Abstract
Objectives
Secondary caries is the main reason for restoration failure, and replacement of the failed restorations accounts for 50–70% of all restorations. Antibacterial adhesives could inhibit residual bacteria in tooth cavity and invading bacteria along the margins. Calcium (Ca) and phosphate (P) ion release could remineralize the lesions. The objectives of this study were to incorporate nanoparticles of silver (NAg) and nanoparticles of amorphous calcium phosphate (NACP) into adhesive for the first time, and to investigate the effects on dentin bond strength and plaque microcosm biofilms.
Methods
Scotchbond multi-purpose adhesive was used as control. NAg were added into primer and adhesive at 0.1% by mass. NACP were mixed into adhesive at 10%, 20%, 30% and 40%. Microcosm biofilms were grown on disks with primer covering the adhesive on a composite. Biofilm metabolic activity, colony-forming units (CFU) and lactic acid were measured.
Results
Human dentin shear bond strengths ( n = 10) ranged from 26 to 34 MPa; adding NAg and NACP into adhesive did not decrease the bond strength ( p > 0.1). SEM examination revealed resin tags from well-filled dentinal tubules. Numerous NACP infiltrated into the dentinal tubules. While NACP had little antibacterial effect, NAg in bonding agents greatly reduced the biofilm viability and metabolic activity, compared to the control ( p < 0.05). CFU for total microorganisms, total streptococci, and mutans streptococci on bonding agents with NAg were an order of magnitude less than those of the control. Lactic acid production by biofilms for groups containing NAg was 1/4 of that of the control.
Significance
Dental plaque microcosm biofilm viability and acid production were greatly reduced on bonding agents containing NAg and NACP, without compromising dentin bond strength. The novel method of incorporating dual agents (remineralizing agent NACP and antibacterial agent NAg) may have wide applicability to other dental bonding systems.
1
Introduction
Extensive studies have been undertaken to improve dental composites with advances in filler compositions and resin chemistry . The property enhancements have enabled composites to be increasingly used as esthetic filling materials . Indeed, recent data showed that composite restorations represented the highest percentage among three categories: 77.3 million restorations (46.6%) were composites, 52.5 million (31.6%) were amalgams, and 36.2 million (21.8%) were crowns, totaling 166 million restorations placed in the USA in 2005 . However, a major shortcoming of composites is that they accumulate more biofilms and plaques in vivo , compared to other restorative materials . The acid production by biofilms can lead to secondary caries especially at the tooth-restoration margins . Recurrent caries is the main reason for restoration failure, and replacement of the failed restorations accounts for 50–70% of all restorations performed .
To address this problem, novel antibacterial dental composites were developed . Quaternary ammonium monomers, such as 12-methacryloyloxydodecylpyridinium bromide (MDPB), were copolymerized in resins to yield antibacterial activities . Another class of antibacterial composites incorporated silver (Ag) particles . For example, a composite containing Ag inhibited S. mutans growth and had a long-lasting antibacterial activity . Ag nanoparticles were highly effective for antibacterial applications . Resins containing Ag nanoparticles were recently reported that inhibited biofilm viability and growth .
The development of composites containing calcium phosphate (CaP) particles with remineralization capabilities represents an important approach to inhibiting secondary caries . These composites could release supersaturating levels of calcium (Ca) and phosphate (P) ions and remineralize enamel and dentin lesions . Traditional CaP composites contained CaP particles of several microns in sizes . Recently, CaP nanoparticles of sizes of about 100 nm were synthesized via a spray-drying technique and filled into dental composites . These nanocomposites achieved Ca and P ion release similar to those of traditional CaP composites, while possessing much better mechanical properties .
