Abstract
Background and objectives
Current clinical methods for diagnosing secondary caries are unreliable for identifying the early stages of decay around restorative materials. The objective of this study was to access the integrity of restoration margins in natural teeth using near-infrared (NIR) reflectance and transillumination images at wavelengths between 1300 and 1700-nm and to determine the optimal NIR wavelengths for discriminating composite materials from dental hard tissues.
Materials and methods
Twelve composite margins ( n = 12) consisting of class I, II and V restorations were chosen from ten extracted teeth. The samples were imaged in vitro using NIR transillumination and reflectance, polarization sensitive optical coherence tomography (PS-OCT) and a high-magnification digital microscope. Samples were serially sectioned into 200-μm slices for histological analysis using polarized light microscopy (PLM) and transverse microradiography (TMR). Two independent examiners evaluated the presence of demineralization at the sample margin using visible detection with 10× magnification and NIR images presented digitally. Composite restorations were placed in sixteen sound teeth ( n = 16) and imaged at multiple NIR wavelengths ranging from λ = 1300 to 1700-nm using NIR transillumination. The image contrast was calculated between the composite and sound tooth structure.
Results
Intensity changes in NIR images at wavelengths ranging from 1300 to 1700-nm correlate with increased mineral loss measured using TMR. NIR reflectance and transillumination at wavelengths coincident with increased water absorption yielded significantly higher ( P < 0.001) contrast between sound enamel and adjacent demineralized enamel. In addition, NIR reflectance exhibited significantly higher ( P < 0.01) contrast between sound enamel and adjacent composite restorations than visible reflectance.
Significance
This study shows that NIR imaging is well suited for the rapid screening of secondary caries lesions.
1
Introduction
Secondary (recurrent) caries is the major etiologic factor in the failure of dental restorations, and dentists spend more time replacing restorations than placing new ones . Conventional clinical methods for evaluating demineralization surrounding restorations rely upon visible inspection for discoloration and gaps/microleakage, and tactile sensation with an explorer or periodontal probe. It is clear from clinical data that neither discoloration nor ditching (for gap distances <500-μm, which are considerable) is a consistent indicator of the integrity of the enamel or dentin, and a considerable number of restorations are unnecessarily replaced using such criteria . Inspection via tactile examination also presents the risk of accelerating decay by damaging the protective lesion surface zone. New imaging methods that can discriminate between sound enamel, demineralized enamel and composites with higher diagnostic performance for secondary caries lesions are needed.
To address this need, optical techniques including digital radiographs (X-rays), quantitative laser fluorescence (QLF, collagen fluorescence), and red laser fluorescence (LF, porphyrin fluorescence) have been investigated as alternative, nondestructive approaches for diagnosing recurrent decay, albeit that each modality has significant limitations.
Digital radiographs lack the sensitivity to detect changes present during the early stages of lesion progression. Generating radiographic images requires ionizing X-rays and a direct viewing angle of the interproximal regions to produce a diagnostic image . When imaging restored teeth, amalgam and composite restorations are radiopaque and may mask the presence of caries either partially or completely which contributes to the difficulty of diagnosis, and therefore are poorly suited for detecting secondary caries .
Quantitative light fluorescence (QLF) uses UV or blue light ranging from 370 to 470-nm in wavelength to excite the proteins in enamel and dentin, and identifies lesions by measuring the loss of fluorescence at wavelengths >500-nm (green light) . Measuring lesion severity based on a loss of signal with visible light is problematic due to the high absorption from stain that attenuates light and results in false positives using this method. Secondary caries detection using QLF has been comparable in accuracy to visual diagnosis .
Laser fluorescence (LF) uses ∼655-nm light to excite fluorescence of bacteria-produced porphyrins and measures light emission at wavelengths >680-nm (near-infrared) . In LF, the presence of a fluorescent signal is used to locate infected areas as a function of bacteria concentration and studies suggest that LF out performs visible and QLF methods for the detection of recurrent decay . Unfortunately, LF is prone to false positives due to poor correlation between porphyrin concentration and lesion severity and requires cleaning of tooth surfaces for effective use. Furthermore, the excitation wavelengths used in LF has been shown to cause fluorescence in many dental restorative materials, including some sealants . For LF devices such as the DIAGNOdent, this requires the user to continually calibrate the device against a ceramic standard, and against sound enamel for each individual tooth .
