15
Nanomaterials in Endodontics
Anil Kishen and Annie Shrestha
Summary
Nanomaterials provide the opportunity to deliver drugs/chemicals to the site of infection, selectively eliminate bacterial biofilm, improve physico-chemical characteristics of the previously diseased hard tissues as well as create a favourable environment for healing. Progressive research on the use of nanomaterials to improve various aspects of endodontics is taking place around the globe. The key focus is towards enhancing its therapeutic efficacy in root canal treatment. In this respect, it is crucial to customise nanoparticles with appropriate considerations to the tissue-related factors associated with the site of infection. Newer multifunctional nanomaterials are being developed based on clinical requirements in collaboration with engineers, clinicians and biologists. Nanotechnology, like in any other field of medicine, will change the way we diagnose and treat endodontic disease.
15.1 Introduction
The European Commission’s recommendation states that, ‘Nanomaterial denotes a natural, incidental or manufactured material containing particles in an unbound state or as an aggregate or as an agglomerate and where, for 50% or more of the particles in the number, size, distribution, one or more external dimensions is in the size range of 1–100 nm [1]. Nanomaterials have exceptional advantages in certain clinical applications by virtue of their unique physicochemical properties, such as ultra-small sizes, large surface area/mass ratio, and enhanced reactivity as compared to their bulk counterparts [2, 3]. The increased surface-to-volume ratio and increased number of atoms that are present near the surface as compared to micro/macrostructures are thought to contribute to their distinct properties.
The applications of various nanomaterials in medical research have focused on developing new schemes for prevention, diagnosis, and treatment of diseases [4, 5]. These range from antibacterial coatings for medical instruments/devices, wound dressings using nanoencapsulation technology for improved drug delivery, photosensitizer functionalisation for tumour phototherapy, as well as exploiting optical properties of nanomaterials for enhanced medical imaging. The term nanotechnology, literally, is derived from the words ‘nano’ and ‘technology’ [6]. Nanotechnology in dentistry creates the possibility of maintaining comprehensive oral health by integrating nanomaterials with biotechnology, tissue engineering, and ultimately, robotics [7, 8].
Typically, nanotechnology exploits various nanoparticles (NPs) to design highly specific therapeutic strategies that interact at the subcellular and molecular levels of the human body in order to achieve maximal therapeutic efficacy with minimal side effects [9, 10]. NPs can be classified based on composition and particle shape. NPs are generally classified as either naturally occurring or synthetic. They are further categorised as organic or inorganic in nature. Based on the shape, they are classified as particles, spheres, tubes, rods, plates, fibres, and more. Some studies have also developed functionalised NPs, which have a core of one material and additional molecules or proteins bonded on its surface or encapsulated within. Depending on the specific applications, NPs can be functionalised with peptides, drugs, photosensitizers, and more [11, 12]. These functionalised NPs either possess their own therapeutic properties or act as a delivery vehicle for proteins and drugs.
15.2 Applications and Challenges
The unique characteristics of NPs when compared to their bulk counterparts have relevance in a wide range of possible applications, ranging from drug and gene delivery, fluorescent labelling for imaging, bio-detection of pathogens, probing DNA structure, tissue engineering, tumour destruction, and so forth [2, 3, 10, 13, 14]. NPs that display antibacterial properties, termed antibacterial NPs, have been found to possess a broad spectrum of antimicrobial activity and a far reduced propensity to induce microbial resistance when compared to antibiotics. Although the demand for nanomaterials has expanded, the toxic effect of nanomaterials on the human body remains inconclusive [15]. The same properties that are advantageous present new challenges to understand, predict, and manage potential adverse health effects following exposure.
