Abstract
Objectives
The occurrence of tooth root caries is increasing as the world population ages and tooth retention in seniors increases. Class V restorations with subgingival margins are difficult to clean and often lead to periodontitis. The objectives of this study were to develop a Class V composite containing dimethylaminohexadecyl methacrylate (DMAHDM) and nanoparticles of amorphous calcium phosphate (NACP), and investigate mechanical properties and the inhibition of six species of periodontitis-related biofilms for the first time.
Methods
Ethoxylated bisphenol A dimethacrylate (EBPADMA) and pyromellitic glycerol dimethacrylate (PMGDM) were mixed at 1:1 mass ratio to form the resin matrix. DMAHDM, NACP, and glass particles were incorporated at 3%, 20% and 50% by mass, respectively. Six species were tested: Porphyromonas gingivalis , Prevotella intermedia , Prevotella nigrescens , Aggregatibacter actinomycetemcomitans , Fusobacterium nucleatum and Enterococcus faecalis . Colony-forming units (CFU), live/dead assay, biomass via crystal violet staining, and polysaccharide production by biofilms were determined on composites.
Result
Adding 3% DMAHDM to composite did not affect the flexure strength and elastic modulus (p > 0.1). For all six species of periodontal pathogens, the DMAHDM composite had biofilm CFU nearly three orders of magnitude less than that without DMAHDM. The killing efficacy of DMAHDM composite against the six species was: E. faecalis < F. nucleatum < P. nigrescens = P. intermedia < A. actinomycetemcomitans < P. gingivalis . Biofilm biomass and polysaccharide were also greatly reduced via DMAHDM (p < 0.05).
Significance
The novel nanocomposite containing DMAHDM and NACP showed strong inhibiting effect against all six species of periodontitis-related pathogens. This composite is promising for Class V restorations to restore root caries and combat periodontitis.
1
Introduction
The world population is aging and seniors are retaining their teeth longer due to improved oral hygiene . However, increased retention of teeth means higher risks of developing tooth root caries because of gingival recession and reduced saliva flow in seniors . Periodontitis-related gingival recession often leads to more exposure of root surfaces to the oral environment which consequently results in the increasing risk of root caries. Root caries can be treated with a Class V restoration, whose margins are often subgingival, which can hinder cleaning and provide pockets for bacterial growth. This in turn would enhance the loss of the tooth’s clinical attachment and the development of periodontitis. It is widely accepted that microbial dental biofilms are the principal aetiological factor of periodontitis . Nevertheless, current resin-based restorations for treating root caries usually have no antibacterial function, and instead may even accumulate more biofilms/plaques in vivo , which could aggravate the progress of periodontitis.
Three main bacterial species that were most frequently detected in subgingival plaque from periodontitis and periimplantitis sites are Porphyromonas gingivalis ( P. gingivalis ), Prevotella intermedia ( P. intermedia ) and Aggregatibacter actinomycetemcomitans ( A. actinomycetemcomitans ) . They can produce virulence factors in periodontal pockets, thereby leading to progressive loss of the alveolar bone and periapical bone . Several studies have indicated that P. gingivalis may act as a keystone pathogen in periodontitis , which presents as a portion of the climax community in the biofilms at sites with progressing periodontitis. P. intermedia is positively associated with pregnancy gingivitis and periodontitis, because this species can use estrogen and progesterone as an essential source of nutrients instead of using vitamin K . Localized aggressive periodontitis is characterized by specific infections with A. actinomycetemcomitans . Besides these three species, a 4th species, Prevotella nigrescens ( P. nigrescens ) is biochemically similar to P. intermedia, and is associated with both healthy and diseased conditions of the periodontium . A 5th species, Fusobacterium nucleatum ( F. nucleatum ) is correlated with increased probing depth and progressive periodontal ligament reduction in periodontitis . F. nucleatum also enhances the invasion into human gingival epithelial and aortic endothelial cells by P. gingivalis . Further, a 6th species, Enterococcus faecalis ( E. faecalis ), while mostly regarded as an endodontic pathogen, is also detected in subgingival biofilms and saliva of subjects with chronic periodontal infections . To date, there has been no report on developing a dental composite for Class V restorations that can inhibit these six species related to the initiation and aggravation of periodontitis.
