15
Non‐surgical Antimicrobial Photodynamic and Photothermal Therapy in Treatment of Peri‐implant Mucositis and Peri‐implantitis
Manolis Vlachos1 and Ioannis Fourmousis2
1 Dental Excellence, Private Dental Clinic, Athens, Greece
2 Department of Periodontology, School of Dentistry, NKUA, Athens, Greece
Introduction
Peri‐implant mucositis and peri‐implantitis are pathological conditions affecting tissues surrounding dental implants. Many factors are contributing etiologically to the pathology of these diseases [1]. The major factor is considered to be microbial plaque (or microbial biofilm). Patients who do not follow daily oral hygiene routine and are not under regular maintenance therapy have been shown to be at a higher risk for developing peri‐implant disease [2]. There are also secondary etiological and predisposing factors such as smoking, diabetes mellitus, radiation, genes associated with host response that contribute to peri‐implant pathology [2, 3].
The main goal of treatment of peri‐implant diseases of microbial etiology is the (i) effective removal of microbial biofilm, (ii) disinfection of implant and prosthetic restoration surfaces, (iii) inflammation resolution and stabilization of marginal bone level, and (iv) regeneration of peri‐implant tissues (if feasible) [4]. Treatment can be (i) surgical (resective and/or regenerative techniques), or (ii) non‐surgical (mechanical removal of microbial biofilm using ultrasonic scaling, curettes, or air‐polishing without raising a flap) [4]. Additional methods that have been studied include (i) systemic administration of antibiotics or application of antimicrobial mouthwashes, (ii) local application of antibiotic or antimicrobial gels in peri‐implant pocket, (iii) mechanical or chemical alteration of implant surfaces, as well as (iv) application of dental lasers [5].
The efficacy of dental lasers in treating peri‐implant diseases has been studied either as monotherapy or adjunctive therapy during i) disease control phase, ii) surgical phase (implant placement), or iii) irradiation phase of implant surfaces for disinfection or modification. Various types of lasers have been investigated: i) neodymium lasers (Nd:YAG, Neodymium‐Doped Yttrium Aluminum Garnet, 1064 nm), ii) Erbium lasers (Er:YAG, Erbium Yttrium Aluminum Garnet, 2940 nm and Er,Cr:YSGG, Erbium, Chromium‐Doped Yttrium Scandium Gallium Garnet, 2780 nm), iii) Carbon Dioxide lasers (CO2, Carbon Dioxide, 10600 nm), as well as iv) Diode lasers (810, 940, 980, and 1064 nm) [4]. Low‐level lasers have been studied in a specific type of therapy termed as “Photodynamic Therapy” or “Photothermal Therapy”.
Photodynamic Therapy
Photodynamic therapy is a treatment modality that includes application of low‐level laser light for activation of a non‐toxic hydrophilic photosensitive substance, termed as photosensitizer, usually in an oxygen‐rich environment. This method was discovered at the beginning of the twentieth century by Raab who reported that Paramecium caudatum could be killed after incubation with acridine hydrochloride or eosin and concomitant exposure to daylight (but not to the dark) [6]. At that period, therapy with local eosin and light was studied in skin carcinoma treatment for first time [7]. At the same time, the requirement for oxygen during this photosensitive reaction was reported and the term “photodynamic action” was introduced for first time a few years later by von Tappeiner [8]. Antimicrobial photodynamic therapy (aPDT) was first introduced by MacMillan and his colleagues in 1966, who applied a continuous wave gas laser (632 nm) to activate Toluidine Blue Ο and killed bacterial microorganisms including bacteria and yeast [9]. Due to discovery of antibiotics and their bactericidal activity, PDT application was forgotten for many years, until resistance to antibiotics developed by many bacteria and multi‐drug resistance has led to the increasing scientific interest and research on alternative antibacterial treatment modalities such as aPDT.
