Abstract
Objectives
Secondary caries at the restoration margins remains the main reason for failure. Although calcium phosphate (CaP) composites are promising for caries inhibition, there has been no report of CaP composite to inhibit caries in situ. The objectives of this study were to investigate the caries-inhibition effect of nanocomposite containing nanoparticles of amorphous calcium phosphate (NACP) in a human in situ model for the first time, and to determine colony-forming units (CFU) and Ca and P ion concentrations of biofilms on the composite restorations.
Methods
NACP with a mean particle size of 116 nm were synthesized via a spray-drying technique. Two composites were fabricated: NACP nanocomposite, and control composite filled with glass particles. Twenty-five volunteers wore palatal devices containing bovine enamel slabs with cavities restored with NACP or control composite. After 14 days, the adherent biofilms were collected for analyses. Transverse microradiography determined the enamel mineral profiles at the margins, and the enamel mineral loss Δ Z was measured.
Results
NACP nanocomposite released Ca and P ions and the release significantly increased at cariogenic low pH ( p < 0.05). Biofilms on NACP nanocomposite contained higher Ca ( p = 0.007) and P ions ( p = 0.005) than those of control ( n = 25). There was no significant difference in biofilm CFU between the two composites ( p > 0.1). Microradiographs showed typical subsurface lesions in enamel next to control composite, but much less lesion around NACP nanocomposite. Enamel mineral loss Δ Z (mean ± sd; n = 25) around NACP nanocomposite was 13.8 ± 9.3 μm, much less than 33.5 ± 19.0 μm of the control ( p = 0.001).
Significance
Novel NACP nanocomposite substantially reduced caries formation in a human in situ model for the first time. Enamel mineral loss at the margins around NACP nanocomposite was less than half of the mineral loss around control composite. Therefore, the Ca and P ion-releasing NACP nanocomposite is promising for caries-inhibiting restorations.
1
Introduction
Dental composites consisting of fillers in a resin matrix are increasingly used because of their esthetics and enhanced performance . Extensive efforts have been performed to improve the resin compositions, filler reinforcement, and polymerization properties . However, secondary caries remains a main challenge facing composite restorations . Caries at the restoration margins is a frequent reason for replacement of existing restorations . The replacement of the failed restorations accounts for 50–70% of all restorations performed . Replacement dentistry costs $5 billion annually in the U.S. . To inhibit caries around the restorations, calcium phosphate (CaP)-resin composite materials were developed, which were shown to be effective in remineralizing tooth lesions in vitro . However, to date, the caries-inhibition efficacy of CaP composite in a human in situ model has not been reported.
Traditional CaP composites were developed by filling micrometer-sized CaP particles into dental resins . For example, amorphous calcium phosphate (ACP, Ca 3 [PO 4 ] 2 ) particles with a mean size of 55 μm were filled into resins . ACP is a precursor that forms initially and then transforms to apatite. Hydroxyapatite, Ca 10 (PO 4 ) 6 (OH) 2 , the prototype of minerals in teeth/bones, is the final stable product in the precipitation of calcium and phosphate ions in neutral and basic solutions . Traditional ACP composite released supersaturating levels of calcium phosphate ions and remineralized enamel lesions in vitro . However, traditional calcium phosphate composites were mechanically weak and not suitable for bulk restoratives . Recently, novel nanocomposites were developed with nanoparticles of ACP (NACP) having a mean particle size of 116 nm . The NACP nanocomposite released calcium and phosphate ions similar to traditional CaP composites, but with a 2-fold increase in flexural strength and elastic modulus for load-bearing restorations . The flexural strength and elastic modulus of the NACP nanocomposite matched those of a commercial composite control . Furthermore, NACP nanocomposite effectively neutralized a lactic acid challenge, while commercial control restoratives failed to neutralize the acid . However, the caries inhibition capability of the NACP nanocomposite in teeth has not been investigated.
