Graphical abstract

Highlights
- •
Initial bacterial adhesion to the Ti and Ti alloy was significantly inhibited after plasma functionalizing.
- •
The bacterial adhesion-resistance effect was induced by carbon cleaning.
- •
The initial cell adhesion and ALP activity at 14 days were enhanced in plasma-functionalized specimens.
Abstract
Objective
Even though roughened titanium (Ti) and Ti alloys have been clinically used as dental implant, they encourage bacterial adhesion, leading to failure of the initial stability. Here, the non-thermal atmospheric pressure plasma jet (NTAPPJ) functionalized Ti and Ti alloy were investigated to promote cellular activities but inhibit the initial attachment of the adherent pioneer bacterium, Streptococcus sanguinis, without topographical changes.
Methods
After the produced radicals from NTAPPJ were characterized, bacterial adhesion to specimens was assessed by PrestoBlue assay and live-dead staining with or without the NTAPPJ functionalizing. After the surface was characterized using optical profilometry, X-ray photoelectron spectroscopy and contact angle analysis, the ions released from the specimens were investigated. In vitro initial cell attachment (4 h or 24 h) with adhesion images and alkaline phosphatase activity (ALP, 14 days) measurements were performed using rat bone marrow-derived mesenchymal stem cells.
Results
The initial bacterial adhesion to the Ti and Ti alloy was significantly inhibited after NTAPPJ functionalizing (p < 0.05) compared to those without NTAPPJ functionalizing. The bacterial adhesion-resistance effect was induced by carbon cleaning, which was dependent on the working gas used on the Ti specimens (nitrogen > ammonia and air, p < 0.05). The initial cell adhesion with well-developed vinculin localization and consequent ALP activity at 14 days to the NTAPPJ-functionalized specimens were superior to the non-treated specimens.
Significance
For the promising success of dental implants, NTAPPJ functionalizing is suggested as a novel surface modification technique; this technique can help ensure the success of integration between the dental implants and bone tissues with less concern of inflammation.
1
Introduction
Dental implants using titanium (Ti) and Ti alloys are widely used for restoring missing tooth by anchoring in bone tissue via osseointegration and connecting prosthodontics for tooth rehabilitation . However, the initial success of dental implants is still far from ideal in patient populations, and further investigation is needed to reduce patient pain. Major attempts to improve the performance of the implants have been made by targeting the surface characteristics . Because the surface features of the implants strongly affect their biofunctionality and initial stability, a variety of surface modification techniques have been developed . Among these, modifications of the roughness, wettability, and chemistry of the surface have greatly impacted the biological interactions between the implants and tissue milieu .
The modification of the surface roughness affects the biological response to the tissue and cells; nano- or micro-rough implant surface have been shown to be much better than smooth counterpart . However, failure in dental implant has been reported due to bacterial adhesion to the surface of the rough implants . Additionally, bacterial adhesion and accumulation on the implant may often accompany failure of bone tissue regeneration, including peri-implantitis .
In many cases, the surface chemical composition that was favorable to the neighboring tissue or cells enhanced surface reactivity and biological performance . In addition, an increase in the surface energy was known to promote the biological response to the implant . Although little information has been reported about how wettability relates to the surface energy of Ti dental implants to affect the tissue, a number of studies have found that the hydrophilic surface of the Ti dental implant improved initial cell adhesion, growth, and differentiation compared to a hydrophobic surface .
To inhibit bacterial adhesion and to induce hydrophilicity from the increased surface energy of the Ti implant without a surface roughness change, a non-thermal atmospheric pressure plasma modification has been introduced . A non-thermal atmospheric pressure plasma jet (NTAPPJ), which can produce reactive radicals in the surgical performance site, can induce hydrophilicity on Ti and Ti alloys without topographical changes; additional advantages include portability, short treatment times, and customizability of radical production compared to other topography-preserved surface modification methods, such as ultraviolet and low level laser therapy . The potentials of NTAPPJ in dental application for bacterial adhesion-resistance, rapid osseointegration, early formation of peri-implant soft tissue seals and sterilization have been investigated . However, no study to date has addressed the effects of NTAPPJ functionalizing on roughened Ti and Ti alloys in terms of bacterial resistance or initial cell adhesion with same functionalizing conditions.
Here, we investigated the in vitro bacterial resistance to the adherent pioneer bacterium , Streptococcus sanguinis, and the initial cell adhesion using bone marrow derived mesenchymal stem cells on NTAPPJ-modified Ti and Ti alloy surfaces in dental applications. The null hypothesis was that there would be no significant difference in bacterial resistance or cell adhesion between the NTAPPJ-modified and original surfaces of the Ti and Ti alloy.