Composites are widely used in dental practice as filling materials due to their esthetics and direct-filling capabilities . Previous efforts have led to significant improvements in resin compositions, filler particles and polymerization efficiency . Nonetheless, composite restorations are challenged with accumulation of more biofilms/plaques . Plaques adjacent to the restoration margins can result in secondary caries. Therefore, efforts were made to develop antibacterial composites . Nanoparticles, such as silver, zinc oxide and polyethylenimine were incorporated into dental composites to decrease the cariogenicity of bacteria . Quaternary ammonium methacrylates (QAMs) were synthesized and incorporate into dental resins . QAMs with polymerizable groups can be immobilized in the polymer backbone to provide long-term antibacterial activity. QAMs are effective against a wide spectrum of microbes, such as Gram-positive and Gram-negative bacteria, fungi, and certain types of viruses, which is realized by positive-charged QAMs binding to the negative-charged bacterial membranes . Previous studies developed 12-methacryloyloxydodecylpyridinium bromide (MDPB) which was copolymerized in dental resins to reduce bacterial growth . Recently, resins containing a new antibacterial monomer dimethylaminohexadecyl methacrylate (DMAHDM) were shown to exhibit a strong antibacterial activity . These studies focused on the inhibition of cariogenic bacteria, without investigating Class V restorations to inhibit periodontitis-related pathogens.
The objectives of this study were to develop an antibacterial Class V composite for root caries restorations, and investigate the inhibition efficacy on biofilms of the six species of periodontitis-related pathogens for the first time. It was hypothesized that: (1) DMAHDM incorporation into the composite would not compromise the mechanical properties; (2) DMAHDM composite would possess potent antibiofilm activity against all six species of periodontal pathogens; (3) Different species of periodontal pathogens would show different killing efficacy via the DMAHDM composite.
2
Material and methods
2.1
Fabrication of DMAHDM-containing composites
A recent study showed that a resin of ethoxylated bisphenol A dimethacrylate (EBPADMA) and pyromellitic dianhydride glycerol dimethacrylate (PMDGDM) at 1:1 mass ratio, when filled with 20% of nanoparticles of amorphous calcium phosphate (NACP), had calcium and phosphate ion rechargeability . Therefore, the present study used the EBPADMA–PMDGDM resin (referred to as EBPM). EBPADMA was obtained from Sigma–Aldrich (St. Louis, MO). PMDGDM was obtained from Esstech (Essington, PA). The resin was rendered light-curable with 0.2% camphorquinone and 0.8% ethyl 4- N , N -dimethylaminobenzoate, following a previous study .
As previously described, antibacterial monomer DMAHDM was synthesized using a modified Menschutkin reaction, in which a tertiary amine group was reacted with an organohalide . A benefit of this reaction was that the reaction was done at virtually quantitative amounts and required minimal purification . DMAHDM was mixed with the photo-activated EBPM at a DMAHDM/(EBPM + DMAHDM) mass fraction of 10%. The 10% DMAHDM was used following a previous study . The resin was filled with NACP which had a mean particle size of 116 nm . The reason for including NACP was for calcium and phosphate ion release which could help remineralize tooth lesions . For mechanical reinforcement, barium boroaluminosilicate glass particles with a median size of 1.4 μm (Caulk/Dentsply, Milford, DE) were silanized with 4% 3-methacryloxypropyltrimethoxysilane and 2% n -propylamine, as previously described . The NACP and glass filler mass fractions were 20% and 50%, respectively. The total filler level of 70% yielded a cohesive composite paste.