Photodynamic therapy has been applied as a method for treating conditions such as carcinoma and actinic keratosis until Wilson et al. reported its application for inactivation of oral and periodontopathogenic bacteria [10–12]. More specifically, Streptococcus sanguis, Fusobacterium nucleatum, Porphyromonas gingivalis, and Actinobacillus actinomycetemcomitans were reported to be killed by applying Toluidine Blue O, Azure B Chloride, or Methylene Blue and activating them by a He:Ne laser. The technique was effective in inactivation of these species not only in culture form but even when they were organized into biofilms. In a report from the same group of colleagues at the same period, subgingival microbial plaque samples from patients with chronic periodontitis were incubated with Toluidine Blue O and activated by He:Ne laser [13]. Photodynamic Therapy led to viability reduction of all different periodontopathogenic species of the samples (aerobes, anaerobes, black‐pigmented anaerobes, P. gingivalis, F. nucleatum, and streptococci) (only a minor toxicity was caused by the dye alone). Due to its targeted activity against microorganisms, Photodynamic Therapy was also termed as Photodynamic Antimicrobial Chemotherapy (PACT), antimicrobial Photodynamic Therapy (aPDT), or Lethal Photosensitization [14, 15].
As it was mentioned above, the main principle of this treatment modality lies in the application of a photoactive dye which is termed as “photosensitizer.” Photosensitizers, applied either locally, or been administered intravenously, consequently bind to target bacterial cells and are activated by light of specific wavelength in the presence of oxygen [16]. Theoretically, neither light of a specific wavelength nor photosensitizers alone can cause cytotoxic effects on cells. Lasers can transmit monochromatic, high‐intensity but low‐level energy to the photosensitizers which can be highly effective for activation of photodynamic reaction.
After light activation, the photosensitizer is promoted from its stable ground state to an excited triplet state. Electrons from highly excited states can return to their ground states, and during this transition, light or heat emission can be observed [17]. The excited triplet state photosensitizers react with their substrate following two different pathways termed as Type I and Type II reactions, depending on the photosensitizer applied and the existing conditions [18–21]. In Type I reaction, electrons are transferred from the excited triplet state photosensitizer to organic substrate molecules within the cells. This leads to production of other reactive agents (free radicals and radical ions) that react with molecular oxygen and produce reactive oxygen species such as hydroxyl radical, superoxide and hydrogen peroxide. These reactive oxygen species react with molecules inside bacterial cells and cause damage to them. Type II reaction includes the interaction of excited triplet‐state photosensitizer with ground‐state oxygen that leads to production of singlet oxygen (1O2). Singlet oxygen can also interact with many molecules inside cells leading to their oxidation. Both reactions can eventually damage the cell wall and cytoplasmic membrane, leading to cell lysis and cell death by necrosis or apoptosis [22].
Singlet oxygen (1O2) is considered to be the primary reactive agent of photodynamic reaction, so most of the effects inside microbial cells are mediated by Type II reaction [23]. Due to the short lifetime of singlet oxygen in cells, the radius of activity of singlet oxygen is also short. As a result, photodynamic reaction is limited to the region of photosensitizer application, and its effects are restricted to a small targeted region of activity, without affecting cells and tissues at a distance.
aPDT offers many advantages in comparison to surgical or non‐surgical treatment modalities (mechanical instrumentation, local or systemic administration of antibiotics, etc.) [23]. When aPDT is performed, patients avoid undergoing the unpleasant experience of a surgical procedure. Furthermore, this treatment modality can be performed even in patients with compromised medical history. Due to application of low‐level lasers, the energy and heat that is transferred to the implant surface is very low. Photosensitizers require less energy to become activated and inactivate pathogenic bacteria. As a result, the overall reaction does not cause any damage to the implant surface and neighboring healthy tissues (carbonization, cracking, or melting). Furthermore, aPDT presents a promising treatment method for overcoming limitations of classical mechanical instrumentation methods to decontaminate implant surfaces, even in difficult‐to‐access regions without raising a flap, and this effect can be completed in a very short time period which is controllable in a light‐dependent manner (wavelength, power, and time of irradiation), and without causing trauma to the neighboring tissues. In contrast to antibiotics, aPDT is advantageous as it overcomes one major disadvantage of antibiotics administration (as it has been reported for periodontal microflora) and does not lead to development of antibiotic‐resistant strains [24]. This is due to non‐target specific mechanism of action of aPDT which is based on the production of highly reactive cytotoxic molecules, as mentioned above. Due to the target‐specific activity of antibiotics, many of them would be required to inactivate different species of bacteria in microbial biofilm. However, aPDT can inactivate different microorganisms non‐specifically due to its mechanism of action. Furthermore, the effect of laser irradiation on the photosensitizer can be diffused to the inner layers of microbial biofilm, an effect that is difficult to achieve by antibiotics [24].
