Abstract
Objective
The aim of this study was to evaluate the void frequency ( V F ) and void volume ( V V ) in different flowable composites using swept-source optical coherence tomography (SS-OCT).
Methods
Standard class I cavities were prepared and filled with six different flowable composites: Clearfil Majesty LV (MJ; Kuraray), MI Flow (MW; GC), MI Fil (ML; GC), Beautifil flow plus (BF; Shofu), Palfique Estelite low flow (EL; Tokuyama) and Surefil SDR flow (SF; Dentsply). The restorations were scanned under OCT. The OCT tomograms were analyzed and average V F and V V per restoration for each composite were calculated. Scanning electron microscope (SEM) was used to observe the structure of each composite. In addition, the flowability of the materials was evaluated measuring the displacement of each material placed up-right on a glass slide. V F and V V obtained by OCT were also compared to those calculated using micro-computed tomography (micro-CT).
Results
Kruskal–Wallis ANOVA and Mann–Whitney U tests revealed significantly different V F and V V values ( p < 0.05) among the composites. Voids ranging from 35 to 785 μm in diameter were detected in OCT tomograms. MJ showed highest V F and V V values followed by MW, but ML, BF, EL and SF showed no significant difference. Filler volume in composites showed a positive correlation with void formation, but flowability did not show a specific trend. Micro-CT evaluation validated the V F and V V calculation by OCT, with a significant correlation in void size ( p < 0.001, r = 0.94).
Conclusion
The results of this study indicate the reliability of SS-OCT for real-time void characterization of composite materials and restorations. Void formation in flowable composites is material dependent.
1
Introduction
Defects such as voids and bubbles, which are essentially formed by entrapment of air within the materials, have shown to be detrimental to the mechanical properties of resin composites, particularly under fatigue loading . Within the last decades, advanced composite resin materials have been developed with a focus on high mechanical properties and excellent esthetic. However, the fatigue behavior of composite resins has not shown a correlation with their initial strength values . Fracture analysis has identified voids as critical defects where cracks nucleate under fatigue loading . It is also reported that initial stress concentration around voids will lower the fatigue resistance , increase the wear, and finally lower the durability and performance of the restorations . Accordingly, clinical data indicate that bulk fracture and chipping of the composite restorations are major contributors in clinical failure of composite restorations, which are attributed to the fatigue behavior of the composite materials . Moreover, voids at the margins may lead to gross microleakage and discoloration . Voids between the successive increments of resin composite have adverse effects on the flexural strength of the material , and voids on the surface of the restoration may require extra chair-side time to be removed. In addition, voids may be misinterpreted as secondary caries due to their radiolucent nature in X-ray radio graphs .
A number of methods have been used in order to assess defects in composite materials. Cutting the sample and observing it under microscope is the most basic destructive method . On the other hand, methods that do not require cutting the sample are ultrasonic techniques, X-ray radiography, and micro-computed tomography (micro-CT) . A more recent imaging technique is optical coherence tomography (OCT) . The unique features of this safe technology enable a broad range of research and clinical applications .
OCT is a fundamentally new type of nondestructive and noninvasive optical imaging modality which uses the principle of low coherence interferometry to provide real time 1D depth, 2D cross-sectional and 3D volumetric images with μm level resolution and mm level of imaging depth . OCT applies near infra-red (NIR) radiation to cross-sectionally scan the scattering medium. Images are reconstructed by measuring the backscattered or back reflected light . The OCT technology has improved during the last decade, and swept-source OCT (SS-OCT) is one of the latest OCT systems with improved signal-to-noise ratio and scanning speed . This system is capable of real-time 2D and 3D scanning . In the dental field, the potential of OCT has been explored for oral tissue characterization , dental caries assessment , and interface evaluation of composite restorations . The technology has a great potential for comparative assessment of light-scattering structures such as dental composites . However, to our knowledge, there is no previous record on quantitative evaluation of voids within composites using OCT.
Therefore, the aims of this study were to apply SS-OCT to non-destructively determine the void frequency ( V F ) and void volume ( V V ) in different flowable composites, and to validate findings by micro-CT. The null hypothesis stated that V F and V V values were not significantly different among the tested composites bulk placed in class I cavities.
2
Experimental
2.1
Materials and sample preparation
Thirty extracted bovine incisors were selected for this study. Box formed cavities with round internal line angles 4 mm in diameter and 2 mm in depth were prepared on the flattened labial surfaces using high speed hand piece and round diamond bur (Shofu, Kyoto, Japan). The specimens were randomly distributed among six groups based on the flowable composites used for filling ( n = 5). After applying a dental adhesive (Clearfil SE Bond; Kuraray Medical, Japan) according to the manufacturer’s instructions, each composite resin was injected into the cavity. The composite materials used and their compositions are listed in Table 1 .