Since the resin matrix mass fraction was 30%, the DMAHDM mass fraction in the composite was 3%. The composite with 0% DMAHDM served as a control. In addition, a commercial composite (Heliomolar, Ivoclar, Amherst, NY) also served as a comparative control. According to the manufacturer, Heliomolar contained 66.7% of nanofillers of 40–200 nm of silica and ytterbium-trifluoride with fluoride-release. Heliomolar is indicated for Class I–IV as well as Class V restorations. Therefore, three composites were tested:
- (1)
Commercial composite control (Heliomolar);
- (2)
Experimental composite control: 30% EBPM + 20% NACP + 50% glass particles (referred to as “EBPM + 0% DMAHDM”);
- (3)
Antibacterial composite: 27% EBPM + 3% DMAHDM + 20% NACP + 50% glass particles (referred to as “EBPM + 3% DMAHDM”).
2.2
Mechanical testing
A composite paste was placed into a rectangular mold of 2 × 2 × 25 mm which was covered by Mylar strips and clamped between two glass slides. Light-cure (Triad 2000, Dentsply, York, PA) was performed for 1 min on each open side of the mold. The cured specimens were stored at 37 °C in water for 24 h and then fractured in three-point flexure with a 10 mm span at a crosshead-speed of 1 mm/min on a computer-controlled Universal Testing Machine (5500R, MTS, Cary, NC) . Flexural strength was calculated as: S = 3P max /L(2bh 2 ), where P max is the fracture load, L is span, b is specimen width and h is thickness. Elastic modulus was calculated as: E = (P/d)(L 3 /[4bh 3 ]), where load P divided by displacement d is the slope in the linear elastic region. Six specimens were tested for each group (n = 6).
2.3
Bacteria culture
The use of the bacterial species was approved by the University of Maryland Baltimore Institutional Review Board. All six species were obtained from American Type Culture Collection (ATCC, Manassas, VA):
P. gingivalis ATCC33277;
P. intermedia ATCC 25611;
P. nigrescens ATCC 25261;
A. actinomycetemcomitans ATCC 43717;
F. nucleatum ATCC 25586;
E. faecalis ATCC 4083.
E. faecalis was grown in brain–heart infusion broth (BHI, Sigma) at 37 °C aerobically (95% air, 5% CO 2 ), following a previous study . For the other five species, each was grown in a tryptic soy broth (TSB, Sigma) supplemented with yeast extract (5 g/L), l -cysteine hydrochloride (0.5 g/L), hemin(5 mg/L) and menadione (1 mg/L) at 37 °C anaerobically (90% N 2 , 5% CO 2 , 5% H 2 ), following a previous study . Then for each species, the inoculum was adjusted to 10 8 colony-forming unit counts CFU/mL, based on the standard curve of OD 600nm versus CFU/mL for each species. The inoculum was then diluted in growth medium at a ratio of 1:10 and used for subsequent experiments.
2.4
Single-species biofilm formation on composite
For each composite, disk specimens were made using molds with a diameter of 9 mm and thickness of 2 mm, and the composite was light-cured for 1 min on each open side between transparent Mylar strips . The cured disks were immersed in 200 mL of distilled water and magnetically-stirred with a bar at a speed of 100 rpm for 1 h to remove any uncured monomers, following a previous study . The disks were then sterilized with ethylene oxide (AnproleneAN 74i, Andersen, Haw River, NC) and de-gassed for 7 days.
Saliva was collected from fifteen healthy adult donors having natural dentition without active caries or periopathology, and without the use of antibiotics within the last 3 months. The donors did not brush teeth for 24 h and abstained from food and drink intake for 2 h prior to donating saliva. Stimulated saliva was collected during parafilm chewing and was kept on ice. An equal volume of saliva from each of the fifteen donors was combined to form the saliva sample. The saliva was centrifuged at 3000 rpm for 20 min to remove cellular debris. The supernatant was collected and filter-sterilized through disposable, sterile 0.22 μm filters (VWR International, Radnor, PA). The sterile saliva was used to coat a salivary pellicle on the composite disks by immersing each disk in saliva in a 24-well plate for 2 h at 37 °C .