2
Materials and methods
2.1
Non-thermal atmospheric pressure plasma jet functionalizing
The NTAPPJ ( Fig. 1 ) was designed and provided by the Plasma Bioscience Research Center (Kwangwoon University Seoul, Korea). Details of the NTAPPJ are described elsewhere and in the supplement . Briefly, the modulated NTAPPJ equipment comprises an inner electrode of tungsten (1.2 mm depth and 0.2 mm thickness) with a 3.2 mm depth of quartz as a dielectric. The hole in the stainless steel outer electrode was 0.7 mm with a 2 mm height of porous alumina having a 150–200 μm pore size with 35% porosity. A high-voltage power supply and Neon Trans (PNP-1000, maximum output voltage 15 kV, current 13 mA, 60 Hz in AC power) were attached to input or control the power, and a mass flow controller (AFC600, Astrovac, Korea) was installed to change the type and flow rate of the gases ( Fig. 1 ). The plasma was formed by passing 1000 standard cubic centimeters per minute (sccm) of nitrogen (99.9%) or compressed air through the plasma-jet device. To produce the ammonia plasma, vaporized ammonia gas was generated by passing 100 sccm of nitrogen through a 28% ammonium hydroxide solution (Sigma Aldrich, USA) with 900 sccm of nitrogen. In total, 1000 sccm of nitrogen-containing ammonia gas passed through the plasma-jet device to treat one specimen. The NTAPPJ functionalizing was applied to the roughened (Sa = 0.5 ± 0.05 μm) Ti and Ti–Ag disk specimens ( ϕ = 12 mm, d = 1 mm) for 10 s with a 3-mm distance between the jet tip and the surface of the specimen. Previously, the effective distance from the center of the NTAPPJ-treated spot was calculated to be ∼15 mm using a polystyrene dish, however, the NTAPPJ effect diminished according to an increase in the distance from the center spot . To obtain uniform NTAPPJ functionalization on the substrate, the disk specimen was manually moved horizontally during treatment time (5 s/cycle). The control samples were not exposed to NTAPPJ because there was no difference in the surface characteristics of the samples with gas only or plasma treatment . To avoid aging effects, which result in a decrease in functionalization according to an increase in incubation time in atmospheric pressure following NTAPPJ treatment , the NTAPPJ-treated substrate was immediately used for further studies. The optical emission spectrum was measured to identify the excited plasma species produced from NTAPPJ using the protocols outlined .
2.2
Specimens
To assess the effects of the NTAPPJ functionalizing on the Ti and Ti–Ag dental implants, Ti and Ti–Ag disks ( ϕ = 12 mm, d = 1 mm) were used as models of dental implant. These disks were prepared as previously described . The Ti disks were kindly provided by Osstem Implant (Busan, Korea). A Ti–Ag alloy, including 2% Ag in Ti, was chosen based on previous results due to its high corrosion resistance and mechanical properties among various Ti–Ag alloys . To generate a rough surface, the specimens were polished using 200-grit SiC sandpaper. After confirming that the surface roughness (Sa) value was within the range of 0.5 ± 0.05 μm, the specimen was used for further study. The specimens were decontaminated and sterilized as previously described . In brief, the specimens were ultrasonically rinsed using a series of acetone, ethanol and distilled water for 10 min each. After drying under a vacuum at 25 °C for 1 day, the specimens were sterilized using ethylene oxide gas and exposed to air for 48 h to eliminate any remaining ethylene oxide gas.