Many photosensitizers have been applied in aPDT, in general, and more specifically in treatment of periodontal and peri‐implant diseases. Ideally, they should have the following features: (i) absence of toxicity, (ii) high binding affinity, specificity and absorption for target diseased cells/tissues and low for healthy cells/tissues, (iii) quick accumulation at the target cells/tissues and short half‐life, in order to be dispersed at a specific radius from the desired target region of tissue and not affect non‐desired tissue regions, (iv) have a broad spectrum of activity, (v) should be activated by a specific wavelength of light and be able to produce a significant quantity of cytotoxic molecules [25].
Since the first in vivo human study published in 2001 by Doertbudak et al. (that applied aPDT after raising a flap), many reports have been published in PubMed database of the US National Library of Medicine so far that have applied non‐surgical aPDT in peri‐implant disease treatment (Table 15.1) [14]. The most common photosensitizers applied in these studies are Toluidine Blue O [Tolonium Chloride: (7‐amino‐8‐methyl‐phenothiazin‐3‐ylidene)‐dimethyl‐ammonium (C15H16N3S+)], Methylene Blue [3,7‐bis(dimethyl‐amino) phenazathionium chloride tetramethylthionine chloride (C16H18N3ClS) or phenothiazine‐5‐ium, 3,7‐bis(dimethylamino)‐chloride], and Phenothiazine Chloride (Table 15.1).
Toluidine Blue has a blue–violet color, while MB in its oxidized form is blue and becomes colorless in its reduced state. Both photosensitizers are similar in their features and are effective against periodontopathogenic bacteria (both Gram+ and Gram−) [59]. MB is a cationic dye with its absorption peak at light of 660 nm wavelength, while Toluidine blue is also cationic with its absorption peak at light of 635 nm wavelength for inactivating bacterial cells. Phenothiazine Chloride is also a blue photosensitizer (its main ingredient is Toluidine blue) and its maximum absorbance is at 670 nm wavelength of light. In seven studies published so far in PubMed database of the US National Library of Medicine, aPDT with MB was performed together with mechanical debridement or glycine air‐polishing (Table 15.1). MB was activated by (i) 630 nm, (ii) 635 nm, (iii) 660 nm, or (d) 670 nm wavelength of light. In six out of seven studies, aPDT resulted in improvement of clinical and/or microbiological and immunological parameters in these studies. The only study that did not report any additive positive result after aPDT with MB was that done by Alresheedi and Alazmi [55]. In five studies published so far in PubMed database of the US National Library of Medicine, aPDT with Toluidine Blue was performed in combination with scaling and/or mechanical debridement and/or subgingival glycine air‐polishing and/or professionally administered plaque removal and/or home‐based administration of probiotics, and/or irrigation of peri‐implant pocket with saline solution. In these studies, Toluidine Blue was activated by (i) LED, (ii) 630 or (iii) 635 wavelength of light. In all of these studies, aPDT with Toluidine Blue resulted in improvement of clinical parameters examined in each study. Finally, nine studies have been published so far in PubMed database of the US National Library of Medicine, reporting aPDT with phenothiazine chloride. In all of these studies, phenothiazine chloride was activated by 660 nm wavelength of light. APDT was performed as sole treatment or in combination with air‐polishing and/or mechanical debridement and/or scaling and root planing.