Each bacteria species was used individually to form single-species biofilms following previous studies . The salivary pellicle-coated composite disks were transferred to a new 24-well plate, and each bacterial species was inoculated at a concentration of 10 7 CFU/mL in 1.5 mL medium in each well. After 24 h, the disks with adherent biofilms were transferred to new 24-well plates filled with fresh medium and incubated for 24 h. This totaled 2 days of culture for each species to form biofilm, following previous studies .
Six composite disks were tested for each composite with each bacterial species for each of the following biological tests, except for live/dead bacteria assay. For live/dead assay, three composite disks were tested for each composite with each bacterial species, using a total of 54 composite disks. Five random images were chosen from each disk, yielding 15 images for each composite with each bacterial species.
2.5
Live/dead bacteria assay
For live/dead staining assay, each composite disk with 2-day biofilm was washed three time with cysteine peptone water (CPW) to remove the non-adherent bacteria. The Live/Dead Baclight bacterial viability kits (Molecular Probes, Eugene, OR) were used to stain the bacteria, following the manufacturer’s instructions. A mixture of 2.5 μM SYTO 9 and 2.5 μM propidium iodide was used to stain each sample for 15 min in the dark room. The live bacteria were stained with SYTO 9 to emit a green fluorescence. Bacteria with compromised membranes were stained with propidium iodide to emit a red fluorescence. The biofilms were imaged with an inverted epifluorescence microscope (TE2000-S, Nikon, Melville, NY).
2.6
Colony-forming unit (CFU) counts
A 3 × 6 full-factorial design was used with three composites and six bacterial species. Biofilms were formed by culturing for 2 days as described above. Disks with 2-day biofilms were transferred into tubes with 2 mL CPW, and the biofilms were harvested by scraping by sterilized blades and sonication/vortexing (Fisher, Pittsburg, PA). For E. faecalis , the BHI agar was used to measure the CFU counts. For the other five species, tryptic soy blood agar culture plates with 5 g/L yeast extract, 0.5 g/L l -cysteine hydrochloride, 5 mg/L hemin, 1 mg/L menadione and 5% sheep blood were used. The biofilm suspensions were serially diluted, spread onto the agar plate and incubated at 37 °C aerobically for E. faecalis , and anaerobically for the other five species, for 48 h. Then, the number of growing colonies was counted by a colony counter (Reichert, NY) and used, along with the dilution factor, to calculate the CFU counts.
2.7
Crystal violet biofilm biomass assay
The biofilm biomass was evaluated by a modified method based on staining the biofilms with crystal violet (CV). Composite disks were prepared and 2-day biofilms were formed. Then, the disks were rinsed with CPW, air-dried for 20 min, fixed in 200 mL of formalin for 15 min, and stained with 0.1% crystal violet (Sigma–Aldrich, St. Louis, MO) for 15 min at room temperature. The excess of unbound dye was removed by washing the disk with PBS. The bound CV was de-stained with 95% ethanol. The extracted solution was transfer into a 96-well plate. The biofilm biomass was evaluated by the absorbance at optical density 600 nm (OD 600 nm ) using a microplate reader (SpectraMax M5, Molecular Devices, Sunnyvale, CA). CV is a basic dye, which binds to negatively charged surface molecules and polysaccharides in the extracellular matrix. The purple color could be eluted by ethanol measured at a wavelength of 600 nm . The background staining was calibrated by subtracting the mean value for CV bound to the sterilized disks without bacteria .
2.8
Polysaccharide measurement
The water-insoluble polysaccharide in the extracellular polymeric substance (EPS) of the biofilm was determined by a phenol-sulfuric acid method . The disk with 2-day biofilm was immersed in a vial with 2 mL CPW, and the biofilm was collected by sonication/votexing. Centrifugation yielded a precipitate, which was rinsed twice with PBS and resuspended in 1 mL of de-ionized water. Subsequently, 1 mL of a 6% phenol solution was added to the vial, followed by 5 mL of 95–97% sulfuric acid . The vial was incubated at room temperature for 30 min. Then, 100 μL of the solution was transferred into a 96-well plate. The amount of polysaccharide in the biofilm was determined by measuring the absorbance at 490 nm with the microplate reader (SpectraMax M5). Five different glucose concentrations of 0, 5, 10, 20, 50 and 100 mg/L were used as standard in the conversion of OD readings to polysaccharide concentrations .