Near-infrared (NIR) imaging has the potential for improved performance over current methods used to detect recurrent decay by illuminating and capturing images of tooth structures formed by deeply penetrating, non-ionizing light ranging from 700 to 1700-nm in wavelength. Compared to visible light, light scattering in sound enamel at λ = 1300-nm is ∼20×–30× less making sound enamel virtually transparent . When sound enamel becomes demineralized by caries, pores within the lesion grow to a similar size of the wavelength of light and act as Mie scatterers increasing the scattering coefficient 2–3 orders of magnitude . Differences in light scattering between sound and demineralized enamel can be detected by imaging light transmitted through or reflected back from the tooth . Imaging with longer wavelength ( λ ≥ 1300 nm) NIR light also avoids the absorption bands of organic molecules responsible for pigmentation allowing direct imaging of decay beneath stained surfaces such as the occlusal grooves . Additionally, composite restorative materials have unique spectral signatures in the NIR resulting from combination absorption bands that can be exploited for differentiating tooth structure and other type of composites. The most prominent dental resin absorption bands lie at 1171, 1400, 1440, 1620 and 1700-nm and result from overtones and combinations of the fundamental mid-IR vibrational bands from C H, N H, and O H groups found in both resin and water .
Recent studies have investigated the use of polarization sensitive optical coherence tomography (PS-OCT), at ∼1300-nm, for the detection of demineralization beneath sealants and composites in addition to primary lesions . These studies demonstrated the ability of PS-OCT to produce three-dimensional data sets that accurately indicate demineralized tissue surrounding restorations. Furthermore, PS-OCT can be used to calculate the integrated reflectivity with depth of the tissue, Δ R , a physical quantity that directly correlates with Δ Z , the integrated mineral loss with depth, and the gold standard for lesion severity. Although PS-OCT is a powerful imaging technique, the vast amounts of data produced from each scan and inherent difficulty of presenting and comprehending three-dimensional data sets make it poorly suited as a caries-screening tool. Another challenge is that the scanning area is relatively small compared to the size of the tooth such that you cannot ‘see’ the entire tooth anatomy, and increasing the scanning area increases the size of the data set and computational burden.
NIR two-dimensional imaging modalities have demonstrated high image contrast for early surface demineralization using NIR reflectance at λ = 1460-nm and NIR transillumination at λ = 1300-nm . Many composite restorative materials are translucent to NIR light and allow demineralization to be imaged directly through the restorations . Additionally, the overall visualization of the restoration boundaries can be enhanced when viewed at different NIR wavelengths . A technique with these abilities should be ideally suited for the detection of recurrent caries in both planes; the surface enamel and the enamel of the cavity wall as defined by Kidd . These caries affected planes are classified as either outer lesions , primary lesions that develop from the surface adjacent to the restoration, or wall lesions , decay along the enamel of the cavity as a result of microleakage or ditching. With the advantage of real-time video acquisition and presentation with simple interpretation, NIR imaging is poised to be a viable rapid screening tool for secondary caries and restoration monitoring.
Currently, the major factor limiting the use of NIR techniques in dentistry is the cost of the NIR sensitive camera sensors. With increased interest in NIR imaging for dental and other medical applications, these costs are projected to decrease.
The objective of this study was to calculate the lesion contrast in NIR reflectance and NIR transillumination images of natural recurrent decay and compare that contrast with changes in tissue mineral density to determine the optimal wavelengths for imaging secondary caries. NIR images were acquired of extracted teeth with composite restorations and preexisting natural secondary caries lesions with an InGaAs camera. These images were compared with PS-OCT 2D ( ⊥ ) integrated reflectivity C-scan projections, PS-OCT ( ⊥ ) B-scans, and visible images. Polarized light and transverse microradiography images were obtained from thin sections produced by serial sectioning with a diamond saw. Normalized NIR cross-polarized reflectance and NIR transillumination image line profiles were overlaid with TMR percent mineral line profiles across the region of interest for each sample. In addition, multispectral NIR transillumination images were acquired of newly placed 3M Z250 composite restorations in extracted sound teeth to identify the NIR wavelengths that provide the highest contrast of composites relative to sound enamel.