Material | Composition | Instruction for light curing by halogen unit |
---|---|---|
Clearfil Majesty LV: MJ (Kuraray Medical) LOT:00306B |
TEGDMA, hydrophobic aromatic dimethacrylate photoinitiator Barium glass, colloidal silica 81 wt.%, 62 vol.% |
Up to 2 mm bulk-fill Light cure 20 s (>300 mW/mm 2 ) |
MI FIL: ML (GC) LOT:1009223 |
UDMA, Bis-MEPP, TEGDMA photoinitiator Silicon dioxide, strontium glass 69 wt.%, 50 vol.% |
Up to 2 mm bulk-fill Light cure 20 s (>700 mW/mm 2 ) |
MI FLOW: MW (GC) LOT:1104111 |
UDMA, Bis-MEPP, TEGDMA photoinitiator Silicon dioxide, Strontium glass 65 wt.%, 50 vol.% |
Up to 2 mm bulk-fill Light cure 20 s (>700 mW/mm 2 ) |
BEAUTIFIL Flow Plus F00: BF (Shofu) LOT: 061012 |
Bis-GMA, TEGDMA photoinitiator Fluoroboroaluminosilicate S-PRG 67.3 wt.%, 47 vol.% |
Up to 2 mm bulk-fill Light cure 20 s (intensity not specified) |
PALFIQUE Estelite Low Flow: EL (Tokuyama) LOT: 670 |
Bis-GMA, TEGDMA, Bis-MPEPP photoinitiator Silica–zirconia 71 wt.%, 53 vol.% |
Up to 2 mm bulk-fill Light cure 30 s (intensity not specified) |
Surefil SDR flow: SF (DENTSPLY) LOT:1103111 |
EBPADMA, TEGDMA, modified UDMA photoinitiator, BHT UV stabilize, TiO 2 , FeO pigment Ba–Al–F–Si glass, Sr–Al–F–Si glass 68 wt.%, 44 vol.% |
Up to 4 mm bulk-fill Light cure 20 s (>550 mW/mm 2 ) |
2.2
SS-OCT system and tomographic procedure
The SS-OCT system used in the present study (IVS-2000, Santec, Komaki, Japan) was a frequency domain OCT with 100 nm bandwidth, centered at 1310 nm spectra at 20 kHz scan rate. The sensitivity of the current OCT system is 106 dB, whilst the shot-noise limited sensitivity is 119 dB. The system incorporated a hand-held probe with a power less than 20 mW. The axial resolution of this system is 11 μm in air, equivalent to approximately 7 μm in oral hard tissue structures and resin composites (assuming a refractive index of around n = 1.5), and the lateral resolution is about 17 μm .
The laser light beam is projected from the probe onto the sample, cross-sectionally scanning the selected area. Backscattered light is collected and digitized in time scale and analyzed in the Fourier domain to reveal the depth-resolved reflectivity profile (A-scan) at each location. The combination of a series of A-scans along a scan path creates a B-scan. B-scans contain the signal intensity value (dB) of each point on the x and z axes. By converting the B-scan raw data into gray-scale, a 2D cross-sectional image can be created. This system is capable of 3D acquisition of data from the structure by serial B-scans over an area.
All the composites were carefully filled into the cavities at room temperature by the same operator following the same placement technique. During injection of the composite into the cavity, the nozzle tip was constantly held away from the dispensed composite. After placing of each composite material into the prepared cavity and irradiation with a halogen light curing unit (Optilux 501, Kerr, Orange, CA, USA) according to the instructions in Table 1 . The specimens were then cross-sectionally scanned with SS-OCT. 3D scans were performed over a volume of interest of 5 mm × 5 mm × 5 mm centered at the cavity obtained in the form of 300 serial sagittal B-scans at a resolution of 760 × 430 pixels ( x , z ).
2.3
Void evaluation
2.3.1
Void volume ( V V )
Fig. 1 demonstrates the appearance of voids in an OCT tomogram. The dimensions of each void were calculated by measuring the distance between the peaks resulting from refraction of light at the top and bottom of each void on the OCT signals from the corresponding A-scan. The volume of each void was calculated, and V V for each restoration was obtained as the sum of void volumes at each restoration in μm 3 ( Fig. 1 ).
2.3.2
Void frequency ( V F )
The total number of independent voids within each restoration was counted in the OCT 3D-scans obtained from each specimen.
2.3.3
Statistical analysis
V V and V F values were statistically analyzed using Kruskal–Wallis ANOVA followed by Mann–Whitney post-hoc test ( α = 0.05). The correlation between the void parameters of the materials was evaluated using the Pearson’s correlation test. All statistical analysis was performed using SPSS (ver. 16, SPSS, Chicago, IL, USA).