Therefore, the objective of this study was to investigate the effects of NACP nanocomposite on caries inhibition in situ in the oral environment for the first time. A randomized split-mouth design was conducted with 25 volunteers wearing palatal devices for 14 days. After 14 days, the samples were removed, the biofilms were analyzed for Ca and P ion concentrations, and mineral content in enamel was measured via transverse microradiography. It was hypothesized that: (1) NACP nanocomposite will increase the Ca and P ion concentrations in biofilms in situ; (2) NACP nanocomposite will inhibit enamel caries at the enamel–composite interface to yield much less mineral loss, compared to control composite without NACP fillers.
2
Materials and methods
2.1
NACP nanocomposite fabrication
A spray-drying technique was developed to synthesize calcium phosphate and calcium fluoride nanoparticles . To make NACP, a solution was prepared using 1.5125 g of acetic acid (Baker, Phillipsburg, NJ), 0.8 g of calcium carbonate (Fisher, Fair Lawn, NJ) and 5.094 g of dicalcium phosphate-anhydrous with water. The calcium and phosphate concentrations in the solution were 8 and 5.333 mmol/L, respectively. The solution was sprayed through a nozzle into a heated chamber. The water and volatile acid were evaporated and the dried particles were collected by an electrostatic precipitator (AirQuality, Minneapolis, MN). This method yielded NACP with a mean particle size of 116 nm as measured in a previous study .
A monomer consisting of Bis-GMA (bisphenol-glycidyl dimethacrylate) and TEGDMA (triethylene glycol dimethacrylate) at 1:1 ratio (all by mass) was rendered light-curable with 0.2% camphorquinone and 0.8% ethyl 4-N,N-dimethylaminobenzoate. As reinforcement co-fillers, barium–boroaluminosilicate glass particles with a median diameter of 1.4 μm (Caulk/Dentsply, Milford, DE) were silanized with 4% 3-methacryloxypropyltrimethoxysilane and 2% n-propylamine . The fillers were mixed with the resin at a total filler mass fraction of 60% to form a cohesive paste. Two composites were fabricated with fillers of: (1) 40% NACP + 20% glass (NACP nanocomposite); and (2) 0% NACP + 60% glass (control composite).
2.2
Calcium (Ca) and phosphate (P) ion release measurement
Ca and P ion release from the NACP nanocomposite was measured. The composite paste was placed into rectangular molds of 2 mm × 2 mm × 12 mm following previous studies . The specimens were photo-cured (Triad 2000, Dentsply, York, PA) in air for 1 min on each side, and then incubated at 37 °C in a humidor for 1 day. A sodium chloride solution (133 mmol/L) was buffered to three pH values: pH 4 with 50 mmol/L lactic acid, pH 5.5 with 50 mmol/L acetic acid, and pH 7 with 50 mmol/L HEPES. Following previous studies , three specimens of approximately 2 mm × 2 mm × 12 mm were immersed in 50 mL of solution at each pH, yielding a specimen volume/solution of 2.9 mm 3 /mL. This compared to a specimen volume per solution of approximately 3.0 mm 3 /mL in a previous study . At 1, 2, 3, 5, 7, 10, 14, 21, and 28 days, aliquots of 0.5 mL were removed and replaced by fresh solution. Ca and P ion concentrations at each time period were measured via a spectrophotometric method (DMS-80 UV–visible, Varian, Palo Alto, CA) using known standards and calibration curves .
2.3
In situ tooth demineralization model
The in situ model of the present study was approved by the Research and Ethics Committee of the Federal University of Ceará in Brazil (Protocol #028/2011) and conformed to the Resolution 196/96 of the National Health Council concerning the Human Research Code of Ethics. This in situ model was similar to those reported in previous studies which successfully enabled the investigation of enamel and dentin caries formation . All participants signed written informed consent before being accepted into the study. Twenty-five volunteers were selected who fulfilled the following inclusion criteria: normal salivary flow rate, stimulated-saliva buffering capacity, good general and oral health with no active caries lesions or periodontal treatment needs, ability to comply with the experimental protocol, not having used antibiotics during the 3 months prior to the study, and not using fixed or removable orthodontic devices .