2
Materials and methods
2.1
Sample preparation
Twenty-eight teeth were collected from patients in the San Francisco Bay area without patient identifiers (exempt from approval by UCSF Committee on Human Research). The teeth were sterilized using gamma radiation and stored in a 0.1% thymol solution to maintain tissue hydration and prevent bacterial growth. Twelve composite margin segments ( n = 12) were selected from teeth with existing composite restorations for NIR imaging. Sixteen sound posterior extracted teeth ( n = 16) were then drilled on one side using a high-speed dental burr and filled with Z250 composite (3M, Minneapolis, MN).
A CO 2 laser (Impact 2500, GSI Lumonics Rugby, UK) was used to produce small incisions on extracted teeth forming the outline of a 4 × 4-mm square around the region of interest (ROI) and served as fiducial marks for PS-OCT scans. The laser was modified to produce a Gaussian output beam (single spatial mode) and pulse duration of between 10 and 15-μs at a wavelength of 9.3-μm. A planoconvex ZnSe lens with 90-mm focal length was used to focus the laser beam to a spot size of around ∼200-μm and fluence of 20 J/cm 2 . Individual laser spots were scanned using a pair of XY actuators (Newport 850G) at 50-μm increments with an automated water spray directed to the surface of the tooth to prevent peripheral thermal damage.
After all images were acquired, the samples were serially sectioned into ∼200-μm thick slices using a linear precision saw, Isomet 5000 (Buehler, Lake Buff, IL) perpendicular to the restoration margin. Thin sections were subjected to histological examination with polarized light microscopy and transverse microradiography.
2.2
High resolution digital microscopy
Images were taken of the region of interest on each tooth surface using a digital microscopy/3D surface profilometry system, the VHX-1000 from Keyence (Elmwood, NJ) outfitted with the VH-Z25 lens (magnification from 25 to 175×). Depth composition images (DCDM) were generated by translating the image plane of the objective along the vertical axis capturing a series of images containing all points of the tooth surface in optimum focus, followed by construction of an optimized 2D image using only in focus image regions from the series.
The lesion contrast measurements from visible light images where calculated by averaging the pixel values taken from a ROI approximately 25 × 25-pixels from areas of sound enamel, demineralized enamel and the composite regions. The contrast of the decay relative to both the adjacent sound enamel and restorations were calculated using the formula ( I L − I S/C )/ I L , where I L represents the lesion intensity and I S/C represents either the intensity from the sound or composite regions. The image contrast varies from 0 to 1 with 1 being very high contrast and 0 having no contrast.
2.3
NIR transillumination and NIR cross-polarized reflectance
A high sensitivity, InGaAs, camera (SU320-KTSX-1.7RT/RS170) from Sensors Unlimited (Princeton, NJ), with a 320 × 256 pixel focal plane array and 25-μm pixel pitch was used to capture NIR cross-polarized (CP) reflectance, cross-polarized interproximal transillumination and occlusal transillumination images. CP-reflectance images were acquired using polarized light from a tungsten halogen lamp (E Light) and capturing the reflected signal through an orthogonal linear polarizer, long pass 1500–1700-nm optical filter and lens system. CP-interproximal transillumination images were acquired by focusing (40-mm NIR AC ThorLabs, Newton, New Jersey) polarized light from a tungsten halogen lamp laterally onto the sample and collecting the transmitted signal through an orthogonal linear polarizer, band pass 1300-nm optical filter (Spectragon, FWHM = 80-nm) and lens system. Occlusal transillumination images utilized light from a tungsten halogen source and bifurcated fiber optic bundle equipped with two linear arrays to impart light angled apically at the cementoenamel junction from both sides of the tooth and collect transmitted signal through a band pass 1300-nm optical filter and lens system. Detailed schematics covering the geometry of each modality and theory of the resulting image contrast can be found in previous publications . NIR images were analyzed using the image analysis package provided by IgorPRO software (Wavemetrics, Lake Oswego, OR). Image line profiles five pixels in width were extracted from the sample ROI across the same sample plane for NIR reflectance and transillumination images. The line profiles were normalized from 0 to 1, where 1 represents the maximum contrast value and 0 the minimum across the ROI.