2.4
Micro-CT analysis
One of the specimens subjected to OCT scanning was selected and mounted on the computer-controlled turntable of a micro-CT system (InspeXio SMX-100CT; Shimadzu, Kyoto, Japan) so that the X-ray beam was almost perpendicular to the tooth axis and cavity walls. A 0.5-mm-thick aluminum metal sheet was used as a filter for beam-hardening correction . X-rays were generated at 90 kV with 100 μA current. Ten-frame averaging was also applied in the acquisition phase to improve the signal-to-noise ratio. The tooth was rotated 360° within an integration time of 400 s. Data were acquired as TIFF files for reconstruction of the 3D image of the coronal part with 1024 × 1024-pixel resolution and 12-μm isotropic voxel size. A 3D imaging software (TRI/3D-BON; Ratoc, Tokyo, Japan) was used to extract the composite and retain voids based on the CT values contrast between the composite structure and air entrapped in each void. The volume of each void as well as V F and V V parameters were then determined by the software volume calculation function. The micro-CT volume data was then compared to corresponding OCT results by paired t -test and Pearson’s correlation using SPSS ( α = 0.05).
2.5
SEM observation
One specimen from each group was randomly selected and cut across the restoration using a low-speed diamond saw (Isomet, Buehler, Lake Bluff, IL, USA) to obtain a 2 mm thick slice. The slices were polished using 2000 grit SiC paper (Sankyo Rikagaku, Saitama, Japan) followed by a series of diamond pastes with particle sizes from 6 to 1 μm. The slices were gold sputtered and filler particles in the resin matrix of each composite were observed under SEM (JSM 5310 LV, JEOL, Tokyo, Japan) at 2000× magnification.
2.6
Flowability
An equal amount (0.15 g) of each composite was dispensed on a micro-glass slide while the glass was placed on a digital scale. The glass was then kept up-right for 30 s, and the displacement of each material was qualitatively observed on photographs taken from each slice using a digital camera (D90, Nikon, Tokyo, Japan).
2
Experimental
2.1
Materials and sample preparation