2.3
Surface analysis
A surface analysis was performed before and after the NTAPPJ functionalizing using optical surface profilometry, X-ray photoelectron spectroscopy and contact angle analysis. To visualize the difference in roughness before and after the 10-s NTAPPJ functionalizing, a roughness analysis was performed using an optical surface profilometer (Contour GT-X3 base, Bruker, Germany). Roughness data (Ra, Sa) were obtained as 2D and 3D roughness parameters, respectively. The vertical resolution was no more than 0.1 nm, according to the manufacturer. The optical profilometer optically measured the surface height and represented it in grayscale. The grayscale represents the surface height of a spot. Therefore, the optical profilometer facilitated the evaluation of the differences in the surface roughness at the same position before and after functionalizing. The objective magnification and zoom of the standard camera were ×10 and ×2.0, respectively. The visualized area was 0.23 × 0.31 mm 2 . The scan length and backscan of each analysis were 30 μm. The average (n = 5) and standard deviation (SD) were shown. A contact angle analysis was performed using a Phoenix 300 (SEO, Suwon-si, Gyeonggi-do, Korea). Five microliters of distilled water and ethylene glycol (Sigma Aldrich) were pipetted onto the center of the NTAPPJ-treated and non-treated specimens. After 5 s, the static contact angle was measured, and the average of the right and left values was reported. Five samples were measured from each group. The surface energy was calculated according to the Owens–Wendt method, which uses non-polar (dispersive) and polar components . The room’s temperature was set at 20 °C. The surface energy with non-polar (tension and dispersive) and polar parameters of the test liquids were as follows: surface tension and dispersive and polar forces, respectively, were set at 72.8, 21.8, and 51.0 mJ/m 2 for water and at 48.3, 29.3, and 19.0 mJ/m 2 for ethylene glycol. The X-ray photoelectron spectra (XPS, K-alpha, Thermo Scientific, UK) were obtained using monochromated Al K-alpha. C1s at 284.8 eV was used as a reference, and Ti, C, O, N, and Ag were detected in detail. The surface analysis was independently performed in triplicate, and the representative data are shown.
2.4
Bacterial adhesion assay
S. sanguinis (ATCC 10556, USA) was chosen because, as a pioneer bacterium, it is responsible for the early stages of biofilm formation on dental implants. S. sanguinis was grown in trypticase soy agar (KisanBio, Seoul, Korea) with 5% defibrinated sheep blood (KisanBio). After picking one colony from an agar plate, that colony was suspended in brain heart infusion broth (BHI, KisanBio) and incubated at 37 °C. After 6 h of incubation, when bacteria are in the log phase of growth, the bacterial suspension was centrifuged for 5 min at 5000 × g . The pellets were re-suspended in fresh BHI solution to 5 × 10 7 CFU/ml. The bacteria suspension (1 ml) was pipetted into each well of a 12-well plate; each well contained a single disk specimen. Each specimen was fully immersed in the bacterial suspension and incubated for 2 h at 37 °C (n = 5). To assess the effect of the ions on bacterial attachment, 50 ppb of TiCl 4 or 20 ppb of AgNO 3 were added together or separately. Each disc was washed twice with PBS (Gibco, USA) and transferred to a fresh 12-well culture dish. After the addition of 1 ml of fresh BHI solution with 100 μl of PrestoBlue (Molecular probes, Eugene, OR, USA) to each well, the culture dish was incubated for 1 h to investigate the adherent bacteria numbers. Traditional colony counting methods can cause damage and loss of bacteria during the detachment step from the specimen before spreading the bacteria on an agar plate; to avoid the damage and loss of bacteria, the bacterial cell counting method using PrestoBlue was chosen. The liquid (100 μl) was transferred to a 96-well plate, and the absorbance was read at 570 and 600 nm using a microplate reader (Epoch, BioTek, USA). The absorbance that normalized the 570 nm values to the 600 nm values for each well was used to count the attached bacteria. To confirm the correlation between the normalized absorbance from the PrestoBlue assay and the bacterial numbers, the serial colony-forming unit (CFU) method was used. In short, serial dilutions were generated using BHI, and a 0.1 ml aliquot from each dilution was plated on BHI agar plates (Bacto Agar, BD) and incubated at 37 °C for 24 h. To count the bacteria using the PrestoBlue assay, 10 μl of the chemical was added to each serially diluted bacterial suspension, and a calibration curve was generated. The assays were independently performed in triplicate. The representative means (n = 5) and SD were recorded.
2.5
Confocal laser microscopy
Live-dead staining was performed using SYTO ® 9 and propidium iodide (Thermo Fisher Scientific, Carlsbad, CA, USA) according to the manufacturer’s instructions. Staining solutions were added to each well for observation under a confocal laser microscope (LSM700, Carl Zeiss, Thornwood, NY, USA) with 40× magnification (4× objective × 10× eyepiece). Intense green fluorescence (ex/em = 480/500) was observed from the live cells, and bright red fluorescence (ex/em = 490/635) was observed from the dead cells. The tests were independently performed in triplicate, and the representative images are shown.
2.6
ICP-MS
Inductively coupled plasma mass spectrometry (ICP-MS, ELAN 6100, PerkinElmer, Waltham, MA, USA) was used to determine the ion concentrations (Ti and Ag). Following the incubation of 1 ml of BHI with the NTAPPJ treated or non-treated specimens in a 12-well plate for 2 h at 37 °C, the conditioned solutions were used for measurement.