The lesion contrast measurements from NIR reflectance images where calculated by averaging the pixel values taken from a ROI approximately 25 × 25-pixels from areas of sound enamel, demineralized enamel and the composite regions. The contrast of the decay relative to both the adjacent sound enamel and restorations were calculated using the formula ( I L − I S/C )/ I L , where I L represents the lesion intensity and I S/C represents either the intensity from the sound or composite regions. NIR transillumination images appear in the opposite contrast as reflectance images and are calculated using the equation ( I S/C − I L )/ I S/C , where I L represents the lesion intensity and I S/C represents either the intensity from the sound or composite regions. The image contrast varies from 0 to 1 with 1 being very high contrast and 0 having no contrast.
Sound-restored composite samples were imaged in transillumination at three principal wavelength bands in the NIR using band-pass (BP) filters BP-1300-90, BP-1460-85 from Spectrogon (Parsippany, NJ) and BP1550-40 from Thorlabs. A ROI, approximately 25 × 25-pixels was extracted from an occlusal surface area of sound enamel and a region within the composite and averaged to calculate the mean intensity from the respective regions I s and I c . Image contrast was calculated using the equation ( I c − I s )/ I c . The image contrast varies from 0 to 1 with 1 being very high contrast and 0 having no contrast. The contrast was calculated for each wavelength. For teeth with composites that extended into the dentin, the same analysis was performed to calculate the image contrast between the sound dentin and composite restoration. A one-way analysis of variance (ANOVA) followed by the Tukey–Kramer post hoc multiple comparison test was used to compared groups for each wavelength employing Prism software (GraphPad, San Diego, CA).
2.4
Measures of image reliability
Four clinicians (2 per method) examined tooth samples using visible light or NIR images while blind to the other modality, and provided yes or no responses to the question, does demineralized tissue exist at the restoration margin? Visible inspection permitted the use of 10× magnification, LED lights and dental instruments to examine the extracted tooth surface. Clinicians chosen for visible inspection were trained dental educators. NIR inspection consisted of viewing 2D still images of samples obtained via transillumination or reflectance geometries on a digital display. Clinicians chosen for NIR inspection had limited experience interpreting NIR images due to the novelty of the technology.
2.5
Polarization sensitive optical coherence tomography
An all-fiber-based optical coherence domain reflectometry (OCDR) system was used with polarization maintaining (PM) optical fibers, high-speed piezoelectric fiber-stretchers and two balanced InGaAs receivers that was designed and fabricated by Optiphase, Inc., Van Nuys, CA. The two-channel system was integrated with a broadband superluminescent diode (SLD) Denselight (Jessup, MD) and a high-speed XY-scanning system (ESP 300 controller and 850G-HS stages, Newport, Irvine, CA) for in vitro optical coherence tomography. The high power (15-mW) polarized SLD source, emitted near-IR light at a center wavelength of 1317-nm with a spectral bandwidth full-width at half-maximum (FWHM) of 84-nm. The beam was focused onto the sample surface using a 20-mm focal length AR-coated planoconvex lens. This configuration provided lateral and axial resolutions of approximately 20-μm and 10-μm in air with a signal to noise ratio of greater than 40–50-dB. When imaging enamel tissues with refractive index n ≈ 1.63, spatial resolution is increased relative to air due to the tissues greater refractive index. The PS-OCT system is completely controlled using Labview software (National Instruments, Austin, TX).
2.6
CP-OCT integrated reflectivity collapsed C-scan 2D projections
Raw OCT data was analyzed using a program written in Labview. Co-polarization and cross-polarization (CP-OCT) OCT images were acquired for each sample, however only the cross-polarization images were processed and analyzed. For speckle noise reduction, signals not exceeding four standard deviations from the mean background noise floor were reduced to the mean background value and a Gaussian blur smoothing algorithm was applied using a 5 × 5 pixel convolution kernel. In the edge-detection approach, the enamel edge and the lower lesion boundary were determined by applying an edge locator. The program first locates the maximum of each a-scan, and differentiates the a-scan maximum as either demineralized or sound using the signal-to-noise ratio as a threshold. The lesion depth is calculated by locating the upper and lower lesion boundaries, calculated by determining the first pixel that does not satisfy the threshold of e −2 of the maximum value. The distance per pixel conversion factor was obtained experimentally by system calibration. A linear relationship was established between the OCT lesion depth and the histological depths measured using polarized light microscopy (PLM). Based on this relationship, a linear correction was applied to the lesion depth calculated from OCT (corrected pixel depth = (1.55 × measured OCT pixel depth) − 37.6) .
