Abstract
Objectives
Regardless of the cause, gap formation at the tooth–restoration interface may result in treatment failure; non-destructive assessment and monitoring of these defects are important. The aim of this in vitro study is to assess the tooth–restoration interface using a non-invasive technique; swept source optical coherence tomography (SS-OCT) and to confirm the findings with confocal laser scanning microscope (CLSM).
Methods
Cylindrical class-I cavities (3 mm in diameter and 1.5 mm in depth) were prepared in the occlusal surface of human premolars. Each cavity was restored using an all-in-one adhesive system (Clearfil Tri-S Bond) and one of the three types of composites placed in bulk; Majesty Posterior, AP-X and Majesty LV (all by Kuraray Medical, Japan). Ten serial cross-sectional images of the whole restored cavity were obtained by SS-OCT at 1319 nm center wave length, to which locations the specimens were later trimmed, polished and observed under CLSM. An image analysis software was used to detect significant peaks in the signal intensity at the resin–dentin interface of the cavity floor. The presence and dimensions of gaps at the interface were also confirmed by CLSM.
Results
Increased SS-OCT signal intensity along the interface corresponded well to the interfacial gaps detected by CLSM. The actual gap size detected ranged from 26 μm to 1.9 mm in length, and the universal composite APX showed lowest interfacial gaps.
Conclusion
SS-OCT imaging technology can be used to non-invasively detect and quantify micrometer gaps at the bottom of composite restorations, and potentially become a monitoring tool for composite restorations both in the laboratory research, and in the clinics.
1
Introduction
Gaps and lack of integrity at the tooth–restoration interface may affect the success of an adhesive restoration. The loss of interfacial seal may result in bacterial microleakage, secondary caries and hypersensitivity of the restored vital tooth, eventually leading to failure of the treatment .
Most of the composite restorations suffer from volumetric polymerization shrinkage . This shrinkage phenomenon generates stresses at tooth–restoration interface that might weaken the restoration integrity, and lead to gap formation. Low adaptation to the cavity might also be the result of the low bonding performance of the adhesive, relatively high stiffness of the uncured composite during placement or insufficient copolymerization of adhesive and bonding agent .
Detection of these micro-defects is important both from the clinical point of view and in dental materials research. It was reported that the gaps mainly occur at the line-angles or at the cavity base, and range from less than a micrometer up to several tens of micrometers in size . They are undetectable by the conventional diagnostic methods, such as radiographic films, which suffer from image superimposition and lack of accuracy on the micron-scale . Conventionally, dye-penetration leakage tests and microscopic assessment of the interface have been employed for in vitro detection of these interfacial gaps. Those methods are highly subjective, and require sectioning of the teeth to evaluate the interface, which make such destructive techniques unsuitable. These disadvantages led to incorporation of newer diagnostic technologies for research on adaptation of restoration, such as 3D imaging by X-ray micro computed tomography (micro-CT) .
Recently, optical coherence tomography (OCT) was addressed as a non-invasive cross-sectional imaging of the internal biological system at the submicron scale . It is a promising imaging modality, which does not require cutting and processing of the specimens and allows the visualization of microstructures of tissue and biomaterials in the real time .
OCT was developed based on the concept of low-coherence interferometry. In simple words, a laser source is projected over a sample, and the backscattered signal intensity from within the scattering medium reveals depth-resolved information about scattering and reflection of the light in the sample. The signal from serial scans can be transformed into an image by a software .
It has been reported in the literature that the first application of low-coherence interferometry in the biomedical optics field was for the eye length measurement by Fercher et al., in 1988. Nowadays, OCT is being used as a clinical diagnostic modality in various medical fields . In dentistry, the first series of reports about imaging of the dental hard and soft tissues appeared in the late 1990s . Afterwards, several researchers used different types of OCT systems for research and diagnosis of dental diseases, including periodontal diseases and early caries lesions . The majority of earlier OCT imaging systems were based on the principles of time-domain low-coherence interferometry. OCT technology has greatly advanced in recent years by the development of spectral discrimination techniques, which provide a substantial increase in sensitivity over traditional time-domain OCT. Swept source OCT (SS-OCT) is one of the most recent implements of the spectral discrimination, using a wavelength-tuned laser as the light source and providing improved imaging resolution and scanning speed .
