal interval distances, at 100, 200, and 300 μm away from the adhesive margin. Eight indentations for each lane from the external side to the internal side were made at 25, 50, 75, 100, 125, 150, 175, and 200 μm depth ( Fig. 1 F). Another lane of indentations was made on sound enamel beneath the varnish-covered region as the hardness baseline. Integrated demineralization (ΔS) of each sample was calculated by the following steps. First, all the lanes of KHN values in the experimental region and the baseline were obtained. Second, these data were transformed into mineral content percent values that were linearly proportional to the square root of KHN, according to the relation between hardness and transverse microradiography (TMR) . Then, the mineral content percentage was plotted against the depths to obtain the integrated hardness profile. ΔS was computed based on the profile area of the demineralized enamel and subtracted from the area of sound enamel, following a previous study .
2.9
Scanning electron microscopy (SEM)
Samples after hardness testing were removed from glass slides. They were sputter-coated with gold and examined via a scanning electron microscope (SEM, EVO MA25, Zeiss, Oberkochen, Germany). SEM images were obtained at 100 to 300 μm from the adhesive margin.
2.10
Statistical analysis
SBS, PLM and hardness results were statistically examined with SPSS 17.0 for Windows (SPSS Inc. Chicago, IL, USA). The normal distribution assumption and Levene’s homogeneity test were confirmed for the dependent variables. For SBS and lesion depths of PLM, one-way ANOVA was performed. For ΔS and hardness, ANOVA with Tukey post-hoc tests were used. The significance level of p was set at 0.05.
2
Materials and methods
2.1
Incorporation of MPC into RMGI
An RMGI cement (GC Ortho LC, Fuji, Aichi-ken, Japan) consisted of fluoroaluminosilicate glass particles, hydroxyethyl methacrylate (HEMA) monomer and aqueous polyalkenoic acid. A powder/liquid mass ratio of 2.5/1 was used according to the manufacturer. MPC was obtained commercially (Sigma-Aldrich, St. Louis, MO) which was synthesized via an approach by Ishihara et al. . The MPC powder was mixed into RMGI at a MPC/(RMGI + MPC) mass fraction of 3%, following a previous study .
2.2
Incorporation of DMAHDM into RMGI
DMAHDM with an alkyl chain length of 16 was synthesized via a modified Menschutkin reaction where a tertiary amine group was reacted with an organo-halide . A benefit of this reaction is that the reaction products are generated at quantitative amounts and require minimal purification . Briefly, 10 mmol of 2-(dimethylamino) ethyl methacrylate (DMAEMA, Sigma-Aldrich) and 10 mmol of 1-bromohexadecane (BHD, TCI America, Portland, OR) were combined with 3 g of ethanol in a 20 mL scintillation vial. The vial was stirred at 70 °C for 24 h. The solvent was then removed via evaporation, yielding DMAHDM as a clear and viscous liquid . The DMAHDM was mixed with RMGI at a DMAHDM/(RMGI + DMAHDM) mass fraction of 1.5%, following a previous study .
2.3
Incorporation of NACP into RMGI
NACP were synthesized via a spray-drying technique as described previously . Briefly, calcium carbonate and dicalcium phosphate anhydrous were dissolved into an acetic acid solution to achieve final Ca and P ionic concentrations of 8 mmol/L and 5.333 mmol/L, which led to Ca/P molar mass ratio was 1.5, the same as that for ACP. This solution was sprayed into a heated chamber apparatus, and the dried particles were collected via an electrostatic precipitator (AirQuality, Minneapolis, MN), producing NACP with a mean particle size of 116 nm measured before .
Adding NACP powder into the RMGI paste significantly increased the paste viscosity. Therefore, to maintain a viscosity similar to that of the commercial RMGI, photo-activated resin monomers were added together with NACP into RMGI. The monomers consisted of bisphenol A glycidyl dimethacrylate (BisGMA) and triethylene glycol dimethacrylate (TEGDMA) (Esstech, Essington, PA) at a 1:1 mass ratio, with 0.2% camphorquinone and 0.8% ethyl 4-N,Ndimathylaminobenzoate. The BisGMA-TEGDMA resin (referred to as BT) at a BT/(final cement) mass fraction of 15%, as well as NACP at a NACP/(final cement) mass fraction of 20% was mixed to form the BT-NACP paste. Meanwhile, MPC at a MPC/(final cement) mass fraction of 3% and DMAHDM at a DMAHDM/(final cement) mass fraction of 1.5% were mixed into the powder of the RMGI to form the RMGI-MPC-DMAHDM blend. Then, this blend was mixed with the BT-NACP paste to form the final RMGI + MPC + DMAHDM + NACP cement. The percentages of each component were selected following a previous study . This yielded a modified RMGI paste containing BT and NACP that had an acceptable viscosity for the final paste and good enamel bond strength.
2.4
Preparation of orthodontic bracket-enamel bonded samples
Nighty-six freshly extracted, caries-free premolars for orthodontic reasons were collected and randomly assigned to four groups shown in Table 1 (n = 24 per group). The use of extracted human teeth has been approved by the Ethical Committee of Beijing Stomatological Hospital and the Institutional Review Board of University of Maryland in Baltimore. The selection criteria for teeth included intact buccal enamel without WSLs, and without visible cracks or enamel irregularities. Teeth were cleaned and stored in 0.1% thymol solution at 4 °C before use within 1 month. For each group, twenty-four teeth were randomly divided into two groups of 12 teeth each. One group was used for the shear bond strength test, and the other group for the demineralization experiment. The four types of cements are listed below:
- (1)
Transbond XT control (referred to as TB control);
- (2)
RMGI (GC Ortho LC, Fuji);
- (3)
95.5% RMGI + 3% MPC + 1.5% DMAHDM (referred to as “RMGI + MPC + DMAHDM”);
- (4)
60.5% RMGI + 3% MPC + 1.5% DMAHDM + 20% NACP + 15% BisGMA-TEGDMA (referred to as “RMGI + MPC + DMAHDM + NACP”).
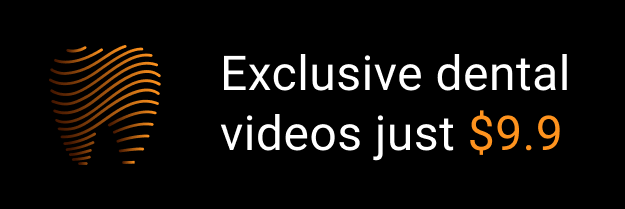