2.9
Statistical analysis
All data were checked for normal distribution with the Kolmogorov–Smirnov test. Testing for significant differences was performed by one-way and two-way analyses of variance (ANOVA) using Tukey’s post-hoc test for pairwise comparisons. Statistical analyses were performed by SPSS 19.0 software (SPSS, Chicago, IL) at a pre-set alpha of 0.05.
2
Material and methods
2.1
Fabrication of DMAHDM-containing composites
A recent study showed that a resin of ethoxylated bisphenol A dimethacrylate (EBPADMA) and pyromellitic dianhydride glycerol dimethacrylate (PMDGDM) at 1:1 mass ratio, when filled with 20% of nanoparticles of amorphous calcium phosphate (NACP), had calcium and phosphate ion rechargeability . Therefore, the present study used the EBPADMA–PMDGDM resin (referred to as EBPM). EBPADMA was obtained from Sigma–Aldrich (St. Louis, MO). PMDGDM was obtained from Esstech (Essington, PA). The resin was rendered light-curable with 0.2% camphorquinone and 0.8% ethyl 4- N , N -dimethylaminobenzoate, following a previous study .
As previously described, antibacterial monomer DMAHDM was synthesized using a modified Menschutkin reaction, in which a tertiary amine group was reacted with an organohalide . A benefit of this reaction was that the reaction was done at virtually quantitative amounts and required minimal purification . DMAHDM was mixed with the photo-activated EBPM at a DMAHDM/(EBPM + DMAHDM) mass fraction of 10%. The 10% DMAHDM was used following a previous study . The resin was filled with NACP which had a mean particle size of 116 nm . The reason for including NACP was for calcium and phosphate ion release which could help remineralize tooth lesions . For mechanical reinforcement, barium boroaluminosilicate glass particles with a median size of 1.4 μm (Caulk/Dentsply, Milford, DE) were silanized with 4% 3-methacryloxypropyltrimethoxysilane and 2% n -propylamine, as previously described . The NACP and glass filler mass fractions were 20% and 50%, respectively. The total filler level of 70% yielded a cohesive composite paste.
Since the resin matrix mass fraction was 30%, the DMAHDM mass fraction in the composite was 3%. The composite with 0% DMAHDM served as a control. In addition, a commercial composite (Heliomolar, Ivoclar, Amherst, NY) also served as a comparative control. According to the manufacturer, Heliomolar contained 66.7% of nanofillers of 40–200 nm of silica and ytterbium-trifluoride with fluoride-release. Heliomolar is indicated for Class I–IV as well as Class V restorations. Therefore, three composites were tested:
- (1)
Commercial composite control (Heliomolar);
- (2)
Experimental composite control: 30% EBPM + 20% NACP + 50% glass particles (referred to as “EBPM + 0% DMAHDM”);
- (3)
Antibacterial composite: 27% EBPM + 3% DMAHDM + 20% NACP + 50% glass particles (referred to as “EBPM + 3% DMAHDM”).
2.2
Mechanical testing
A composite paste was placed into a rectangular mold of 2 × 2 × 25 mm which was covered by Mylar strips and clamped between two glass slides. Light-cure (Triad 2000, Dentsply, York, PA) was performed for 1 min on each open side of the mold. The cured specimens were stored at 37 °C in water for 24 h and then fractured in three-point flexure with a 10 mm span at a crosshead-speed of 1 mm/min on a computer-controlled Universal Testing Machine (5500R, MTS, Cary, NC) . Flexural strength was calculated as: S = 3P max /L(2bh 2 ), where P max is the fracture load, L is span, b is specimen width and h is thickness. Elastic modulus was calculated as: E = (P/d)(L 3 /[4bh 3 ]), where load P divided by displacement d is the slope in the linear elastic region. Six specimens were tested for each group (n = 6).