Freshly extracted bovine teeth were used following previous studies . One hundred bovine incisors were used to prepare one hundred enamel slabs (5 mm × 5 mm × 2 mm) using a diamond saw (Buehler, Lake Bluff, IL), as shown schematically in Fig. 1 A . Following a previous study , circular cavities with an approximate diameter of 2 mm and a depth of 1.5 mm were prepared ( Fig. 1 A). The 100 enamel slabs with cavities were randomly divided according to a computer-generated randomization list, into two groups of 50 slabs each: one group was filled with the NACP nanocomposite, and the other group was filled with the control composite. To focus on the effects of composite without interference from adhesive, no adhesive was used. Each composite paste was placed into the cavity and light-activated for 20 s using a light emitting diode Optilight LD Max (Gnatus, Ribeirão Preto, SP, Brazil) with an output of 600 mW/cm 2 . All the restored slabs were polished using aluminum oxide disc (Sof-lex disk system, M Dental, St. Paul, MN). As shown in Fig. 1 A, an enamel surface area of approximately 5 mm × 1.5 mm adjacent to the restoration was covered with an acid-resistant varnish to act as a control for mineral loss analysis. The filled enamel slabs were stored in a humidor at a relative humidity of 100% at 37 °C for 24 h and then used in the in situ model.
For 14 days, the 25 volunteers wore removable acrylic custom-made palatal devices, each containing four enamel slabs: two slabs were restored with the NACP nanocomposite on one side, and two slabs were restored with the control composite on the other side ( Fig. 1 B). Having slabs with two different treatments on the two opposite sides of the same device enabled them to experience the same oral environment for a fair comparison between the two materials. This method was supported by the absence of cross-contamination shown in previous studies . Each slab was covered with a plastic mesh with a 1 mm space, to allow biofilm accumulation and to protect the biofilms from mechanical disturbances . As a single-blind test, the volunteers did not know which was control composite or NACP nanocomposite. Seven days prior to the experiment and during the 14 days experiment, the volunteers brushed their teeth with non-fluoridated toothpaste. The purpose of this was to determine the effect of NACP nanocomposite on caries formation without interference from fluoridated toothpastes. In order to provide a cariogenic challenge, eight times daily at predetermined times, the volunteers removed the appliance, dripped one drop of a 20% sucrose solution on each slab, and then placed the appliance back into the mouth, following previous studies .
2.4
Biofilm analyses
On day 14, 12 h after the last application of the sucrose solution, the biofilm formed on each specimen was collected with sterilized plastic curettes. The biofilm was weighed in pre-weighed microcentrifuge tubes and agitated for 2 min in a Disrupter Genie Cell Disruptor (Precision Solutions, Rice Lake, WI). An aliquot of 50 μl of the sonicated suspension was diluted in 0.9% NaCl and serial decimal dilutions were inoculated in triplicate by the drop-counting technique in the following culture media : mitis salivarius agar containing 20% sucrose, to determine total streptococci; mitis salivarius agar plus 0.2 bacitracin/mL, to determine mutans streptococci; and Rogosa agar supplemented with 0.13% glacial acetic acid, to determine lactobacilli. The plates were incubated in 10% CO 2 at 37 °C for 48 h. The colony-forming units (CFU) were counted and the results were expressed as CFU/mg of dental biofilm with wet weight .
In addition, the Ca and P ion concentrations in the biofilms were also measured. The biofilms were treated with 0.5 M of HCl to extract the acid-soluble whole-biofilm Ca and P ions. “Phosphate (P) ions” and “inorganic phosphorus (Pi)” are interchangeable in the context of the present study. Samples were agitated at 30 rpm for 3 h and centrifuged. The supernatant was collected for Ca and P ion measurement via a spectrophotometric method .