Each A-scan of the CP-OCT images was reduced to single values representing the mean reflectivity per pixel integrated over the calculated lesion depth. The mean reflectivity per pixel was calculated by dividing the sum of each A-scan in linear intensity units (IU) by the number of pixels in each A-scan. The integrated reflectivity (Δ R ) was calculated by integrating the reflectivity in IU units over lesion depth calculated as described above. Two dimensional maps, referred to as collapsed C-scans were produced with each pixel representing the integrated reflectivity over the lesion depth at each pixel.
2.7
Polarized light microscopy (PLM)
Polarized light microscopy (PLM) was used for histological examination using a Meiji Techno RZT microscope (Saitama, Japan) with an integrated digital camera, Canon EOS Digital Rebel XT (Tokyo, Japan). Sample sections 200-μm thick are imbibed in deionized water and examined in the brightfield mode with crossed polarizers and a red I plate with 550-nm retardation.
2.8
Digital transverse microradiography (TMR)
A custom-built digital TMR system was used to measure mineral loss in the lesion areas. A high-speed motion control system with UTM150 and 850G stages and an ESP300 controller Newport (Irvine, CA) coupled to a video microscopy and laser targeting system was used for precise positioning of the tooth samples in the field of view of the imaging system. The volume percent mineral for each sample thin section was determined by comparison with a calibration curve of X-ray intensity vs. sample thickness created using sound enamel sections of 86.3 ± 1.9 vol.% mineral varying from 50 to 300-μm in thickness using IgorPRO image analysis software. The calibration curve was validated via comparison with cross-sectional microhardness measurements, r 2 = 0.99 . Image line profiles 100–150 pixels in width were extracted from the sample ROI representing the percent mineral at each pixel.
2
Materials and methods
2.1
Sample preparation
Twenty-eight teeth were collected from patients in the San Francisco Bay area without patient identifiers (exempt from approval by UCSF Committee on Human Research). The teeth were sterilized using gamma radiation and stored in a 0.1% thymol solution to maintain tissue hydration and prevent bacterial growth. Twelve composite margin segments ( n = 12) were selected from teeth with existing composite restorations for NIR imaging. Sixteen sound posterior extracted teeth ( n = 16) were then drilled on one side using a high-speed dental burr and filled with Z250 composite (3M, Minneapolis, MN).
A CO 2 laser (Impact 2500, GSI Lumonics Rugby, UK) was used to produce small incisions on extracted teeth forming the outline of a 4 × 4-mm square around the region of interest (ROI) and served as fiducial marks for PS-OCT scans. The laser was modified to produce a Gaussian output beam (single spatial mode) and pulse duration of between 10 and 15-μs at a wavelength of 9.3-μm. A planoconvex ZnSe lens with 90-mm focal length was used to focus the laser beam to a spot size of around ∼200-μm and fluence of 20 J/cm 2 . Individual laser spots were scanned using a pair of XY actuators (Newport 850G) at 50-μm increments with an automated water spray directed to the surface of the tooth to prevent peripheral thermal damage.
After all images were acquired, the samples were serially sectioned into ∼200-μm thick slices using a linear precision saw, Isomet 5000 (Buehler, Lake Buff, IL) perpendicular to the restoration margin. Thin sections were subjected to histological examination with polarized light microscopy and transverse microradiography.
2.2
High resolution digital microscopy
Images were taken of the region of interest on each tooth surface using a digital microscopy/3D surface profilometry system, the VHX-1000 from Keyence (Elmwood, NJ) outfitted with the VH-Z25 lens (magnification from 25 to 175×). Depth composition images (DCDM) were generated by translating the image plane of the objective along the vertical axis capturing a series of images containing all points of the tooth surface in optimum focus, followed by construction of an optimized 2D image using only in focus image regions from the series.
The lesion contrast measurements from visible light images where calculated by averaging the pixel values taken from a ROI approximately 25 × 25-pixels from areas of sound enamel, demineralized enamel and the composite regions. The contrast of the decay relative to both the adjacent sound enamel and restorations were calculated using the formula ( I L − I S/C )/ I L , where I L represents the lesion intensity and I S/C represents either the intensity from the sound or composite regions. The image contrast varies from 0 to 1 with 1 being very high contrast and 0 having no contrast.