Besides composites, it is also important to develop adhesives capable of inhibiting secondary caries. Adhesives bond the composite to dentin and infiltrate into dentinal tubules and a demineralized layer to form an interlocked interface . Vast efforts have been made to enhance adhesive bonding to the tooth structure . Clinically, residual bacteria could exist in the prepared tooth cavity. In addition, microleakage could allow bacteria to invade the tooth-restoration interfaces. Therefore, it is desirable for the adhesive to be antibacterial to inhibit recurrent caries . Antibacterial agents such as MDPB , methacryloxylethyl cetyl dimethyl ammonium chloride , and cetylpyridinium chloride were used to develop antibacterial adhesives. In addition, dental primer directly contacts the tooth structure and could kill residual bacterial if rendered antibacterial . However, a literature search revealed only a small number of publications on antibacterial adhesives and primers . While nanoparticles of amorphous calcium phosphate (NACP) were incorporated into composite with Ca and P ion release , and the release of Ca and P ions were shown to remineralize tooth lesions , NACP have not been incorporated into bonding agents.
The objective of this study was to incorporate NAg and NACP into a bonding system for the first time, and to investigate the antibacterial properties using a dental plaque microcosm biofilm model. While previous studies established the Ca and P ion release with enamel and dentin remineralization , the present study focused on the effect of NAg and NACP on dentin bond strength and antibacterial activity. The hypotheses were: (1) incorporating NACP at mass fractions of 10–40% into the adhesive would not decrease the shear bond strength to human dentin, compared to control with 0% NACP; (2) incorporating NAg into primer and adhesive would not decrease the dentin shear bond strength; (3) NACP (being a remineralizing agent) would have little antibacterial effect, while NAg would have a strong antibacterial effect.
2
Materials and methods
2.1
NAg incorporation into primer and adhesive
Scotchbond multi-purpose (3M, St. Paul, MN), referred to as “SBMP”, was used as the parent bonding system to test the effect of incorporation of NACP and NAg. The purpose was to investigate a model system, and then the method of incorporating NACP and NAg could be applied to other bonding agents. According to the manufacturer, SBMP etchant contained 35% phosphoric acid. SBMP primer single bottle contained 35–45% 2-hydroxyethylmethacrylate (HEMA), 10–20% copolymer of acrylic and itaconic acids, and 40–50% water. SBMP adhesive contained 60–70% BisGMA and 30–40% HEMA.
Silver 2-ethylhexanoate powder (Strem, New Buryport, MA) was dissolved in 2-( tert -butylamino)ethyl methacrylate (TBAEMA, Sigma) at 0.08 g of silver salt per 1 g of TBAEMA, following previous studies . TBAEMA was used because it improves the solubility by forming Ag-N coordination bonds with Ag ions, thereby facilitating the Ag salt to dissolve in the resin solution. TBAEMA was selected since it contains reactive methacrylate groups and can be chemically incorporated into a resin upon photopolymerization. This method produced NAg with a mean particle size of 2.7 nm that were well dispersed in the resin . To incorporate NAg into the primer, the aforementioned Ag-TBAEMA solution was mixed with the SBMP primer at a silver 2-ethylhexanoate/(primer + silver 2-ethylhexanoate) of 0.1% by mass; this mass fraction was selected based on previous studies . To incorporate NAg into the adhesive, the Ag-TBAEMA was mixed with the SBMP adhesive at 0.1% mass fraction.
2.2
Addition of NACP into adhesive
Nanoparticles of ACP (Ca 3 [PO 4 ] 2 ) were synthesized using a spray-drying technique as described previously . Briefly, calcium carbonate (CaCO 3 , Fisher, Fair Lawn, NJ) and dicalcium phosphate anhydrous (CaHPO 4 , Baker Chemical, Phillipsburg, NJ) were dissolved into an acetic acid solution to obtain final Ca and P ionic concentrations of 8 mmol/L and 5.333 mmol/L, respectively. The Ca/P molar ratio for the solution was 1.5, the same as that for ACP. The solution was sprayed into the heated chamber of the spray-drying apparatus. The dried particles were collected via an electrostatic precipitator (AirQuality, Minneapolis, MN), yielding NACP with a mean particle size of 116 nm .