2.7
Initial cell adhesion test
Rat bone marrow-derived mesenchymal stem cells (rBMMSCs) were taken from the femur and tibia of 5-week-old male Sprague-Dawley rats and cultured as previously described . Briefly, after the femur and tibia were dissected free of the soft tissue, they were placed in α-MEM (Welgene); the sectioned bone and flushed bone marrow were aseptically incubated with a collagenase and dispase solution for 30 min. After centrifugation at 1500 rpm, the cell pellets were cultured in α-MEM supplemented with 10% fetal bovine serum, containing 0.5% antibiotic/antimycotic at 37 °C in an atmosphere of 5% CO 2 . After 5 days of culture, the non-adherent cells were removed. rBMMSCs between passages 2 and 5 were used for this experiment. All animal protocols were conducted according to the standards and regulations of the Institutional Review Board, Dankook University (DKU-11-028). For the initial cell adhesion assay, 1 × 10 5 cells/100 μl were seeded onto the specimen and incubated for 4 or 24 h in a humidifying incubator with 5% CO 2 at 37 °C. After washing three times, the adherent cell numbers were indirectly measured using the cell viability of the adherent cells as measured using the water-soluble tetrazolium salt (WST) assay; the plate reader was used at an absorbance of 450 nm (SpectraMax M2e, Molecular Devices, Sunnyvale, CA, USA) after the incubation with 10% WST salt (EA-Cytox, Daeil Lab, Seoul, Korea) for 1 h. A calibration curve between the number of cells and the absorbance at 450 nm of the WST solution was plotted, and the correlation coefficient was calculated to be 0.995, indicating a strong linear response between the cell number and the absorbance at 450 nm. Confocal microscopy was used to detect the vinculin and actin filaments at 200× magnification (20× objective × 10× eyepiece) after 1 × 10 5 cells/100 μl were seeded on the specimen for 4 h and stained with the actin cytoskeleton and focal adhesion staining kit (Millipore, Bedford, MA) according to the manufacturer’s protocol . The experiments were performed in triplicate, and the representative images are shown.
2.8
Alkaline phosphatase activity
After 1 × 10 5 cells/100 μl of rBMMSCs were seeded onto the specimens for 4 h and washed with PBS, the specimens were incubated in a humidifying incubator at 5% CO 2 and 37 °C for 14 days with or without osteogenic supplement medium (OS) containing 50 μg/ml l -ascorbic acid and 10 mM β-glycerophosphate in a 12-well plate. One milliliter of 0.2% Triton X-100 (Sigma) was added to each well after removing the media. The cell lysates were transferred to a 1.5 ml centrifuge tube, and three freeze–thaw cycles were performed (−70 °C and room temperature, 1 h each) to extract the proteins and DNA from the cells; then, the samples were centrifuged (13,000 × g ) for 15 min at 4 °C. Using the supernatant, the alkaline phosphatase (ALP) activity (n = 5) was measured using p -nitrophenyl phosphate (3.3 mM final concentration) as the substrate in 0.25 M 2-aminomethyl-1-propanol (pH 10.3) and 0.16 mM MgCl 2 . The absorbance was measured at a wavelength of 405 nm using a microplate reader (SpectraMax M2e), and the absorbance was normalized to the dsDNA quantity measured by the PicoGreen assay using the Quant-iT PicoGreen Kit (Invitrogen), following the manufacturer’s protocol. Briefly, 100 μl of the above supernatant was added to 100 μl of the PicoGreen reagent and incubated in the dark at room temperature for 5 min. The absorbance was read at an ex/em of 480/520 nm on the microplate reader (SpectraMax M2e). Five replicate samples (n = 5) were used for each condition. The data from triplicate experiments are shown as the average and standard deviation.
2.9
Statistical analyses
All data are reported as the mean ± standard deviation (SD) of at least three independent experiments. The surface roughness values before and after the NTAPPJ functionalizing were analyzed using a paired t -test. Other statistical analyses were carried out using a one-way ANOVA with Tukey’s method as the post hoc test; significance was set at p < 0.05. Simple linear regression and calculation of Pearson’s correlation coefficient were performed. The significance level was set at p < 0.05. The IBM SPSS statistics 22 software (Armonk, NY, USA) was used for statistical analyses.