Thirty extracted bovine incisors were selected for this study. Box formed cavities with round internal line angles 4 mm in diameter and 2 mm in depth were prepared on the flattened labial surfaces using high speed hand piece and round diamond bur (Shofu, Kyoto, Japan). The specimens were randomly distributed among six groups based on the flowable composites used for filling ( n = 5). After applying a dental adhesive (Clearfil SE Bond; Kuraray Medical, Japan) according to the manufacturer’s instructions, each composite resin was injected into the cavity. The composite materials used and their compositions are listed in Table 1 .
Material | Composition | Instruction for light curing by halogen unit |
---|---|---|
Clearfil Majesty LV: MJ (Kuraray Medical) LOT:00306B |
TEGDMA, hydrophobic aromatic dimethacrylate photoinitiator Barium glass, colloidal silica 81 wt.%, 62 vol.% |
Up to 2 mm bulk-fill Light cure 20 s (>300 mW/mm 2 ) |
MI FIL: ML (GC) LOT:1009223 |
UDMA, Bis-MEPP, TEGDMA photoinitiator Silicon dioxide, strontium glass 69 wt.%, 50 vol.% |
Up to 2 mm bulk-fill Light cure 20 s (>700 mW/mm 2 ) |
MI FLOW: MW (GC) LOT:1104111 |
UDMA, Bis-MEPP, TEGDMA photoinitiator Silicon dioxide, Strontium glass 65 wt.%, 50 vol.% |
Up to 2 mm bulk-fill Light cure 20 s (>700 mW/mm 2 ) |
BEAUTIFIL Flow Plus F00: BF (Shofu) LOT: 061012 |
Bis-GMA, TEGDMA photoinitiator Fluoroboroaluminosilicate S-PRG 67.3 wt.%, 47 vol.% |
Up to 2 mm bulk-fill Light cure 20 s (intensity not specified) |
PALFIQUE Estelite Low Flow: EL (Tokuyama) LOT: 670 |
Bis-GMA, TEGDMA, Bis-MPEPP photoinitiator Silica–zirconia 71 wt.%, 53 vol.% |
Up to 2 mm bulk-fill Light cure 30 s (intensity not specified) |
Surefil SDR flow: SF (DENTSPLY) LOT:1103111 |
EBPADMA, TEGDMA, modified UDMA photoinitiator, BHT UV stabilize, TiO 2 , FeO pigment Ba–Al–F–Si glass, Sr–Al–F–Si glass 68 wt.%, 44 vol.% |
Up to 4 mm bulk-fill Light cure 20 s (>550 mW/mm 2 ) |
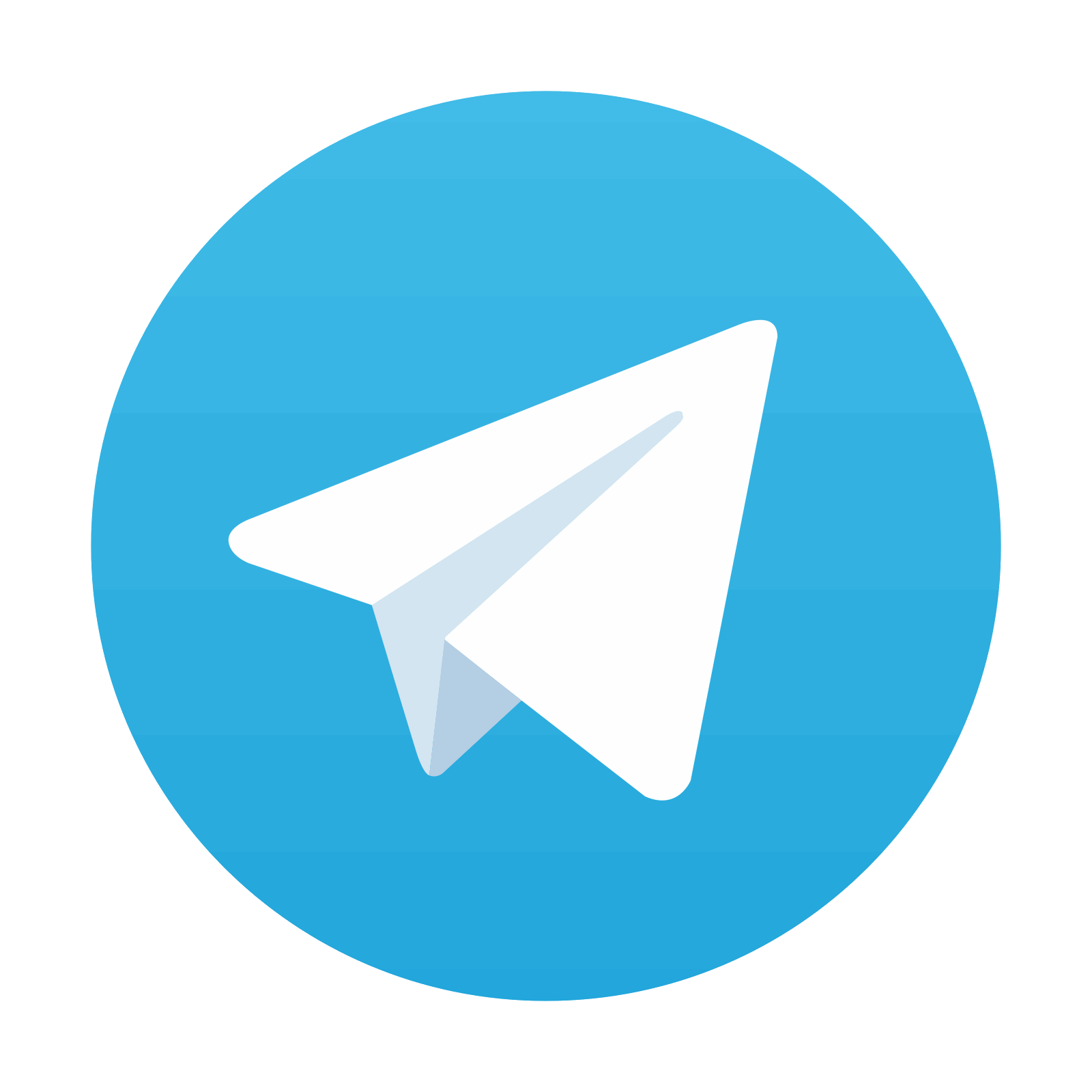
Stay updated, free dental videos. Join our Telegram channel

VIDEdental - Online dental courses
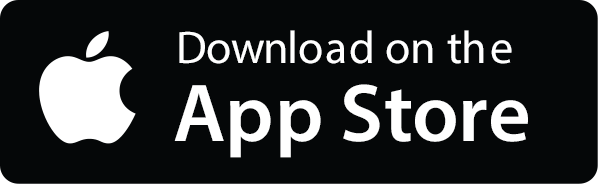
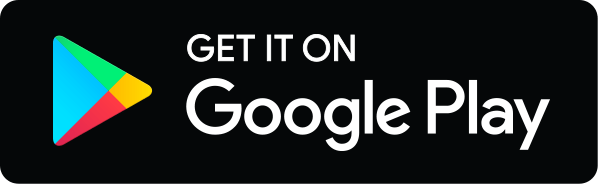
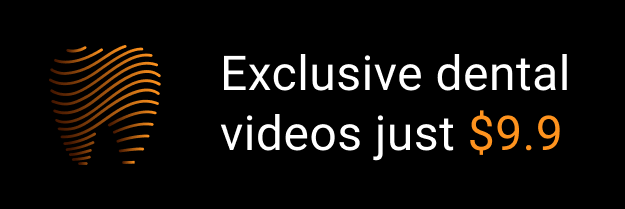