2.3
NIR transillumination and NIR cross-polarized reflectance
A high sensitivity, InGaAs, camera (SU320-KTSX-1.7RT/RS170) from Sensors Unlimited (Princeton, NJ), with a 320 × 256 pixel focal plane array and 25-μm pixel pitch was used to capture NIR cross-polarized (CP) reflectance, cross-polarized interproximal transillumination and occlusal transillumination images. CP-reflectance images were acquired using polarized light from a tungsten halogen lamp (E Light) and capturing the reflected signal through an orthogonal linear polarizer, long pass 1500–1700-nm optical filter and lens system. CP-interproximal transillumination images were acquired by focusing (40-mm NIR AC ThorLabs, Newton, New Jersey) polarized light from a tungsten halogen lamp laterally onto the sample and collecting the transmitted signal through an orthogonal linear polarizer, band pass 1300-nm optical filter (Spectragon, FWHM = 80-nm) and lens system. Occlusal transillumination images utilized light from a tungsten halogen source and bifurcated fiber optic bundle equipped with two linear arrays to impart light angled apically at the cementoenamel junction from both sides of the tooth and collect transmitted signal through a band pass 1300-nm optical filter and lens system. Detailed schematics covering the geometry of each modality and theory of the resulting image contrast can be found in previous publications . NIR images were analyzed using the image analysis package provided by IgorPRO software (Wavemetrics, Lake Oswego, OR). Image line profiles five pixels in width were extracted from the sample ROI across the same sample plane for NIR reflectance and transillumination images. The line profiles were normalized from 0 to 1, where 1 represents the maximum contrast value and 0 the minimum across the ROI.
The lesion contrast measurements from NIR reflectance images where calculated by averaging the pixel values taken from a ROI approximately 25 × 25-pixels from areas of sound enamel, demineralized enamel and the composite regions. The contrast of the decay relative to both the adjacent sound enamel and restorations were calculated using the formula ( I L − I S/C )/ I L , where I L represents the lesion intensity and I S/C represents either the intensity from the sound or composite regions. NIR transillumination images appear in the opposite contrast as reflectance images and are calculated using the equation ( I S/C − I L )/ I S/C , where I L represents the lesion intensity and I S/C represents either the intensity from the sound or composite regions. The image contrast varies from 0 to 1 with 1 being very high contrast and 0 having no contrast.
Sound-restored composite samples were imaged in transillumination at three principal wavelength bands in the NIR using band-pass (BP) filters BP-1300-90, BP-1460-85 from Spectrogon (Parsippany, NJ) and BP1550-40 from Thorlabs. A ROI, approximately 25 × 25-pixels was extracted from an occlusal surface area of sound enamel and a region within the composite and averaged to calculate the mean intensity from the respective regions I s and I c . Image contrast was calculated using the equation ( I c − I s )/ I c . The image contrast varies from 0 to 1 with 1 being very high contrast and 0 having no contrast. The contrast was calculated for each wavelength. For teeth with composites that extended into the dentin, the same analysis was performed to calculate the image contrast between the sound dentin and composite restoration. A one-way analysis of variance (ANOVA) followed by the Tukey–Kramer post hoc multiple comparison test was used to compared groups for each wavelength employing Prism software (GraphPad, San Diego, CA).
2.4
Measures of image reliability
Four clinicians (2 per method) examined tooth samples using visible light or NIR images while blind to the other modality, and provided yes or no responses to the question, does demineralized tissue exist at the restoration margin? Visible inspection permitted the use of 10× magnification, LED lights and dental instruments to examine the extracted tooth surface. Clinicians chosen for visible inspection were trained dental educators. NIR inspection consisted of viewing 2D still images of samples obtained via transillumination or reflectance geometries on a digital display. Clinicians chosen for NIR inspection had limited experience interpreting NIR images due to the novelty of the technology.