Some studies have pointed out the potential of OCT for investigation of the gap formation at tooth–restoration interface . However, to our knowledge, few reports have been published in the dental literature focusing on the method development for quantitative gap measurement at the tooth–composite interface using OCT, and validating the results with a common destructive microscopic technique.
In this study, we examined the tooth–composite restoration interface at the cavity floor of the restored teeth by SS-OCT and compared the findings with confocal scanning laser microscope (CLSM). The hypotheses in this study were that an increased OCT signal intensity at the cavity floor under composites indicated existence of interfacial gap, and that this area of increased signal intensity represented gap size, both in length and height.
2
Materials and methods
2.1
Materials used
The materials used in this study are listed in Table 1 . The lot number and chemical composition of each material are according to the information provided by the manufacturer (Kuraray Medical, Tokyo, Japan). Three composite resins were evaluated in this study; Clearfil Majesty Posterior (MJ-POST) is a posterior composite with high nanofiller content, Clearfil AP-X (APX) is a conventional hybrid composite restoration and Clearfil Majesty LV (MJ-LV) is a high filler-loaded flowable composite. An all-in-one self-etching adhesive system, Clearfil Tri-S Bond was used in combination with each one of the composite resins in this experiment.
Material (Manufacturer) Code | Ingredients | Lot no. | Shrinkage a | Composite filler content (weight %) a |
---|---|---|---|---|
Clearfil Majesty Posterior composite restoration (Kuraray Medical, Japan) MJ-POST | Bis-GMA, TEGDMA, silanated silica filler, silanated glass ceramics, surface treated alumina micro-filler, hydrophobic aromatic dimethacrylate, others. | 00107B | 1.5% (volumetric) | 91 |
Clearfil AP-X composite restoration (Kuraray Medical, Japan) APX | TEGDMA, Bis-GMA, silanated barium glass, silanated colloidal silica, silanated silica, others. | 01044A | 1.24% (linear) | 85 |
Clearfil Majesty LV Flowable composite (Kuraray Medical, Japan) MJ-LV | TEGDMA, silanated barium glass powder, silanated colloidal silica, hydrophobic aromatic dimethacrylate, others. | 00214A | 1.88% (linear) | 81 |
Tri-S bond All-in-One Light Cure Adhesive System (Kuraray Medical, Japan) Tri-S | Bis-GMA, MDP, HEMA, ethanol, water, photo-initiators, silanated colloidal silica | 00133A |
2.2
Tooth specimen, cavity preparation and restoration
The experimental design of this study and the use of extracted human teeth were subjected to the guideline of the Ethics Committee of Tokyo Medical and Dental University.
Twenty-one caries-free human premolar teeth were used in this study. After cleaning with a dental scaler, the occlusal cusps were grounded to obtain a flat occlusal table in enamel using a model trimmer, and then polished with 600-grit silicon carbide papers (Sankyo Rikagaku, Saitma, Japan).
The roots of the teeth were cut away, and the remaining coronal portions were trimmed into a block shape with the parallel opposing walls (buccal, lingual, mesial and distal) perpendicular to the side walls (occlusal and cervical). A standard cylindrical class-I cavity with the floor located in dentin, 3 mm in diameter and 1.5 mm in depth was prepared using a flat-end tapered diamond bur (SB2, GC, Tokyo, Japan) attached to an air turbine headpiece under copious cooling water. Clearfil Tri-S Bond was used according to the manufacturer’s instructions. It was applied on the cavity surface for 20 s, dried with gentle air pressure for 5 s and irradiated with a halogen light curing unit (Optilux 501, Kerr, CA, USA; 550 mW/cm 2 ) for 10 s. The teeth were divided into 3 groups according to the study design (MJ-POST, APX and MJ-LV) with 7 teeth in each group. The cavities group were filled with the corresponding composite following the bulk-filling technique and then light-cured for 40 s. A schematic drawing for the sample preparation and visualization under the SS-OCT and CLSM is showed in Fig. 1 a .