An overview of all of these studies (samples, methodology followed, results) investigating the effectiveness of non‐surgical photodynamic therapy in treatment of peri‐implant diseases published in PubMed database of the US National Library of Medicine so far are presented in Table 15.1. A brief comment is that in many studies, aPDT either as sole or adjunctive treatment leads to short‐term improvement (three to six months) of clinical, microbiological, and immunological parameters in patients with peri‐implant disease in comparison to control groups that have undergone only classical treatment methods (e.g. mechanical debridement, air‐polishing, local, or systemic administration of antibiotics). In some of the studies, no additional benefit from aPDT in any of the parameters measured was reported. There are some studies that presented some limitations (no control groups). APDT is a technique, that as mentioned above, due to its advantage of not causing resistant strain growth, could be repeated many times to achieve better results if possible without having adverse effects (in some studies, repeated application of therapy in regular sessions after initial therapy has been reported). There are some studies in which aPDT has reported improvement levels of Hb1Ac in diabetic patients with peri‐implant disease, while in others no additional benefit was observed. All these results are shown in Table 15.1. Although in some of the studies, no details of methodology are provided either in abstract or in full text, it is obvious that there has been increasing interest in ongoing research around application of this treatment in therapy of peri‐implant mucositis and peri‐implantitis.
Photothermal Therapy
In recent years, another photosensitizer reported to be applied in a more contemporary version of aPDT is Indocyanine Green (Table 15.2). This photosensitizer is anionic, hydrophilic, and lipophilic, and shows its absorption peak at 810 nm. This wavelength is different from wavelengths applied with the other photosensitizers in aPDT (Methylene Blue, Toluidine Blue, and Phenothiazine Chloride), has a greater penetration depth compared to them, which is favorable in deep periodontal and peri‐implant pockets. In contrast with photosensitizers used in aPDT, Indocyanine Green’s main effect derives primarily from its molecules’ vibration and heat release inside target cells/tissues, and at a secondary degree through production of cytotoxic reactive agents (thought to be 80% photothermal and 20% photochemical) [23, 63]. Due to its different mechanism of action, this type of aPDT is termed as “Photothermal Therapy.” Photothermal therapy with Indocyanine Green was initially applied in treatment of acne bulgaris [64]. Indocyanine green has been shown to be selectively absorbed by periodontopathogenic bacteria (P. gingivalis and A. actinomycetemcomitans) [63].