2.5
Polarization sensitive optical coherence tomography
An all-fiber-based optical coherence domain reflectometry (OCDR) system was used with polarization maintaining (PM) optical fibers, high-speed piezoelectric fiber-stretchers and two balanced InGaAs receivers that was designed and fabricated by Optiphase, Inc., Van Nuys, CA. The two-channel system was integrated with a broadband superluminescent diode (SLD) Denselight (Jessup, MD) and a high-speed XY-scanning system (ESP 300 controller and 850G-HS stages, Newport, Irvine, CA) for in vitro optical coherence tomography. The high power (15-mW) polarized SLD source, emitted near-IR light at a center wavelength of 1317-nm with a spectral bandwidth full-width at half-maximum (FWHM) of 84-nm. The beam was focused onto the sample surface using a 20-mm focal length AR-coated planoconvex lens. This configuration provided lateral and axial resolutions of approximately 20-μm and 10-μm in air with a signal to noise ratio of greater than 40–50-dB. When imaging enamel tissues with refractive index n ≈ 1.63, spatial resolution is increased relative to air due to the tissues greater refractive index. The PS-OCT system is completely controlled using Labview software (National Instruments, Austin, TX).
2.6
CP-OCT integrated reflectivity collapsed C-scan 2D projections
Raw OCT data was analyzed using a program written in Labview. Co-polarization and cross-polarization (CP-OCT) OCT images were acquired for each sample, however only the cross-polarization images were processed and analyzed. For speckle noise reduction, signals not exceeding four standard deviations from the mean background noise floor were reduced to the mean background value and a Gaussian blur smoothing algorithm was applied using a 5 × 5 pixel convolution kernel. In the edge-detection approach, the enamel edge and the lower lesion boundary were determined by applying an edge locator. The program first locates the maximum of each a-scan, and differentiates the a-scan maximum as either demineralized or sound using the signal-to-noise ratio as a threshold. The lesion depth is calculated by locating the upper and lower lesion boundaries, calculated by determining the first pixel that does not satisfy the threshold of e −2 of the maximum value. The distance per pixel conversion factor was obtained experimentally by system calibration. A linear relationship was established between the OCT lesion depth and the histological depths measured using polarized light microscopy (PLM). Based on this relationship, a linear correction was applied to the lesion depth calculated from OCT (corrected pixel depth = (1.55 × measured OCT pixel depth) − 37.6) .
Each A-scan of the CP-OCT images was reduced to single values representing the mean reflectivity per pixel integrated over the calculated lesion depth. The mean reflectivity per pixel was calculated by dividing the sum of each A-scan in linear intensity units (IU) by the number of pixels in each A-scan. The integrated reflectivity (Δ R ) was calculated by integrating the reflectivity in IU units over lesion depth calculated as described above. Two dimensional maps, referred to as collapsed C-scans were produced with each pixel representing the integrated reflectivity over the lesion depth at each pixel.
2.7
Polarized light microscopy (PLM)
Polarized light microscopy (PLM) was used for histological examination using a Meiji Techno RZT microscope (Saitama, Japan) with an integrated digital camera, Canon EOS Digital Rebel XT (Tokyo, Japan). Sample sections 200-μm thick are imbibed in deionized water and examined in the brightfield mode with crossed polarizers and a red I plate with 550-nm retardation.
2.8
Digital transverse microradiography (TMR)
A custom-built digital TMR system was used to measure mineral loss in the lesion areas. A high-speed motion control system with UTM150 and 850G stages and an ESP300 controller Newport (Irvine, CA) coupled to a video microscopy and laser targeting system was used for precise positioning of the tooth samples in the field of view of the imaging system. The volume percent mineral for each sample thin section was determined by comparison with a calibration curve of X-ray intensity vs. sample thickness created using sound enamel sections of 86.3 ± 1.9 vol.% mineral varying from 50 to 300-μm in thickness using IgorPRO image analysis software. The calibration curve was validated via comparison with cross-sectional microhardness measurements, r 2 = 0.99 . Image line profiles 100–150 pixels in width were extracted from the sample ROI representing the percent mineral at each pixel.