2.3
OCT system
The SS-OCT system (OCT-2000 ® , Santec, Komaki, Japan) used in this study had the same setup components as described by Shimada et al. . It is a frequency domain OCT system integrating a high-speed frequency swept external cavity laser, of which the probe power is less than 20 mW, within the safety limits defined by American National Standards Institute. The light source in this system sweeps the wavelength from 1260 nm to 1360 nm at 20 kHz sweep rate with central wavelength at 1319 nm. The axial resolution of this OCT system is 11 μm in air which corresponds to approximately 7 μm within a biomedical structure with a refractive index of around 1.5. The lateral resolution of 17 μm is determined by the objective lens at the probe. The focused light-source beam is projected onto the sample and scanned across the area of interest in two dimensions ( x , z ) using a hand-held probe. Backscattered light from the sample is returned to the system, digitized in time scale and then analyzed in the Fourier domain to reveal the depth-resolved reflectivity profile (A-scan) at each point.
The combination of a series of A-scans along the section of interest creates a raw data file (B-scan) with information on the signal intensity (backscattered light) and x , z coordinates from each point within the scanned area. Two-dimensional cross-sectional images can be created by converting the B-scan raw data into a gray-scale image .
2.4
Tomography imaging of the restored cavities using SS-OCT
The restored cavities were stored in a hydrated state at the room temperature. After 24 h, each sample was fixed on a micrometer head stage and tomographic imaging of the sample was carried out under the SS-OCT. The hand-held scanning probe connected to the SS-OCT was positioned over the occlusal table, with scanning beam oriented at about 90° with respect to the occlusal plane. The B-scans were performed in the mesio-distal cross-sections. Ten B-scan images were obtained along the restoration at 250 μm pitch. Each B-scan corresponded to an image, 8 mm × 6.6 mm in x , z dimensions (2001 pixels × 1019 pixels), obtained in approximately 100 ms.
2.5
Cross-sectional viewing of the cavities using CLSM
Direct observation of the interface in the sample under CLSM (1LM21H/W, Lasertec Co., Yokohama, Japan) was accomplished after trimming off the labial wall of the sample up to the cross-section that was previously imaged by SS-OCT. The trimming was done with different sizes of silicon carbide paper ranging from 280-grit up to 2000-grit in an ascending sequence and the specimens were further polished with diamond paste down to a particle size of (1/4) μm in circular motion under copious cooling water. The cavity floor on each cross-section was imaged at (1250×) magnifications.
2.6
Image analysis
2.6.1
OCT data
SS-OCT raw B-scan data were imported to an image analysis software (ImageJ ver. 1.42q) to detect significant increase in the signal intensity at the resin–dentin interface at the cavity floor. The higher signal intensity appeared as bright clusters formed by bright pixels at the interface. In order to calculate the size of those bright clusters, an image analysis procedure was followed; the size scale was set, and the image was subjected to a median filter (1 px radius) to reduce the noise. The median filter is a non-linear digital filtering technique, often used to remove noise as a typical pre-processing and widely used step to improve the results of later processing. Median filtering is very in digital image processing because under certain conditions, it preserves edges while removing noise . These steps were performed by a plug-in compiled for the software. The image was then cropped to the area including the cavity floor in the center, of which the height was 500 μm (optical), and the width was equal to that of the cavity floor according to the location of the slice. The cropped image of the cavity floor was subjected to the binarization process according to the Isodata algorithm in the auto-threshold function of the software to determine the target pixels with significantly higher brightness compared to other pixels in the background. Image binarization converts a grayscale image to a binary image (bi-level or black and white image), using a certain threshold (cut-off range) to visualize important information in an image. In an automated binarization process, the target image is produced base on a threshold determined automatically using an algorithm. On the binary image, the length of each bright cluster along the cavity floor was calculated. Average height of each cluster was calculated by dividing the length of each cluster on the total area of that cluster.