2.3
Bacteria culture
The use of the bacterial species was approved by the University of Maryland Baltimore Institutional Review Board. All six species were obtained from American Type Culture Collection (ATCC, Manassas, VA):
P. gingivalis ATCC33277;
P. intermedia ATCC 25611;
P. nigrescens ATCC 25261;
A. actinomycetemcomitans ATCC 43717;
F. nucleatum ATCC 25586;
E. faecalis ATCC 4083.
E. faecalis was grown in brain–heart infusion broth (BHI, Sigma) at 37 °C aerobically (95% air, 5% CO 2 ), following a previous study . For the other five species, each was grown in a tryptic soy broth (TSB, Sigma) supplemented with yeast extract (5 g/L), l -cysteine hydrochloride (0.5 g/L), hemin(5 mg/L) and menadione (1 mg/L) at 37 °C anaerobically (90% N 2 , 5% CO 2 , 5% H 2 ), following a previous study . Then for each species, the inoculum was adjusted to 10 8 colony-forming unit counts CFU/mL, based on the standard curve of OD 600nm versus CFU/mL for each species. The inoculum was then diluted in growth medium at a ratio of 1:10 and used for subsequent experiments.
2.4
Single-species biofilm formation on composite
For each composite, disk specimens were made using molds with a diameter of 9 mm and thickness of 2 mm, and the composite was light-cured for 1 min on each open side between transparent Mylar strips . The cured disks were immersed in 200 mL of distilled water and magnetically-stirred with a bar at a speed of 100 rpm for 1 h to remove any uncured monomers, following a previous study . The disks were then sterilized with ethylene oxide (AnproleneAN 74i, Andersen, Haw River, NC) and de-gassed for 7 days.
Saliva was collected from fifteen healthy adult donors having natural dentition without active caries or periopathology, and without the use of antibiotics within the last 3 months. The donors did not brush teeth for 24 h and abstained from food and drink intake for 2 h prior to donating saliva. Stimulated saliva was collected during parafilm chewing and was kept on ice. An equal volume of saliva from each of the fifteen donors was combined to form the saliva sample. The saliva was centrifuged at 3000 rpm for 20 min to remove cellular debris. The supernatant was collected and filter-sterilized through disposable, sterile 0.22 μm filters (VWR International, Radnor, PA). The sterile saliva was used to coat a salivary pellicle on the composite disks by immersing each disk in saliva in a 24-well plate for 2 h at 37 °C .
Each bacteria species was used individually to form single-species biofilms following previous studies . The salivary pellicle-coated composite disks were transferred to a new 24-well plate, and each bacterial species was inoculated at a concentration of 10 7 CFU/mL in 1.5 mL medium in each well. After 24 h, the disks with adherent biofilms were transferred to new 24-well plates filled with fresh medium and incubated for 24 h. This totaled 2 days of culture for each species to form biofilm, following previous studies .
Six composite disks were tested for each composite with each bacterial species for each of the following biological tests, except for live/dead bacteria assay. For live/dead assay, three composite disks were tested for each composite with each bacterial species, using a total of 54 composite disks. Five random images were chosen from each disk, yielding 15 images for each composite with each bacterial species.
2.5
Live/dead bacteria assay
For live/dead staining assay, each composite disk with 2-day biofilm was washed three time with cysteine peptone water (CPW) to remove the non-adherent bacteria. The Live/Dead Baclight bacterial viability kits (Molecular Probes, Eugene, OR) were used to stain the bacteria, following the manufacturer’s instructions. A mixture of 2.5 μM SYTO 9 and 2.5 μM propidium iodide was used to stain each sample for 15 min in the dark room. The live bacteria were stained with SYTO 9 to emit a green fluorescence. Bacteria with compromised membranes were stained with propidium iodide to emit a red fluorescence. The biofilms were imaged with an inverted epifluorescence microscope (TE2000-S, Nikon, Melville, NY).