The NACP were mixed with the adhesive containing 0.1% silver 2-ethylhexanoate. The NACP mass fractions in the adhesive were: 0%, 10%, 20%, 30%, and 40%, following previous studies on NACP nanocomposites . To compare the differences in the viscosity of the various adhesive resin-NACP pastes, a previous flow method was adapted, because the flow of the paste is inversely related to the viscosity . Briefly, 0.1 g of each adhesive paste was placed on a glass slide and the diameter of the adhesive drop was measured, yielding the area A before . A second glass slide was placed on the top, a 100 g weight was applied for 5 min for the paste to flow, and the diameter of the adhesive paste was measured again, yielding the area A after . The change in area was calculated: Δ A = ( A after − A before )/ A before . A smaller Δ A corresponded to a higher viscosity. Six measurements were made for each adhesive ( n = 6).
2.3
Dentin shear bond strength testing and SEM examination
Six bonding agents were tested:
- [1].
SBMP primer, SBMP adhesive (termed “SBMP control”).
- [2].
Primer + 0.1% NAg, adhesive + 0.1% NAg (termed “P&A + NAg”. P = primer, A = adhesive).
- [3].
Primer + 0.1% NAg, adhesive + 0.1% NAg + 10% NACP (“P + NAg, A + NAg + 10NACP”).
- [4].
Primer + 0.1% NAg, adhesive + 0.1% NAg + 20% NACP (“P + NAg, A + NAg + 20NACP”).
- [5].
Primer + 0.1% NAg, adhesive + 0.1% NAg + 30% NACP (“P + NAg, A + NAg + 30NACP”).
- [6].
Primer + 0.1% NAg, adhesive + 0.1% NAg + 40% NACP (“P + NAg, A + NAg + 40NACP”).
Extracted caries-free human third molars were cleaned and stored in 0.01% thymol solution. The tips of the molar crowns were cut off via a diamond saw (Isomet, Buehler, Lake Bluff, IL) to yield flat mid-coronal dentin surfaces. Following a previous study , the tooth was embedded in a poly-carbonate holder (Bosworth, Skokie, IL) and ground perpendicular to the longitudinal axis using 320-grit SiC paper until there was no occlusal enamel left. The bonding procedures are shown in Fig. 1 A. Briefly, the dentin surface was etched with phosphoric acid gel for 15 s and rinsed with water for 15 s . The primer was applied with a brush-tipped applicator and rubbed in for 15 s, and the solvent was removed with a stream of air. The adhesive was then applied and photo-cured for 10 s (Optilux VCL 401, Demetron Kerr, Danbury, CT). Then, a stainless-steel iris with a central opening (diameter = 4 mm, thickness = 1.5 mm) was held against the adhesive-treated dentin surface. The central opening was filled with a composite (TPH, Caulk/Dentsply, Milford, DE) and photo-cured for 60 s.
The bonded specimens were stored in distilled water at 37 °C for 24 h . Then, the dentin shear bond strength, S D , was measured as schematically shown in Fig. 1 B. The chisel was connected with a computer-controlled Universal Testing Machine (MTS, Eden Prairie, MN), held parallel to the composite-dentin interface, and loaded at 0.5 mm/min until the bond failed. S D was calculated as: S D = 4 P /( πd 2 ), where P is the load at failure, and d is the diameter of the composite. Ten teeth were tested for each group ( n = 10).
For scanning electron microscopy (SEM) examination, the bonded tooth was cut through the center parallel to the longitudinal axis via a diamond saw (Isomet) with copious water. The sectioned surface was polished with increasingly finer SiC paper up to 4000 grit. Following a previous study , the polished surface was treated with 50% phosphoric acid for 30 s, then with 5% NaOCl for 10 min. After being thoroughly rinsed with water for 10 min, the specimens were air dried and then sputter-coated with gold. Three specimens were prepared for each group. The specimens were then examined in an SEM (Quanta 200, FEI, Hillsboro, OR).