Table 15.1 In vivo human studies of non‐surgical antimicrobial photodynamic therapy in treatment of peri‐implant diseases.
Study Authors | Sample | Type of intervention Groups | Results | |||
---|---|---|---|---|---|---|
Deppe et al. [26] | 16 patients with peri‐implantitis (PD ≤8 mm) | PD <5 mm: PC for 3 min, Diode laser (660 nm)(100 mW, continuous, 1 min) | PD = 5–8 mm: PC for 3 min, Diode laser (660 nm)(100 mW, continuous, 1 min) | PD = 5–8 mm group: At 6 months, significant increase of CAL and DIB | ||
Schar et al. [27] | 40 patients with peri‐implantitis (PD = 4–6 mm) Mean age: 58 years |
MD, Subgingival Glycine Air‐Polishing, Minocycline microspheres in peri‐implant pocket. If residual BOP was recorded at 3 and 6 months, 1 additional session was performed each time | MD, Subgingival Glycine Air‐Polishing, PC for 3 min, Diode laser (660 nm)(100 mW, continuous, 10 sec per site)(repeat after 1 week). If residual BOP was seen at 3 and 6 months, 1 additional session was performed each time | Comparable reduction in mucosal inflammation, PD, and gain of CAL up to 6 months in both groups | ||
Thierbach et al. [28] | 28 patients with peri‐implantitis (PD>5 mm) Mean age: 50.6 ± 9.41 years |
PUS: Full mouth SRP. At 2 months, additional SRP, PC for 3 min, Diode laser (660 nm)(1 min). At 4 months surgical treatment | No PUS: Full mouth SRP. At 2 months, additional SRP, PC for 3 min, Diode laser (660 nm) (1 min) | PD, BOP, and aMMP‐8 levels improved in both groups. PUS group: peri‐implantitis can be effectively treated with additional surgical treatment at 4 months | ||
Bassetti et al. [29] | 40 patients with peri‐implantitis (PD = 4–6 mm) Mean age: 58 years |
MD, Subgingival Glycine Air‐Polishing, Minocycline microspheres in peri‐implant pocket. If residual BOP was seen at 3, 6, 9, or 12 months, 1 additional session was performed each time | MD, subgingival glycine air‐polishing, PC for 3 min, Diode laser (660 nm)(100 mW, continuous) for 10 sec per site (repeat after 1 week). If residual BOP was seen at 3, 6. 9, 12 months, 1 additional session was performed each time | At 12 months: significant reduction of BOP and CF levels of IL‐1β in both groups At 9 months (in aPDT group) and at 12 months (in control group) significant reduction of PD At 6 months (in aPDT group) and at 12 months (in control group) significant reduction of P.g and T.f |
||
Al Amri et al. [30] | 67 patients with T2DM and peri‐implant inflammation Mean age: 51.4 ± 3.7 years (control group), 53.6 ± 9.5 years (aPDT group) |
MD | MD, PC for 2 min, Diode laser (660 nm)(100 mW, 10 sec per site) | aPDT group: significant difference in HbA1c levels at 6 and 12 months. BOP and PD (not MBL) were significantly reduced at 6 and 12 months | ||