2.6
Colony-forming unit (CFU) counts
A 3 × 6 full-factorial design was used with three composites and six bacterial species. Biofilms were formed by culturing for 2 days as described above. Disks with 2-day biofilms were transferred into tubes with 2 mL CPW, and the biofilms were harvested by scraping by sterilized blades and sonication/vortexing (Fisher, Pittsburg, PA). For E. faecalis , the BHI agar was used to measure the CFU counts. For the other five species, tryptic soy blood agar culture plates with 5 g/L yeast extract, 0.5 g/L l -cysteine hydrochloride, 5 mg/L hemin, 1 mg/L menadione and 5% sheep blood were used. The biofilm suspensions were serially diluted, spread onto the agar plate and incubated at 37 °C aerobically for E. faecalis , and anaerobically for the other five species, for 48 h. Then, the number of growing colonies was counted by a colony counter (Reichert, NY) and used, along with the dilution factor, to calculate the CFU counts.
2.7
Crystal violet biofilm biomass assay
The biofilm biomass was evaluated by a modified method based on staining the biofilms with crystal violet (CV). Composite disks were prepared and 2-day biofilms were formed. Then, the disks were rinsed with CPW, air-dried for 20 min, fixed in 200 mL of formalin for 15 min, and stained with 0.1% crystal violet (Sigma–Aldrich, St. Louis, MO) for 15 min at room temperature. The excess of unbound dye was removed by washing the disk with PBS. The bound CV was de-stained with 95% ethanol. The extracted solution was transfer into a 96-well plate. The biofilm biomass was evaluated by the absorbance at optical density 600 nm (OD 600 nm ) using a microplate reader (SpectraMax M5, Molecular Devices, Sunnyvale, CA). CV is a basic dye, which binds to negatively charged surface molecules and polysaccharides in the extracellular matrix. The purple color could be eluted by ethanol measured at a wavelength of 600 nm . The background staining was calibrated by subtracting the mean value for CV bound to the sterilized disks without bacteria .
2.8
Polysaccharide measurement
The water-insoluble polysaccharide in the extracellular polymeric substance (EPS) of the biofilm was determined by a phenol-sulfuric acid method . The disk with 2-day biofilm was immersed in a vial with 2 mL CPW, and the biofilm was collected by sonication/votexing. Centrifugation yielded a precipitate, which was rinsed twice with PBS and resuspended in 1 mL of de-ionized water. Subsequently, 1 mL of a 6% phenol solution was added to the vial, followed by 5 mL of 95–97% sulfuric acid . The vial was incubated at room temperature for 30 min. Then, 100 μL of the solution was transferred into a 96-well plate. The amount of polysaccharide in the biofilm was determined by measuring the absorbance at 490 nm with the microplate reader (SpectraMax M5). Five different glucose concentrations of 0, 5, 10, 20, 50 and 100 mg/L were used as standard in the conversion of OD readings to polysaccharide concentrations .
2.9
Statistical analysis
All data were checked for normal distribution with the Kolmogorov–Smirnov test. Testing for significant differences was performed by one-way and two-way analyses of variance (ANOVA) using Tukey’s post-hoc test for pairwise comparisons. Statistical analyses were performed by SPSS 19.0 software (SPSS, Chicago, IL) at a pre-set alpha of 0.05.