2.4
Specimen fabrication for biofilm experiments
Following previous studies , layered disk specimens were made as shown in Fig. 3 A. A polyethylene mold (inner diameter = 9 mm, thickness = 2 mm) was situated on a glass slide. A primer was applied into the mold to cover the glass. After drying with a stream of air, an adhesive was applied and cured for 20 s with Optilux. A composite (TPH) was placed on the adhesive to completely fill the mold, and light-cured for 1 min. The bonded specimens were immersed in water and agitated for 1 h to remove any uncured monomer . The disks were then dried and sterilized with ethylene oxide (Anprolene AN 74i, Andersen, Haw River, NC).
2.5
Dental plaque microcosm model and live/dead assay
Saliva is ideal for growing dental plaque microcosm biofilms in vitro which maintain much of the complexity and heterogeneity of dental plaque in vivo . The dental plaque microcosm model was approved by the University of Maryland. Saliva was collected from a healthy adult donor having natural dentition without active caries or periopathology, and without the use of antibiotics within the last 3 months . The donor did not brush teeth for 24 h and abstained from food/drink intake for at least 2 h prior to donating saliva. Stimulated saliva was collected during parafilm chewing and kept on ice. The saliva was diluted in sterile glycerol to a concentration of 70%, and stored at −80 °C .
The saliva-glycerol stock was added, with 1:50 final dilution, to a growth medium as inoculum. The growth medium contained mucin (type II, porcine, gastric) at a concentration of 2.5 g/L; bacteriological peptone, 2.0 g/L; tryptone, 2.0 g/L; yeast extract, 1.0 g/L; NaCl, 0.35 g/L, KCl, 0.2 g/L; CaCl 2 , 0.2 g/L; cysteine hydrochloride, 0.1 g/L; haemin, 0.001 g/L; vitamin K1, 0.0002 g/L, at pH 7 . The inoculum was cultured in an incubator (5% CO 2 , 37 °C) for 24 h. Each disk was placed into a well of 24-well plates, with the primer on the top. Then, 1.5 mL of inoculum was added to each well, and incubated for 8 h. The disks were transferred to new 24-well plates with fresh medium and incubated. After 16 h, the disks were transferred to new 24-well plates with fresh medium and incubated for 24 h. This totalled 2 d of incubation, which was shown in a previous study to be sufficient to form microcosm biofilms .
Disks with 2-d biofilms were washed three times with phosphate buffered saline (PBS), and then stained using a live/dead bacterial kit (Molecular Probes, Eugene, OR). Live bacteria were stained with Syto 9 to produce a green fluorescence, and bacteria with compromised membranes were stained with propidium iodide to produce a red fluorescence. Specimens were examined with an epifluorescence microscope (TE2000-S, Nikon, Melville, NY). The area of green staining (live bacteria) was computed using NIS Elements imaging software (Nikon). The area fraction of live bacteria = green staining area/total area of the image. Six specimens were used for each group ( n = 6). Three randomly chosen fields of view were photographed from each specimen yielding a total of 18 images for each group.
2.6
MTT assay of metabolic activity
MTT (3-[4,5-dimethylthiazol-2-yl]-2,5-diphenyltetrazolium bromide) assay was used to measure the metabolic activity of biofilms . MTT is a colorimetric assay that measures the enzymatic reduction of MTT, a yellow tetrazole, to formazan. Each disk with the 2-d biofilm was transferred to a new 24-well plate. One mL of MTT dye (0.5 mg/mL MTT in PBS) was added to each well and incubated for 1 h. During this process, metabolically active bacteria reduced the MTT to purple formazan. After 1 h, the disks were transferred to a new 24-well plate, 1 mL of dimethyl sulfoxide (DMSO) was added to solubilize the formazan crystals, and the plate was incubated for 20 min in the dark. After mixing via pipetting, 200 μL of the DMSO solution from each well was transferred to a 96-well plate, and the absorbance at 540 nm (optical density OD540) was measured via a microplate reader (SpectraMax M5, Molecular Devices, Sunnvale, CA). A higher absorbance is related to a higher formazan concentration, which indicates a higher metabolic activity in the biofilm adherent on the disk.