2
Materials and methods
2.1
Non-thermal atmospheric pressure plasma jet functionalizing
The NTAPPJ ( Fig. 1 ) was designed and provided by the Plasma Bioscience Research Center (Kwangwoon University Seoul, Korea). Details of the NTAPPJ are described elsewhere and in the supplement . Briefly, the modulated NTAPPJ equipment comprises an inner electrode of tungsten (1.2 mm depth and 0.2 mm thickness) with a 3.2 mm depth of quartz as a dielectric. The hole in the stainless steel outer electrode was 0.7 mm with a 2 mm height of porous alumina having a 150–200 μm pore size with 35% porosity. A high-voltage power supply and Neon Trans (PNP-1000, maximum output voltage 15 kV, current 13 mA, 60 Hz in AC power) were attached to input or control the power, and a mass flow controller (AFC600, Astrovac, Korea) was installed to change the type and flow rate of the gases ( Fig. 1 ). The plasma was formed by passing 1000 standard cubic centimeters per minute (sccm) of nitrogen (99.9%) or compressed air through the plasma-jet device. To produce the ammonia plasma, vaporized ammonia gas was generated by passing 100 sccm of nitrogen through a 28% ammonium hydroxide solution (Sigma Aldrich, USA) with 900 sccm of nitrogen. In total, 1000 sccm of nitrogen-containing ammonia gas passed through the plasma-jet device to treat one specimen. The NTAPPJ functionalizing was applied to the roughened (Sa = 0.5 ± 0.05 μm) Ti and Ti–Ag disk specimens ( ϕ = 12 mm, d = 1 mm) for 10 s with a 3-mm distance between the jet tip and the surface of the specimen. Previously, the effective distance from the center of the NTAPPJ-treated spot was calculated to be ∼15 mm using a polystyrene dish, however, the NTAPPJ effect diminished according to an increase in the distance from the center spot . To obtain uniform NTAPPJ functionalization on the substrate, the disk specimen was manually moved horizontally during treatment time (5 s/cycle). The control samples were not exposed to NTAPPJ because there was no difference in the surface characteristics of the samples with gas only or plasma treatment . To avoid aging effects, which result in a decrease in functionalization according to an increase in incubation time in atmospheric pressure following NTAPPJ treatment , the NTAPPJ-treated substrate was immediately used for further studies. The optical emission spectrum was measured to identify the excited plasma species produced from NTAPPJ using the protocols outlined .
2.2
Specimens
To assess the effects of the NTAPPJ functionalizing on the Ti and Ti–Ag dental implants, Ti and Ti–Ag disks ( ϕ = 12 mm, d = 1 mm) were used as models of dental implant. These disks were prepared as previously described . The Ti disks were kindly provided by Osstem Implant (Busan, Korea). A Ti–Ag alloy, including 2% Ag in Ti, was chosen based on previous results due to its high corrosion resistance and mechanical properties among various Ti–Ag alloys . To generate a rough surface, the specimens were polished using 200-grit SiC sandpaper. After confirming that the surface roughness (Sa) value was within the range of 0.5 ± 0.05 μm, the specimen was used for further study. The specimens were decontaminated and sterilized as previously described . In brief, the specimens were ultrasonically rinsed using a series of acetone, ethanol and distilled water for 10 min each. After drying under a vacuum at 25 °C for 1 day, the specimens were sterilized using ethylene oxide gas and exposed to air for 48 h to eliminate any remaining ethylene oxide gas.