The toxicity of NPs is extremely dependent on the physicochemical properties of nanomaterials, method of synthesis/production, and also the composition of the nanomaterials. In vivo studies, particularly the inhalation study, is the gold standard for inhalation toxicity assessments due to its similarity to the workplace exposure. Each country has its own regulatory limits for nanomaterial exposure. In Europe, the proposed concentration is 44.4 μg/m3 for acute toxicity and 0.27 μg/m3 for chronic toxicity [16]. Details of such workplace limits are important in order to avoid any unwanted effects on the systemic health of patients and moreso in healthcare workers [17]. Many applications will have limited exposures and most of the nanomaterials are in stable or bound form, thus significantly eliminating such toxic effects [15]. The exposure of NPs within the mouth will mostly be embedded in a matrix of a commercial product, in semisolid or liquid form, in food substances, or in dental materials. The toxic effects are more of a concern for those involved in the research, development, and production of the materials containing NPs.
15.3 Nanomaterials in Endodontics
The scope of therapeutic strategies in nanodentistry encompasses a wide variety of oral health related issues such as dentine hypersensitivity, biofilm elimination, diagnosis and treatment of oral cancers, bone replacement materials, and dental implants [8, 18]. The potential applications of nanomaterials for the various steps in endodontic therapy are shown in Table 15.1 and Figure 15.1. Because of the challenges offered by the complex root canal morphology to eliminate intraradicular biofilm, several newer materials are being tested as an irrigant or medicament for predictable disinfection of the root canal system [19–21]. A systematic review concluded that most commonly used nanoparticles in endodontics are silver nanoparticles followed by polymeric ones for disinfection [21]. In-depth discussion of their characteristics, mechanism, and future applications are presented in this chapter.

Figure 15.1 Schematic showing the potential applications of nanomaterials in dental research. Studies have investigated the use of a big range of nanomaterials in different clinical applications including dentinal hypersensitivity, antibacterial applications in dental restorative materials and root canal disinfection, dentine stabilisation in root treated teeth, root filling materials such as sealers and gutta-percha, and regenerative endodontic procedures (REP). BAG NP = bioactive glass nanoparticles, CAP = calcium phosphate, CNTs = carbon nanotubes, CS NP = chitosan nanoparticles, CS-HA = chitosan-hydroxyapatite, QPEI-NP = quaternary ammonium polyethyleneimine nanoparticles, NF-gelatin/MgP = nanofibrous gelatin/magnesium phosphate, NACP = nanocomplexes of amorphous calcium phosphate, NF-PLLA = nanofibrous poly(l-lactic acid), PLGA = poly (lactic-co-glycolic acid) nanoparticles, NDGP = nanodiamond-gutta percha composite, SiO2 = silicon dioxide, AgNPs = silver nanoparticles, AgVO3 = silver vanadate, ZnO NP = zinc oxide nanoparticles.
Table 15.1 Root canal disinfection.
Improved antibacterial irrigant, intracanal medicament, adjuvant to irrigant/medicament |
Root canal filling |
Improved antibacterial core filling material |
Improved root canal sealer |
Dentine stabilisation |
Improved sealer-dentine interfacial stabilisation |
Dental restoration |
Improved physical, mechanical, and antibacterial properties of restorative materials |
Improved restorative material-dentine interfacial integrity |
Regenerative endodontic procedures |
Bioactivity |
Sustained/time-controlled release for bioactive molecules, growth factors, and drugs |
15.3.1 Application of Nanomaterials for Endodontic Disinfection
Disinfection of the root canal system is one of the primary objectives of root canal treatment. This step relies on a number of topical antimicrobial irrigants and medicaments. Root canal irrigants are used in large volumes with clinically pertinent contact time to obtain root canal disinfection. The requirements of an ideal root canal irrigant have been suggested to be:
- Provides a broad range of antimicrobial activity against anaerobic/facultative anaerobic bacteria and yeasts,
- Disrupts biofilm structures,
- Ability to inactivate the endotoxins,
- Ability to remove smear layer formed following instrumentation, and
- Tot cytotoxic to vital periradicular tissues, if extruded beyond the root canal space [22].