Bright cluster length percentage of the cavity floor on each cross-section was calculated according to the following equation:
Bright clusters length % = total length of bright clusters at each slice length of the cavity floor at that slice × 100
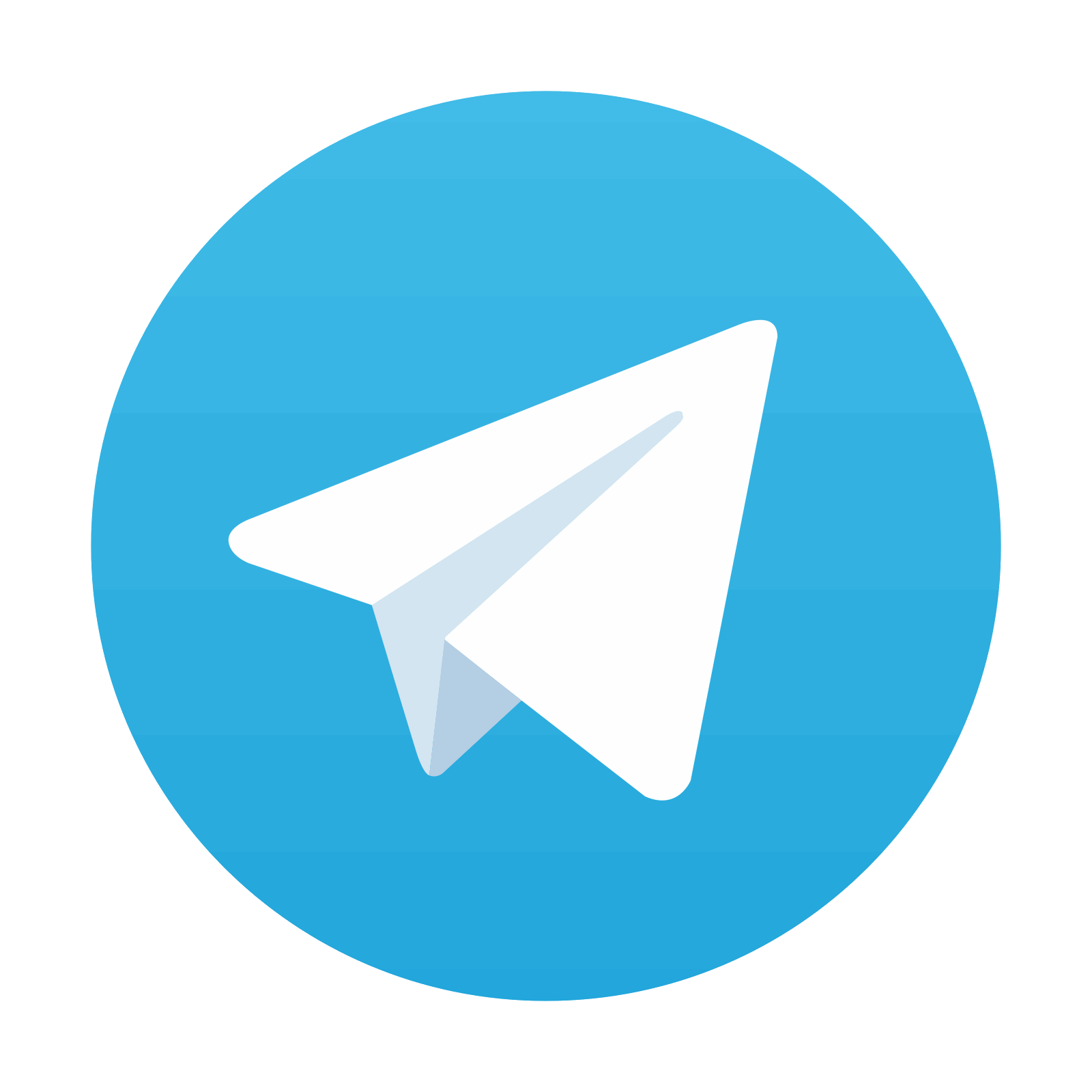
Stay updated, free dental videos. Join our Telegram channel

VIDEdental - Online dental courses
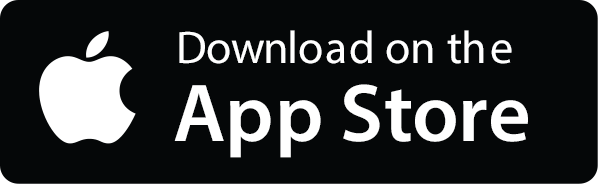
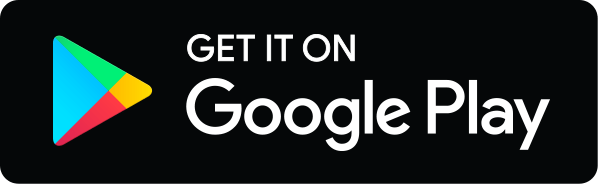