2.3
Surface analysis
A surface analysis was performed before and after the NTAPPJ functionalizing using optical surface profilometry, X-ray photoelectron spectroscopy and contact angle analysis. To visualize the difference in roughness before and after the 10-s NTAPPJ functionalizing, a roughness analysis was performed using an optical surface profilometer (Contour GT-X3 base, Bruker, Germany). Roughness data (Ra, Sa) were obtained as 2D and 3D roughness parameters, respectively. The vertical resolution was no more than 0.1 nm, according to the manufacturer. The optical profilometer optically measured the surface height and represented it in grayscale. The grayscale represents the surface height of a spot. Therefore, the optical profilometer facilitated the evaluation of the differences in the surface roughness at the same position before and after functionalizing. The objective magnification and zoom of the standard camera were ×10 and ×2.0, respectively. The visualized area was 0.23 × 0.31 mm 2 . The scan length and backscan of each analysis were 30 μm. The average (n = 5) and standard deviation (SD) were shown. A contact angle analysis was performed using a Phoenix 300 (SEO, Suwon-si, Gyeonggi-do, Korea). Five microliters of distilled water and ethylene glycol (Sigma Aldrich) were pipetted onto the center of the NTAPPJ-treated and non-treated specimens. After 5 s, the static contact angle was measured, and the average of the right and left values was reported. Five samples were measured from each group. The surface energy was calculated according to the Owens–Wendt method, which uses non-polar (dispersive) and polar components . The room’s temperature was set at 20 °C. The surface energy with non-polar (tension and dispersive) and polar parameters of the test liquids were as follows: surface tension and dispersive and polar forces, respectively, were set at 72.8, 21.8, and 51.0 mJ/m 2 for water and at 48.3, 29.3, and 19.0 mJ/m 2 for ethylene glycol. The X-ray photoelectron spectra (XPS, K-alpha, Thermo Scientific, UK) were obtained using monochromated Al K-alpha. C1s at 284.8 eV was used as a reference, and Ti, C, O, N, and Ag were detected in detail. The surface analysis was independently performed in triplicate, and the representative data are shown.
2.4
Bacterial adhesion assay
S. sanguinis (ATCC 10556, USA) was chosen because, as a pioneer bacterium, it is responsible for the early stages of biofilm formation on dental implants. S. sanguinis was grown in trypticase soy agar (KisanBio, Seoul, Korea) with 5% defibrinated sheep blood (KisanBio). After picking one colony from an agar plate, that colony was suspended in brain heart infusion broth (BHI, KisanBio) and incubated at 37 °C. After 6 h of incubation, when bacteria are in the log phase of growth, the bacterial suspension was centrifuged for 5 min at 5000 × g . The pellets were re-suspended in fresh BHI solution to 5 × 10 7 CFU/ml. The bacteria suspension (1 ml) was pipetted into each well of a 12-well plate; each well contained a single disk specimen. Each specimen was fully immersed in the bacterial suspension and incubated for 2 h at 37 °C (n = 5). To assess the effect of the ions on bacterial attachment, 50 ppb of TiCl 4 or 20 ppb of AgNO 3 were added together or separately. Each disc was washed twice with PBS (Gibco, USA) and transferred to a fresh 12-well culture dish. After the addition of 1 ml of fresh BHI solution with 100 μl of PrestoBlue (Molecular probes, Eugene, OR, USA) to each well, the culture dish was incubated for 1 h to investigate the adherent bacteria numbers. Traditional colony counting methods can cause damage and loss of bacteria during the detachment step from the specimen before spreading the bacteria on an agar plate; to avoid the damage and loss of bacteria, the bacterial cell counting method using PrestoBlue was chosen. The liquid (100 μl) was transferred to a 96-well plate, and the absorbance was read at 570 and 600 nm using a microplate reader (Epoch, BioTek, USA). The absorbance that normalized the 570 nm values to the 600 nm values for each well was used to count the attached bacteria. To confirm the correlation between the normalized absorbance from the PrestoBlue assay and the bacterial numbers, the serial colony-forming unit (CFU) method was used. In short, serial dilutions were generated using BHI, and a 0.1 ml aliquot from each dilution was plated on BHI agar plates (Bacto Agar, BD) and incubated at 37 °C for 24 h. To count the bacteria using the PrestoBlue assay, 10 μl of the chemical was added to each serially diluted bacterial suspension, and a calibration curve was generated. The assays were independently performed in triplicate. The representative means (n = 5) and SD were recorded.
2.5
Confocal laser microscopy
Live-dead staining was performed using SYTO ® 9 and propidium iodide (Thermo Fisher Scientific, Carlsbad, CA, USA) according to the manufacturer’s instructions. Staining solutions were added to each well for observation under a confocal laser microscope (LSM700, Carl Zeiss, Thornwood, NY, USA) with 40× magnification (4× objective × 10× eyepiece). Intense green fluorescence (ex/em = 480/500) was observed from the live cells, and bright red fluorescence (ex/em = 490/635) was observed from the dead cells. The tests were independently performed in triplicate, and the representative images are shown.
2.6
ICP-MS
Inductively coupled plasma mass spectrometry (ICP-MS, ELAN 6100, PerkinElmer, Waltham, MA, USA) was used to determine the ion concentrations (Ti and Ag). Following the incubation of 1 ml of BHI with the NTAPPJ treated or non-treated specimens in a 12-well plate for 2 h at 37 °C, the conditioned solutions were used for measurement.