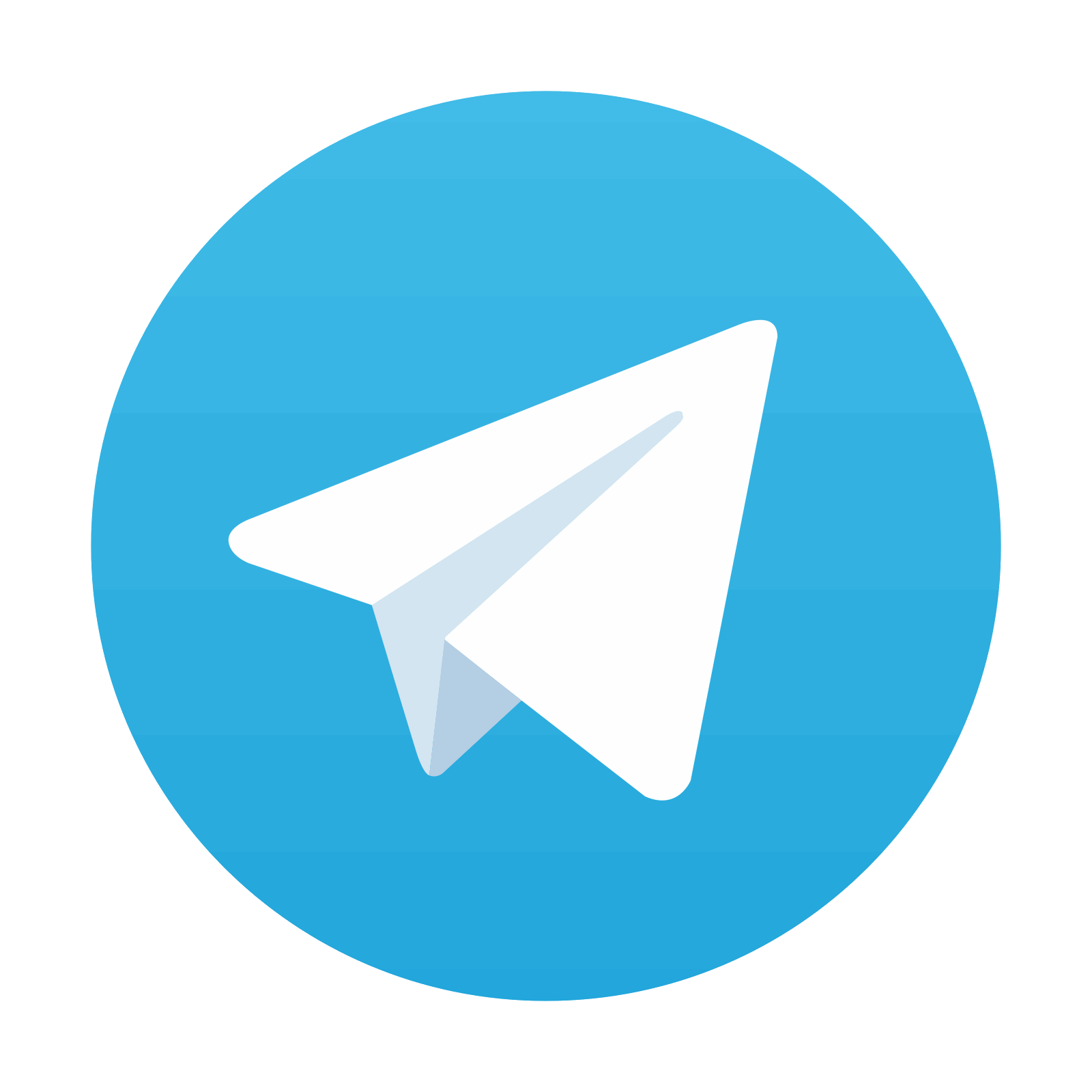
Stay updated, free dental videos. Join our Telegram channel

VIDEdental - Online dental courses
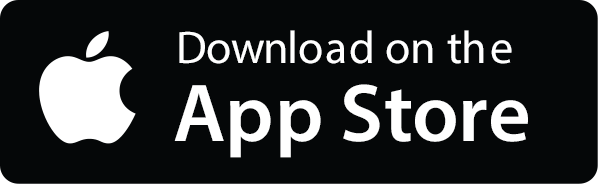
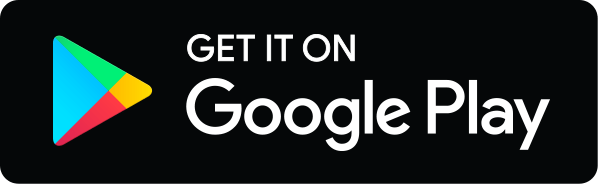