2.7
Lactic acid production and colony-forming unit (CFU) counts
Disks with 2-d biofilms were rinsed with cysteine peptone water (CPW) to remove loose bacteria, and transferred to 24-well plates containing buffered peptone water (BPW) plus 0.2% sucrose. The samples were incubated for 3 h to allow the biofilms to produce acid. The BPW solutions were then stored for lactate analysis. Lactate concentrations were determined using an enzymatic (lactate dehydrogenase) method . The microplate reader was used to measure the absorbance at 340 nm (optical density OD 340 ) for the collected BPW solutions. Standard curves were prepared using a lactic acid standard (Supelco, Bellefonte, PA).
Disks with biofilms were transferred into tubes with 2 mL CPW, and the biofilms were harvested by sonication and vortexing via a vortex mixer (Fisher, Pittsburgh, PA). Three types of agar plates were used. First, tryptic soy blood agar culture plates were used to determine total microorganisms . Second, mitis salivarius agar (MSA) culture plates, containing 15% sucrose, were used to determine total streptococci . This is because MSA contains selective agents crystal violet, potassium tellurite and trypan blue, which inhibit most Gram-negative bacilli and Gram-positive bacteria except streptococci, thus enabling streptococci to grow . Third, cariogenic mutans streptococci are known to be resistant to bacitracin, and this property was used to isolate mutans streptococci from the oral microflora. The MSA agar plates with 0.2 units of bacitracin per mL were used to determine mutans streptococci.
One-way analysis of variance (ANOVA) was performed to detect the significant effects of the variables. Tukey’s multiple comparison was used to compare the data at a p value of 0.05.
2
Materials and methods
2.1
NAg incorporation into primer and adhesive
Scotchbond multi-purpose (3M, St. Paul, MN), referred to as “SBMP”, was used as the parent bonding system to test the effect of incorporation of NACP and NAg. The purpose was to investigate a model system, and then the method of incorporating NACP and NAg could be applied to other bonding agents. According to the manufacturer, SBMP etchant contained 35% phosphoric acid. SBMP primer single bottle contained 35–45% 2-hydroxyethylmethacrylate (HEMA), 10–20% copolymer of acrylic and itaconic acids, and 40–50% water. SBMP adhesive contained 60–70% BisGMA and 30–40% HEMA.
Silver 2-ethylhexanoate powder (Strem, New Buryport, MA) was dissolved in 2-( tert -butylamino)ethyl methacrylate (TBAEMA, Sigma) at 0.08 g of silver salt per 1 g of TBAEMA, following previous studies . TBAEMA was used because it improves the solubility by forming Ag-N coordination bonds with Ag ions, thereby facilitating the Ag salt to dissolve in the resin solution. TBAEMA was selected since it contains reactive methacrylate groups and can be chemically incorporated into a resin upon photopolymerization. This method produced NAg with a mean particle size of 2.7 nm that were well dispersed in the resin . To incorporate NAg into the primer, the aforementioned Ag-TBAEMA solution was mixed with the SBMP primer at a silver 2-ethylhexanoate/(primer + silver 2-ethylhexanoate) of 0.1% by mass; this mass fraction was selected based on previous studies . To incorporate NAg into the adhesive, the Ag-TBAEMA was mixed with the SBMP adhesive at 0.1% mass fraction.