2.7
Initial cell adhesion test
Rat bone marrow-derived mesenchymal stem cells (rBMMSCs) were taken from the femur and tibia of 5-week-old male Sprague-Dawley rats and cultured as previously described . Briefly, after the femur and tibia were dissected free of the soft tissue, they were placed in α-MEM (Welgene); the sectioned bone and flushed bone marrow were aseptically incubated with a collagenase and dispase solution for 30 min. After centrifugation at 1500 rpm, the cell pellets were cultured in α-MEM supplemented with 10% fetal bovine serum, containing 0.5% antibiotic/antimycotic at 37 °C in an atmosphere of 5% CO 2 . After 5 days of culture, the non-adherent cells were removed. rBMMSCs between passages 2 and 5 were used for this experiment. All animal protocols were conducted according to the standards and regulations of the Institutional Review Board, Dankook University (DKU-11-028). For the initial cell adhesion assay, 1 × 10 5 cells/100 μl were seeded onto the specimen and incubated for 4 or 24 h in a humidifying incubator with 5% CO 2 at 37 °C. After washing three times, the adherent cell numbers were indirectly measured using the cell viability of the adherent cells as measured using the water-soluble tetrazolium salt (WST) assay; the plate reader was used at an absorbance of 450 nm (SpectraMax M2e, Molecular Devices, Sunnyvale, CA, USA) after the incubation with 10% WST salt (EA-Cytox, Daeil Lab, Seoul, Korea) for 1 h. A calibration curve between the number of cells and the absorbance at 450 nm of the WST solution was plotted, and the correlation coefficient was calculated to be 0.995, indicating a strong linear response between the cell number and the absorbance at 450 nm. Confocal microscopy was used to detect the vinculin and actin filaments at 200× magnification (20× objective × 10× eyepiece) after 1 × 10 5 cells/100 μl were seeded on the specimen for 4 h and stained with the actin cytoskeleton and focal adhesion staining kit (Millipore, Bedford, MA) according to the manufacturer’s protocol . The experiments were performed in triplicate, and the representative images are shown.
2.8
Alkaline phosphatase activity
After 1 × 10 5 cells/100 μl of rBMMSCs were seeded onto the specimens for 4 h and washed with PBS, the specimens were incubated in a humidifying incubator at 5% CO 2 and 37 °C for 14 days with or without osteogenic supplement medium (OS) containing 50 μg/ml l -ascorbic acid and 10 mM β-glycerophosphate in a 12-well plate. One milliliter of 0.2% Triton X-100 (Sigma) was added to each well after removing the media. The cell lysates were transferred to a 1.5 ml centrifuge tube, and three freeze–thaw cycles were performed (−70 °C and room temperature, 1 h each) to extract the proteins and DNA from the cells; then, the samples were centrifuged (13,000 × g ) for 15 min at 4 °C. Using the supernatant, the alkaline phosphatase (ALP) activity (n = 5) was measured using p -nitrophenyl phosphate (3.3 mM final concentration) as the substrate in 0.25 M 2-aminomethyl-1-propanol (pH 10.3) and 0.16 mM MgCl 2 . The absorbance was measured at a wavelength of 405 nm using a microplate reader (SpectraMax M2e), and the absorbance was normalized to the dsDNA quantity measured by the PicoGreen assay using the Quant-iT PicoGreen Kit (Invitrogen), following the manufacturer’s protocol. Briefly, 100 μl of the above supernatant was added to 100 μl of the PicoGreen reagent and incubated in the dark at room temperature for 5 min. The absorbance was read at an ex/em of 480/520 nm on the microplate reader (SpectraMax M2e). Five replicate samples (n = 5) were used for each condition. The data from triplicate experiments are shown as the average and standard deviation.
2.9
Statistical analyses
All data are reported as the mean ± standard deviation (SD) of at least three independent experiments. The surface roughness values before and after the NTAPPJ functionalizing were analyzed using a paired t -test. Other statistical analyses were carried out using a one-way ANOVA with Tukey’s method as the post hoc test; significance was set at p < 0.05. Simple linear regression and calculation of Pearson’s correlation coefficient were performed. The significance level was set at p < 0.05. The IBM SPSS statistics 22 software (Armonk, NY, USA) was used for statistical analyses.