2.5
Transverse microradiography (TMR) to measure mineral content
For TMR, specimen sections were cut to a thickness of approximately 200 μm, as shown schematically in Fig. 1 C. The sections were serially polished with water-cooled abrasive (320, 600, and 1200-grit Al 2 O 3 papers, Buehler) to a thickness of 110–130 μm, following a previous study . Contact microradiographs of enamel sections were produced on holographic film (Integraf, Kirkland, WA) exposed to 30-min Cu-Kα radiation (Faxitron-43855A, Hewlett Packard, McMinnville, OR). Mineral profiles were determined by quantitative analysis of microradiographs using NIS Elements-3.2 (Nikon, NY). Digital imaging captured the gray levels of a rectangular area (50 μm × 600 μm) of the radiographic image using an intensity resolution of 256 gray levels and a spatial resolution of 1.25 μm/pixel . Following previous studies , the mineral loss (Δ Z ) was obtained in the enamel region next to the enamel–composite, at four distances from the enamel–composite interface: 0–50, 50–100, 100–150, 150–200 μm, respectively. In addition, mineral loss Δ Z was also calculated for the enamel region of 0–250 μm as a whole . This was done by analyzing the grayscale intensity of the rectangular area of 250 μm × 600 μm of enamel next to the composite to obtain the average Δ Z for this entire region. Net Δ Z was obtained as Δ Z exposed − Δ Z varnish covered for the same enamel section, where Δ Z exposed was the enamel mineral loss on the side without varnish ( Fig. 1 C). As shown in Section 3 , Δ Z varnish covered was nearly 0, which indicates sound enamel without demineralization . The unit of the integrated mineral loss Δ Z is μm, because 100 μm vol% = 1 μm, following previous studies .
2.6
Statistical analysis
Sample size was determined based on previous data , where the required number of participants was determined to be 20, in anticipation of a dropout rate of 10%. This was based on an adequate power of 80% and a defined significance level of 5% ( p < 0.05). Accordingly, to ensure that this group size was available at the end of the study, 25 volunteers were recruited to compensate for possible dropouts (however, all 25 volunteers completed this study). One-way and two-way analyses-of-variance (ANOVA) were performed to detect the significant effects of the variables. Tukey’s multiple comparison procedures were performed at a p value of 0.05.
2
Materials and methods
2.1
NACP nanocomposite fabrication
A spray-drying technique was developed to synthesize calcium phosphate and calcium fluoride nanoparticles . To make NACP, a solution was prepared using 1.5125 g of acetic acid (Baker, Phillipsburg, NJ), 0.8 g of calcium carbonate (Fisher, Fair Lawn, NJ) and 5.094 g of dicalcium phosphate-anhydrous with water. The calcium and phosphate concentrations in the solution were 8 and 5.333 mmol/L, respectively. The solution was sprayed through a nozzle into a heated chamber. The water and volatile acid were evaporated and the dried particles were collected by an electrostatic precipitator (AirQuality, Minneapolis, MN). This method yielded NACP with a mean particle size of 116 nm as measured in a previous study .
A monomer consisting of Bis-GMA (bisphenol-glycidyl dimethacrylate) and TEGDMA (triethylene glycol dimethacrylate) at 1:1 ratio (all by mass) was rendered light-curable with 0.2% camphorquinone and 0.8% ethyl 4-N,N-dimethylaminobenzoate. As reinforcement co-fillers, barium–boroaluminosilicate glass particles with a median diameter of 1.4 μm (Caulk/Dentsply, Milford, DE) were silanized with 4% 3-methacryloxypropyltrimethoxysilane and 2% n-propylamine . The fillers were mixed with the resin at a total filler mass fraction of 60% to form a cohesive paste. Two composites were fabricated with fillers of: (1) 40% NACP + 20% glass (NACP nanocomposite); and (2) 0% NACP + 60% glass (control composite).