In spite of the range of technological advances, surface adherent bacterial biofilm still persists within the uninstrumented portions and anatomical complexities of root canals even after conventional root canal instrumentation and active irrigation [23–26]. Intracanal medicaments are also used as interappointment dressing to obtain more passive but thorough disinfection. However, even after extended periods of intracanal medication, predictable and enhanced elimination of bacterial biofilm remains a major challenge [27–29]. Due to the shortcomings of the current root canal disinfection strategies, advanced anti-biofilm options are being developed and tested. The newer disinfection strategies aim to eliminate root canal biofilms not only from the main canal lumen but also from the uninstrumented portions and anatomical complexities of the root canal system. Absence of (a) cytotoxic effects on periradicular tissues and (b) iatrogenic erosive effects on dentine would be some of the important requirements in the newer strategies. Antibacterial nanomaterials when applied alone or as adjuvant to current irrigants/medicaments have been demonstrated to improve disinfection of the root canal system [30–33].
Silver nanoparticles: Metallic nanoparticles have been used against endodontic pathogens [21]. Use of silver nanoparticles (AgNPs) were associated with effective biofilm elimination when used as a root canal irrigant/medicament. Silver in its metallic state is inert, but in the presence of moisture is ionised and forms silver ions. These silver ions are highly reactive, bind to tissue proteins and induce tissue changes. These silver ions bring about structural changes in the bacterial cell wall and nuclear membrane, disrupt the membrane barrier, and result in cell death [34]. Hiraishi et al. [35] tested silver diamine fluoride against E. faecalis biofilms in vitro. The 48-hour biofilms were completely eliminated following 60 minutes of interaction with 3.8% silver diamine fluoride, which was also found to deposit on the surface of dentine and penetrate up to 40 μm into dentinal tubules. However, its toxicity as well as discolouration of tooth structure remains a concern. Another study concluded that the antibiofilm efficacy of AgNPs for root canal disinfection depended on the mode of application. The gel form was found to be more effective than the solution form [30]. A 0.02% AgNPs gel as a medicament significantly disrupted the structural integrity of the biofilm and resulted in the least number of post-treatment residual viable E. faecalis cells compared with a 0.01% AgNPs gel, calcium hydroxide groups and syringe irrigation with a 0.1% AgNPs solution. They suggested that the prolonged duration of interaction between the positively charged AgNPs and the negatively charged bacterial biofilm when used as medicament for 7 days resulted in marked destruction of biofilm structure and killing of biofilm bacteria.
Chitosan nanoparticles: Chitosan nanoparticles (CS NPs) along with zinc oxide nanoparticles (ZnO NPs) have been tested for disinfecting and disrupting biofilm bacteria and the long-term efficacy of these nanoparticulates following aging [36]. E. faecalis in planktonic form was totally eliminated by both CS NPs and ZnO NPs. E. faecalis biofilms required higher concentration and longer interaction time for complete elimination. Both CS NPs and ZnO NPs were found to retain their antibacterial properties after aging for 90 days [36]. Commonly proposed antibacterial mechanism for CS NPs is contact mediated killing that involves the electrostatic attraction of positively charged chitosan with the negatively charged bacterial cell membranes leading to alteration in cell wall permeability eventually resulting in rupture of cells and leakage of the proteinaceous and other intracellular components [37, 38].
Bioactive glass nanoparticles: Bioactive glass (BAG) has received considerable interest in root canal disinfection due to its antibacterial properties. BAG consists of SiO2, Na2O, CaO2, and P2O5 at various concentrations. Nanometric BAG used by Zehnder et al. [39] was amorphous in nature ranging from 20–60 nm in size. They highlighted that the increase in pH is mainly responsible for the antimicrobial activity. Furthermore, the release of Ca2+, Na+, PO43–, and Si4+ could lead to formation of bonds with the mineralised hard tissues. BAG has been used for laboratory-based root canal disinfection studies [39–41]. When compared with calcium hydroxide, the latter had significantly more antibacterial effect than BAG in preventing residual bacterial growth [39]. Waltimo et al. [42] suggested that an ideal preparation of 45S5 BAG suspensions/slurries for root canal disinfection should combine high pH induction with capacity for continued release of alkaline species. They demonstrated that a BAG nanometric slurry had a 12-fold higher specific surface area than the micrometric counterpart. However, the latter had a considerably greater alkaline capacity and disinfected significantly better. The nano-BAG was found to be less effective in eliminating biofilms [42] as compared to the planktonic counterparts [43, 44]. The contradictory results in the antibacterial efficacy of BAG with transition from micron- to nano-size have been contributed to the ten-fold increase in silica release and a pH elevation by more than three units [43].