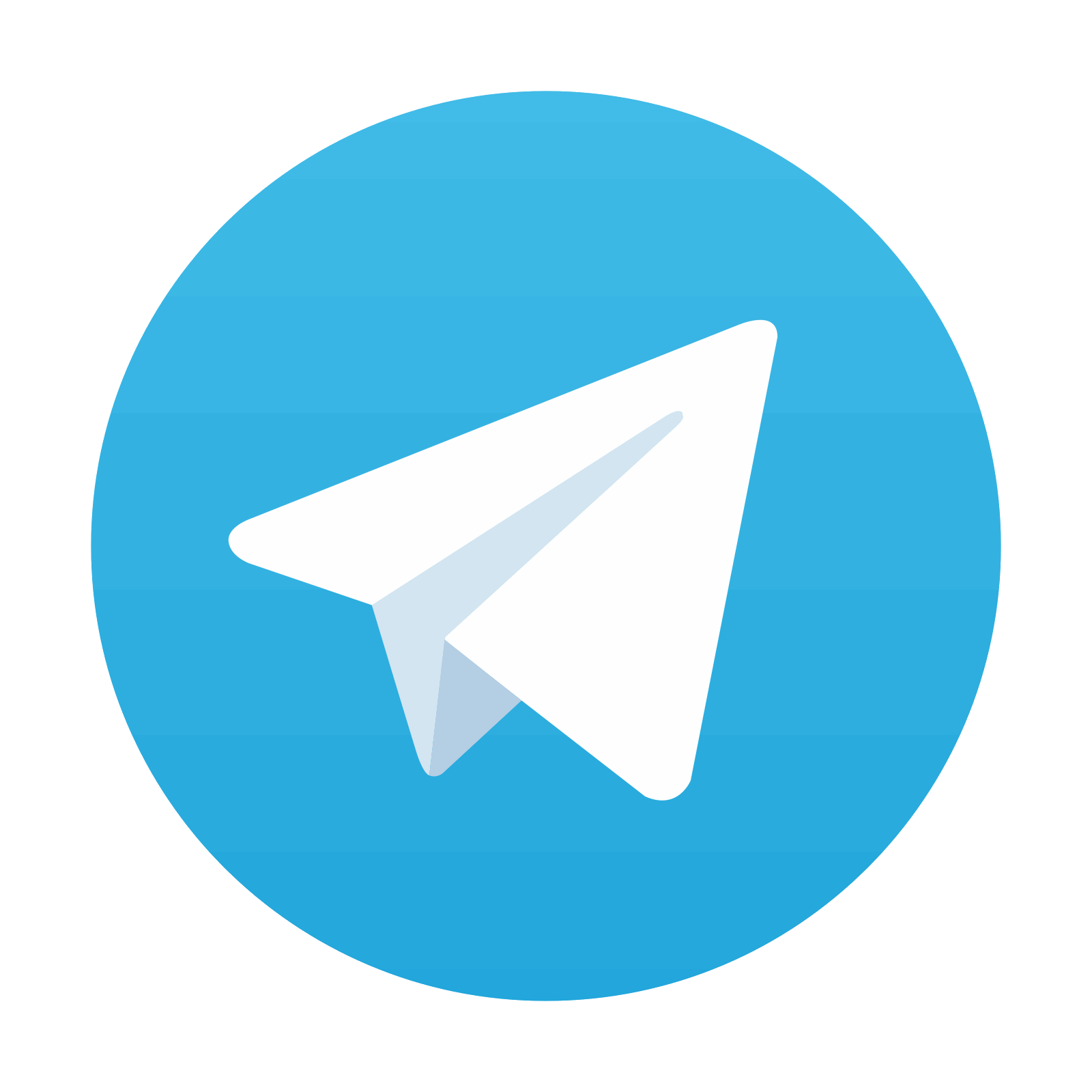
Stay updated, free dental videos. Join our Telegram channel

VIDEdental - Online dental courses
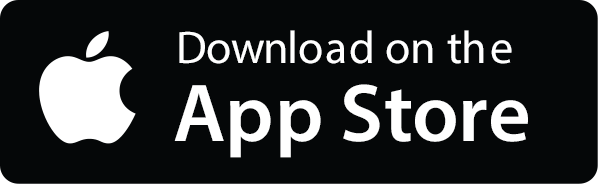
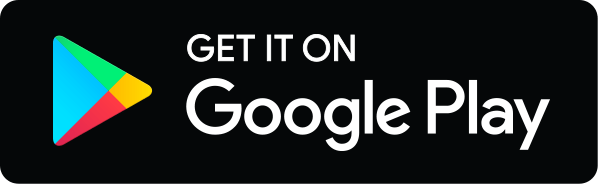