2.2
Calcium (Ca) and phosphate (P) ion release measurement
Ca and P ion release from the NACP nanocomposite was measured. The composite paste was placed into rectangular molds of 2 mm × 2 mm × 12 mm following previous studies . The specimens were photo-cured (Triad 2000, Dentsply, York, PA) in air for 1 min on each side, and then incubated at 37 °C in a humidor for 1 day. A sodium chloride solution (133 mmol/L) was buffered to three pH values: pH 4 with 50 mmol/L lactic acid, pH 5.5 with 50 mmol/L acetic acid, and pH 7 with 50 mmol/L HEPES. Following previous studies , three specimens of approximately 2 mm × 2 mm × 12 mm were immersed in 50 mL of solution at each pH, yielding a specimen volume/solution of 2.9 mm 3 /mL. This compared to a specimen volume per solution of approximately 3.0 mm 3 /mL in a previous study . At 1, 2, 3, 5, 7, 10, 14, 21, and 28 days, aliquots of 0.5 mL were removed and replaced by fresh solution. Ca and P ion concentrations at each time period were measured via a spectrophotometric method (DMS-80 UV–visible, Varian, Palo Alto, CA) using known standards and calibration curves .
2.3
In situ tooth demineralization model
The in situ model of the present study was approved by the Research and Ethics Committee of the Federal University of Ceará in Brazil (Protocol #028/2011) and conformed to the Resolution 196/96 of the National Health Council concerning the Human Research Code of Ethics. This in situ model was similar to those reported in previous studies which successfully enabled the investigation of enamel and dentin caries formation . All participants signed written informed consent before being accepted into the study. Twenty-five volunteers were selected who fulfilled the following inclusion criteria: normal salivary flow rate, stimulated-saliva buffering capacity, good general and oral health with no active caries lesions or periodontal treatment needs, ability to comply with the experimental protocol, not having used antibiotics during the 3 months prior to the study, and not using fixed or removable orthodontic devices .
Freshly extracted bovine teeth were used following previous studies . One hundred bovine incisors were used to prepare one hundred enamel slabs (5 mm × 5 mm × 2 mm) using a diamond saw (Buehler, Lake Bluff, IL), as shown schematically in Fig. 1 A . Following a previous study , circular cavities with an approximate diameter of 2 mm and a depth of 1.5 mm were prepared ( Fig. 1 A). The 100 enamel slabs with cavities were randomly divided according to a computer-generated randomization list, into two groups of 50 slabs each: one group was filled with the NACP nanocomposite, and the other group was filled with the control composite. To focus on the effects of composite without interference from adhesive, no adhesive was used. Each composite paste was placed into the cavity and light-activated for 20 s using a light emitting diode Optilight LD Max (Gnatus, Ribeirão Preto, SP, Brazil) with an output of 600 mW/cm 2 . All the restored slabs were polished using aluminum oxide disc (Sof-lex disk system, M Dental, St. Paul, MN). As shown in Fig. 1 A, an enamel surface area of approximately 5 mm × 1.5 mm adjacent to the restoration was covered with an acid-resistant varnish to act as a control for mineral loss analysis. The filled enamel slabs were stored in a humidor at a relative humidity of 100% at 37 °C for 24 h and then used in the in situ model.

VIDEdental - Online dental courses
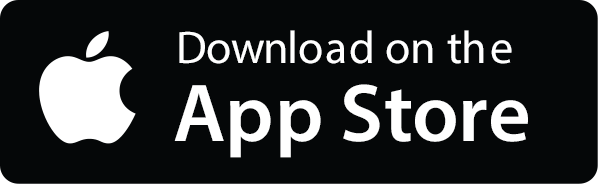
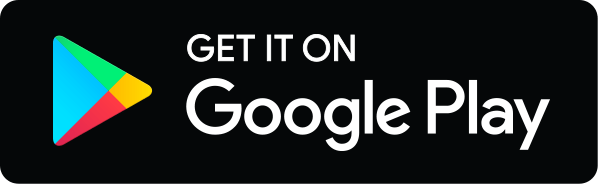