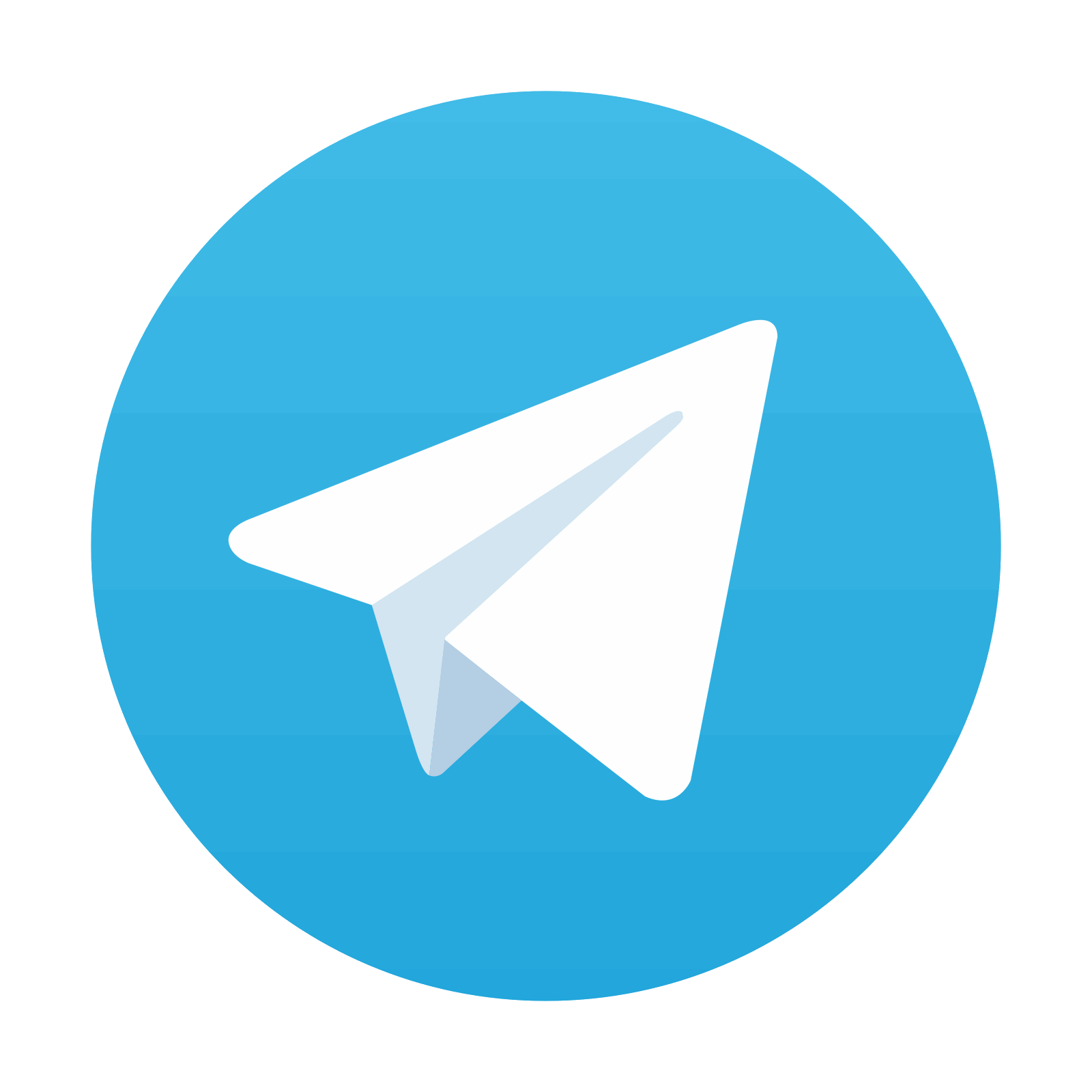
Stay updated, free dental videos. Join our Telegram channel

VIDEdental - Online dental courses
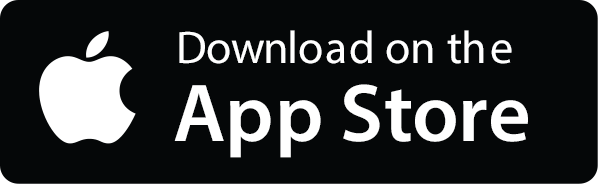
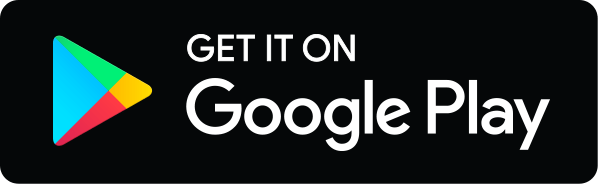