Javed et al. [31] | 84 smokers, 82 non‐smokers Peri‐implant diseases |
MD | MD, PC for 2min, and Diode laser (660 nm)(100 mW, 10 sec per site) | At 6 months: PD higher in smokers vs non‐smokers. BOP, PD, and CBL were comparable between smokers and non‐smokers at 12 months. BOP was comparable among smokers at all‐time intervals | ||
Karimi et al. [32] | 10 patients, 15 pairs of dental implants with peri‐implant mucositis (PD ≤ 5 mm) or peri‐implantitis (PD >5 mm) | Control implants: Scaling aPDT implants: Scaling, TBO for 3 min, LED (2000 mW, 2 min) |
Significant difference in PD, CAL, BOP, and GI at each time point between two groups. No significant difference in any parameter in control group. At 3 months, complete resolution of BOP in all aPDT implants. At 1.5 and 3 months, significant difference in PD and CAL gain in aPDT implants | |||
Romeo et al. [33] | 40 patients with peri‐implantitis (PD ≥ 4 mm) Range of age: 34–68 years |
Glycine Air‐Polishing, MD | Glycine Air‐polishing, MD, MB for 1 min, Diode laser (670 nm) (75 mW, 1 min) | After 24 weeks, in aPDT group, improved PD, BOP, and PI, with an average PD value of 2 mm (control group 3 mm) | ||
Abduljabbar [34] | 60 prediabetic patients with peri‐implant diseases (PD ≥ 4 mm) | MD | MD, aPDT | At 3 months (but not at 6 months), BOP and PD were significantly higher in control group. HbA1c levels were comparable in all groups at all time intervals | ||
Abduljabbar [35] | 64 patients with T2DM and peri‐implant diseases (PD ≥ 4 mm) Mean age: 52.6 ± 0.8 years (smokers), 54.4 ± 1.2 years (non‐smokers) |
T2DM smokers: MD, aPDT | Non‐T2DM smokers: MD, aPDT | At 6‐months, no significant difference in BOP and PD among patients in groups 1 and 2 versus baseline. HbA1c levels were comparable in all groups at all time intervals | ||
Al‐Sowygh [36] | 48 male, smokeless tobacco consuming patients Mean age: 46.8 ± 5.7 years (control group), 42.5 ± 6.5 years (aPDT group) |
MD | MD, aPDT | At 3‐months, PI, BOP, and PD were significantly higher in control group vs aPDT group | ||
Javed et al. [37] | 54 male patients, smokers with peri‐implant mucositis (PD ≥ 4 mm) Mean age: 52.2 ± 0.5 years (control group), 50.6 ± 0.8 years (aPDT group) |
MD | MD, PC for 2 min, Diode laser (660 nm)(100 mW, 10 sec per site) | PD and PI were significantly higher in control versus aPDT group at 12 months | ||
Mongardini et al. [38] | 20 patients with experimentally induced peri‐implant mucositis Mean age: 57 ± 11 years |
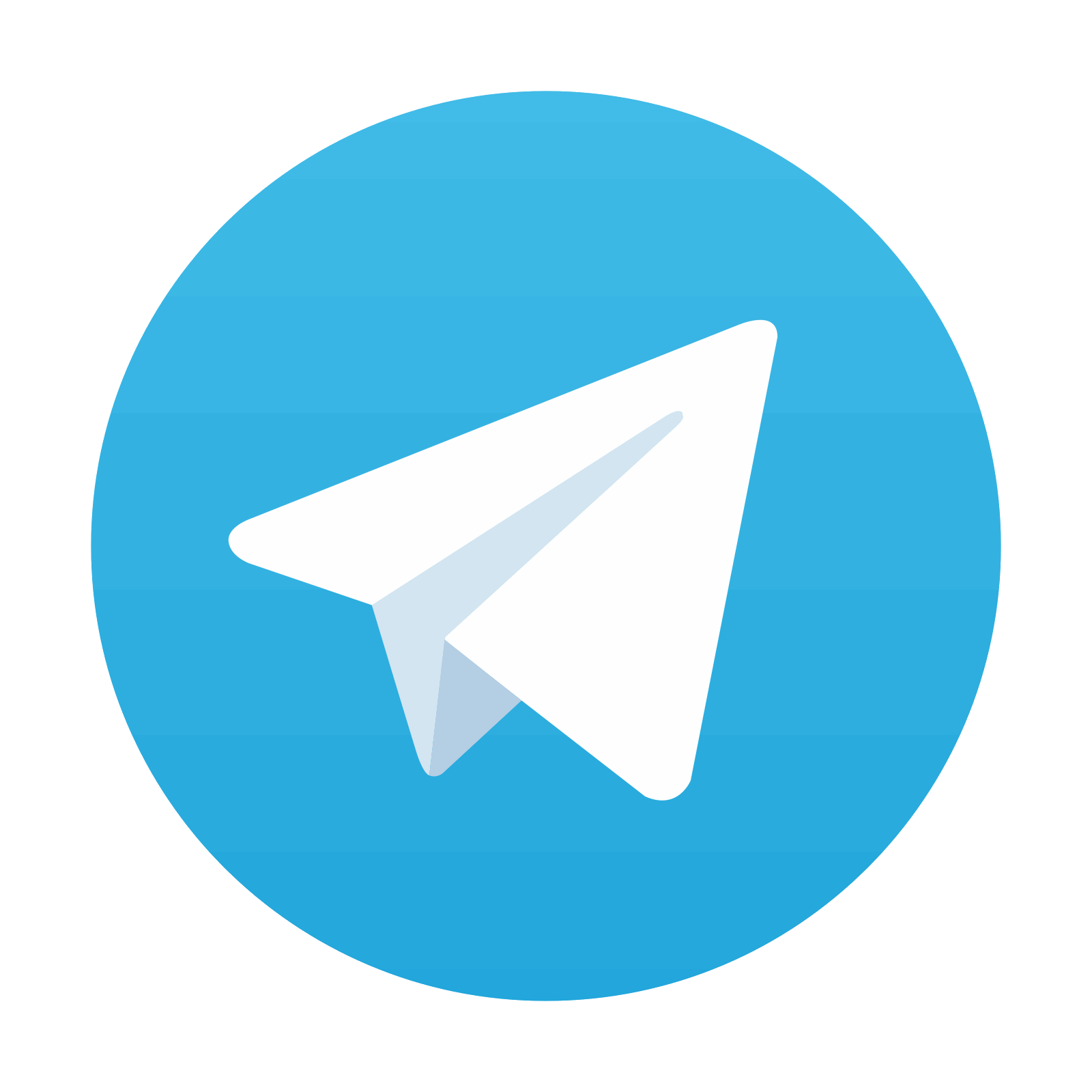
Stay updated, free dental videos. Join our Telegram channel

VIDEdental - Online dental courses
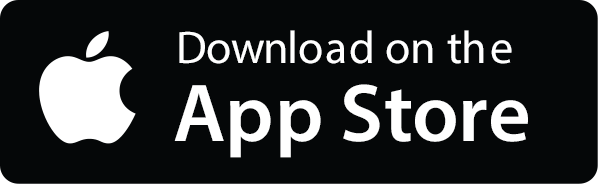
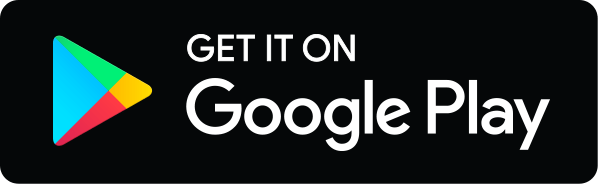