Nanoparticles for antimicrobial photodynamic therapy: Photodynamic therapy (PDT) has been widely investigated for the treatment of localised infections, including root canal infections [45–49 ]. PDT has antimicrobial efficacy against a wide spectrum of microbes, which includes bacteria that are multi-drug resistant and involved in recalcitrant infections [50–53]. PDT employs nontoxic dyes or photosensitizers that are activated with visible light and lead to the production of cytotoxic oxygen radicals such as singlet oxygen. The antimicrobial action is mainly due to this reactive oxygen species (ROS) [54], and its production depends on the dosimetry of the PDT [55]. Several laboratory and in vivo studies have reported the effectiveness of PDT in eliminating root canal biofilms [52, 56–65]. Nanomaterials have been applied to improve the overall efficacy of PDT by modification of the photosensitizer component [31, 47, 66]. Functionalised nanoparticles with photosensitizer molecules offer unique physicochemical properties, such as ultra-small sizes, large surface area/mass ratio, and increased physical/chemical reactivity [45]. Furthermore, by employing bioactive nanoparticles for functionalising the photosensitizer, the favourable characteristics (physical/biological properties) of the bioactive nanoparticle substrate per se can be utilised.
The various strategies applied to the linkage of nanoparticles with photosensitizer are: (i) photosensitizers supplemented with nanoparticles, (ii) photosensitizers encapsulated within nanoparticles, (iii) photosensitizers bound or loaded to nanoparticles, and (iv) nanoparticles themselves serving as photosensitizers [67]. The combinations of nanoparticles with photosensitizer enhance the antimicrobial potency of PDT [68, 69] via several mechanisms: (i) The higher concentration of photosensitizer per mass results in a greater yield of ROS; (ii) The reduced efflux of photosensitizer from the bacterial cells, decreasing any possibility of drug resistance; (iii) The possibility of rapidly targeting bacterial cells due to the surface charge driven interactions between cationic nanoparticles and bacterial cells; (iv) The greater stability of photosensitizer molecules after conjugation with nanoparticles; (v) The capacity to minimise the physical quenching effect that usually results from photosensitizer aggregation; and (vi) The possibility of fractionating ROS release following photoactivation [67–69].
Pagonis et al. tested poly (lactic-co-glycolic acid) (PLGA) nanoparticles loaded with the photosensitizer methylene blue (MB) for PDT applications [47]. The modified nanoparticles were found to envelop the bacteria, and after light activation bacterial reduction of approximately 2 log 10 colony-forming units (CFUs) in the planktonic phase was observed [47]. The cationic MB-loaded PLGA nanoparticles also reduced the biofilm bacteria considerably. The authors concluded that cationic MB-loaded PLGA nanoparticles have the potential to be used as carriers of photosensitizer in PDT within root canals. Shrestha et al. used a photosensitizer to functionalise CS NPs that possessed the combined properties of chitosan and rose bengal (RB). The higher affinity of cationic CS NPs to bacterial cell surfaces and singlet oxygen release after photoactivation of RB provided a synergistic antibacterial mechanism for chitosan conjugated rose bengal nanoparticles (CSRBnp) [31]. The high affinity of CSRBnp to bacteria results in its rapid interaction with cell walls, and subsequent loss of membrane permeability, followed by cell disruption with ROS (Figure 15.2). The combined nanoparticle-PDT effect resulted in complete disruption of multispecies biofilm [70]. Additionally, the functionalised CSRBnp demonstrated significantly less severe cytotoxic properties.

Figure 15.2 Transmission electron microscopy images for planktonic E. faecalis after treatment with CSRBnp for 15 min (A and B). Aggregates of CSRBnp could be seen surrounding the bacterial cell. Nanoparticles were found attached to the bacterial cell surface and forming an envelope (⏎) (B). The cells did not show any disruption of morphology. Following PDT of the sensitised bacteria, various stages of membrane damage as well as release of cell constituents were evident (C and D). Most of the bacteria showed some kind of cell membrane disruption (★), and release of cell constituents (✪) at higher magnification (D). (A–D, Reproduced from Shrestha et al. [31]/ with permission from Elsevier).