2.2
Addition of NACP into adhesive
Nanoparticles of ACP (Ca 3 [PO 4 ] 2 ) were synthesized using a spray-drying technique as described previously . Briefly, calcium carbonate (CaCO 3 , Fisher, Fair Lawn, NJ) and dicalcium phosphate anhydrous (CaHPO 4 , Baker Chemical, Phillipsburg, NJ) were dissolved into an acetic acid solution to obtain final Ca and P ionic concentrations of 8 mmol/L and 5.333 mmol/L, respectively. The Ca/P molar ratio for the solution was 1.5, the same as that for ACP. The solution was sprayed into the heated chamber of the spray-drying apparatus. The dried particles were collected via an electrostatic precipitator (AirQuality, Minneapolis, MN), yielding NACP with a mean particle size of 116 nm .
The NACP were mixed with the adhesive containing 0.1% silver 2-ethylhexanoate. The NACP mass fractions in the adhesive were: 0%, 10%, 20%, 30%, and 40%, following previous studies on NACP nanocomposites . To compare the differences in the viscosity of the various adhesive resin-NACP pastes, a previous flow method was adapted, because the flow of the paste is inversely related to the viscosity . Briefly, 0.1 g of each adhesive paste was placed on a glass slide and the diameter of the adhesive drop was measured, yielding the area A before . A second glass slide was placed on the top, a 100 g weight was applied for 5 min for the paste to flow, and the diameter of the adhesive paste was measured again, yielding the area A after . The change in area was calculated: Δ A = ( A after − A before )/ A before . A smaller Δ A corresponded to a higher viscosity. Six measurements were made for each adhesive ( n = 6).
2.3
Dentin shear bond strength testing and SEM examination
Six bonding agents were tested:
- [1].
SBMP primer, SBMP adhesive (termed “SBMP control”).
- [2].
Primer + 0.1% NAg, adhesive + 0.1% NAg (termed “P&A + NAg”. P = primer, A = adhesive).
- [3].
Primer + 0.1% NAg, adhesive + 0.1% NAg + 10% NACP (“P + NAg, A + NAg + 10NACP”).
- [4].
Primer + 0.1% NAg, adhesive + 0.1% NAg + 20% NACP (“P + NAg, A + NAg + 20NACP”).
- [5].
Primer + 0.1% NAg, adhesive + 0.1% NAg + 30% NACP (“P + NAg, A + NAg + 30NACP”).
- [6].
Primer + 0.1% NAg, adhesive + 0.1% NAg + 40% NACP (“P + NAg, A + NAg + 40NACP”).
Extracted caries-free human third molars were cleaned and stored in 0.01% thymol solution. The tips of the molar crowns were cut off via a diamond saw (Isomet, Buehler, Lake Bluff, IL) to yield flat mid-coronal dentin surfaces. Following a previous study , the tooth was embedded in a poly-carbonate holder (Bosworth, Skokie, IL) and ground perpendicular to the longitudinal axis using 320-grit SiC paper until there was no occlusal enamel left. The bonding procedures are shown in Fig. 1 A. Briefly, the dentin surface was etched with phosphoric acid gel for 15 s and rinsed with water for 15 s . The primer was applied with a brush-tipped applicator and rubbed in for 15 s, and the solvent was removed with a stream of air. The adhesive was then applied and photo-cured for 10 s (Optilux VCL 401, Demetron Kerr, Danbury, CT). Then, a stainless-steel iris with a central opening (diameter = 4 mm, thickness = 1.5 mm) was held against the adhesive-treated dentin surface. The central opening was filled with a composite (TPH, Caulk/Dentsply, Milford, DE) and photo-cured for 60 s.
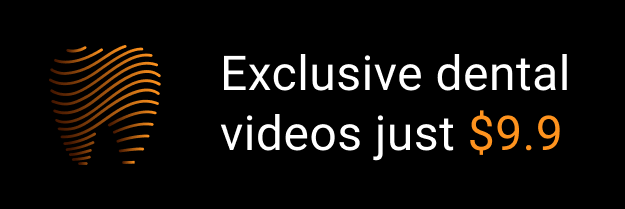