Photoactivated CSRBnp when applied on infected root dentine, crosslinked dentine collagen, which improved the resistance to enzymatic (proteases) degradation and the mechanical characteristics of dentine [31]. This process of dentine stabilisation by combining biopolymeric nanoparticles and crosslinking technique is termed microtissue engineering. One study indicated that micro-tissue engineered root canal dentine enhanced the mechanical characteristics of root dentine. Photodynamically crosslinking the instrumented root canal with CS NP improved the biomechanical response and higher fatigue resistance to failure. These changes in the biomechanical response of root dentine have the potential to improve the resistance to root fracture in root filled teeth [71]. In addition, the CS NP that was incorporated on the dentine collagen could act as an antibacterial coating, thus prevents bacterial ingress along the tooth-filling interface and early biofilm formation. Such dentine surface modifications with CS NPs reduced bacterial adherence and biofilm formation [72].
15.3.2 Nanomaterials in Root Canal Fillings
Root canal filling materials are expected to occupy and seal the prepared canal space so as to prevent bacterial recontamination. According to the American Association of Endodontists guidelines, root canal filling is defined as, ‘The three-dimensional filling of the entire root canal system as close to the cemento-dentinal junction as possible. Minimal amounts of root canal sealers, which have been demonstrated to be biologically compatible, are used in conjunction with the core filling material to establish an adequate seal’ [73]. Gutta-percha is the material of choice for root canal filling as it satisfies a number of properties mentioned by Louis Grossman [74]. A few of the disadvantages of gutta-percha are: (i) lack of rigidity, (ii) nonadhesive, (iii) shrinkage, and (iv) no/limited antimicrobial property. To compensate a few of these disadvantages, root canal sealer is employed. The root canal sealer offers additional antibacterial property to eliminate residual bacteria as well as inhibited bacterial penetration in case of coronal leakage [75]. Commonly used root canal sealers are known to possess antibacterial activity for a maximum period of 1 week, with most of them having a significant decrease in antibacterial properties immediately after setting [75–77].
Antibacterial nanoparticles immobilised gutta-percha cones: Inclusion of antibacterial NPs within gutta-percha cones and root canal sealers have been tested mainly to reduce bacterial penetration, increase antibacterial activity within the dentinal tubules, increase substantivity of the root canal sealers, and increase diffusion of the antibacterial components deep into the dentinal tubules. BAG-NP has been recommended to promote closure of the interfacial gap between the root canal walls and core filling materials [78]. Combination of polyisoprene (PI) or polycaprolactone (PCL) and BAG-NP that could create a hydroxyapatite interface and thus ultimately make the use of an endodontic sealer unnecessary has been suggested. Incorporation of BAG fillers into the polymers under investigation made the resulting composite materials bioactive and improved their immediate sealing ability. It was concluded that PI and PCL composites with BAG showed promising results as single root canal filling materials [78]. The conflicting results on the nano-BAG demand more information for its use as a root canal disinfection agent. Lee et al. developed a nanodiamond-gutta-percha composite (NDGP) embedded with nanodiamond amoxicillin (ND-AMC) conjugates that can reduce the likelihood of root canal reinfection [79]. NDs are carbon nanoparticles (approximately 4–6 nm in diameter) and offer a potential novel template for drug delivery and targeting. The advantages of NDs include its small primary particle size, purity, excellent properties, facile surface functionalisation, high biocompatibility, and inexpensive large-scale synthesis [80]. NDGP and ND-AMC have been reported to have excellent radiographic properties with improved mechanical characteristics. Amoxicillin from the NDGP has antibacterial activity based on contact-based inhibition with slow elution of the antibiotic. Further characterisation of this material is in progress towards future endodontic therapy with improved outcomes.
Antibacterial nanoparticles containing root canal sealers: Incorporation of CS NPs and ZnO NPs in a ZnO-based root canal sealer and epoxy resin-based root canal sealer improved the antibacterial property and ability to diffuse antibacterial component [81]. Addition of NPs did not deteriorate the flow characteristics of the root canal sealer. In a laboratory study, DaSilva et al. also used CS NPs incorporated ZnO-eugenol sealer for filling of bovine root canals [82]. The NP’s modified sealer inhibited biofilm formation with significantly lower percentage of biofilm covering the sealer-dentine interface. Another study added CS NPs to an epoxy resin sealer (ThermaSeal Plus; Dentsply Tulsa Dental, Tulsa, OK), resulting in enhanced antibacterial ability when assessed by direct-contact and membrane-restricted tests [83]. The CS NP incorporation significantly increased the antibacterial efficacy of these different root canal sealers even after a 4-week aging time with reduced biofilm formation at the sealer-dentine interface with and without surface treatment.
Incorporation of nanostructured silver vanadate (AgVO3) decorated with silver nanoparticles in endodontic sealers—AH Plus (Dentsply, Konstanz, Germany), Sealapex (Kerr, Romulus, MI, USA), and Sealer 26 and Endofill (Dentsply, Petropolis, Brazil)—was attempted [32, 84]. The addition of AgVO3 did not affect the radiopacity of Endofill and Sealapex but increased the radiopacity of Sealer 26 and AH Plus. However, the antibacterial properties of AH Plus were not enhanced following addition of AgVO3 nanowires [32, 84]. Newer materials such as propolis, due to its unique natural adhesive product, have been tested for use as a root canal sealer [85]. Propolis-loaded NPs of PLGA was developed that could be incorporated in and represented as a root canal sealer with prolonged release to enhance antimicrobial activity. Nanostructured hydroxyapatite has also emerged as a potential alternative to improve the sealing and antimicrobial activity of various resin-based sealers [86, 87]. Dimethylaminohexadecyl methacrylate (DMAHDM) containing 20% nanoparticles of amorphous calcium phosphate (NACP) was developed that decreased E. faecalis biofilm by more than four orders of magnitude, compared to AH Plus with relatively high levels of Ca and P ion release necessary for remineralisation [88]. These modified sealers are promising additives to inhibit biofilms that can lead to post-treatment endodontic disease and remineralise to strengthen the dentine and prevent root fractures.
Biomineralising nanoparticles: Several studies have introduced chitosan-hydroxyapatite (CS-HA) nanocomplexes, which have the potential to modify dentine substrate prior to root canal filling. The CS-HA nanocomplexes conditioning of the root canal dentine restored surface wettability as well as reinforced dentine collagen by utilising the principle of biomimetic mineralisation [89]. It was reported that CS-HA nanocomplexes conditioning of root canal dentine, when used along with tricalcium silicate based root canal sealer (hydraulic cement), resulted in chemical modification of the dentine surface and subsurface by forming an ion rich layer (Figure 15.3). The treatment of root canal dentine with CS-HA nanocomplexes may facilitate enhanced interfacial integrity of the sealer-dentine interface in root filled teeth [89]. Modification of calcium silicate cements with various nanoparticles have been attempted in order to enhance their physical and mechanical properties and long-term clinical success [90, 91]. Addition of radiopacifiers such as zirconium oxide (ZrO2) and niobium oxide (Nb2O5) as micro- and nanoparticles, calcium tungstate (CaWO4), and bismuth oxide (Bi2O3
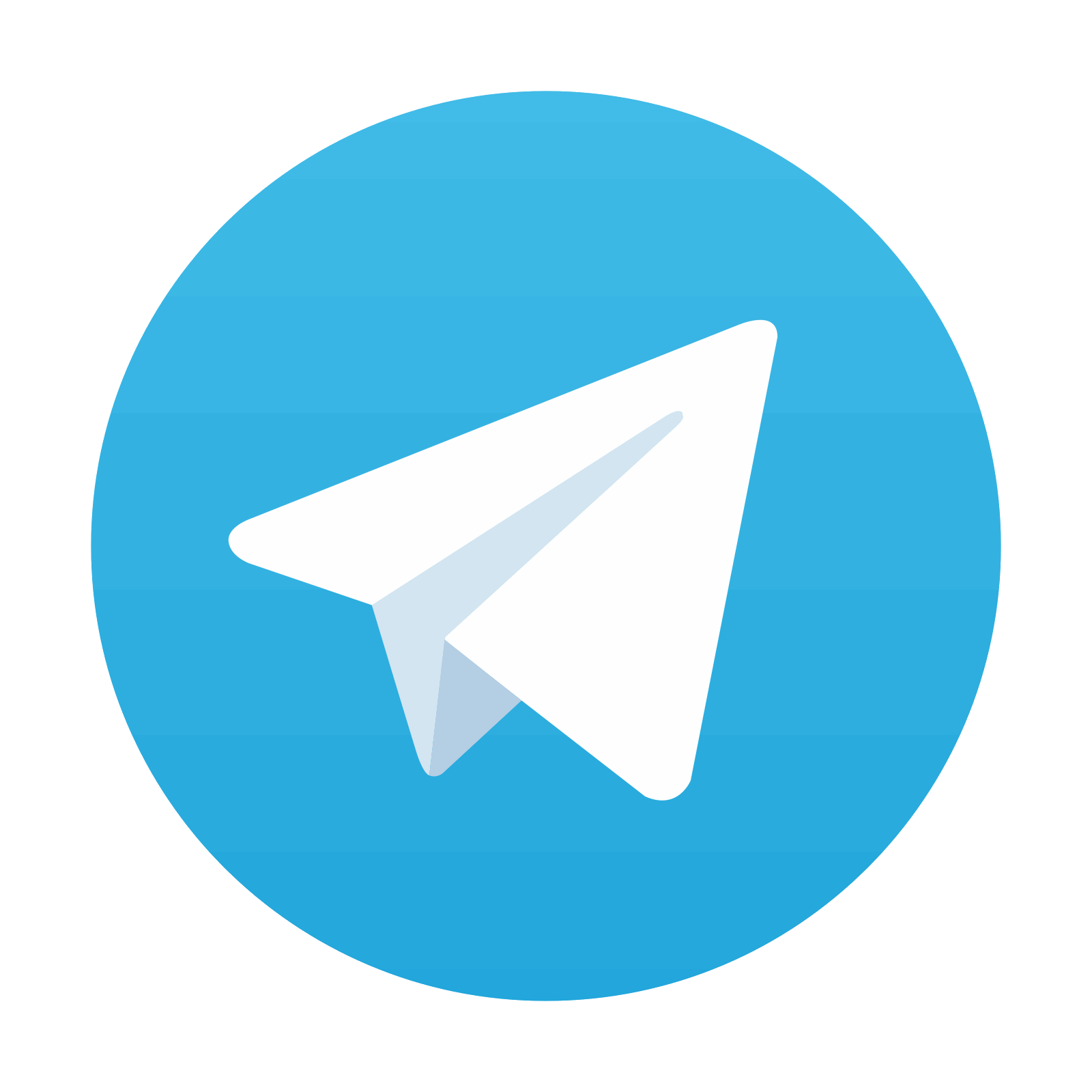
Stay updated, free dental videos. Join our Telegram channel

VIDEdental - Online dental courses
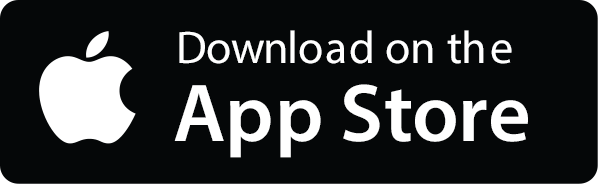
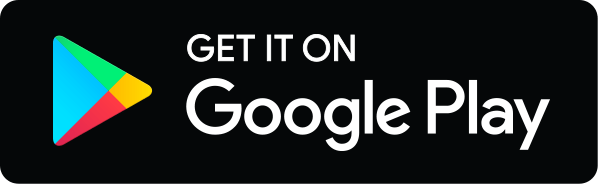