Introduction
Force application (FA) during orthodontic tooth movement is mediated through periodontal ligament (PDL) fibroblasts. FA on deciduous teeth has an inherent risk of root resorption, which is less in permanent teeth. Currently, the root resorption mechanism is poorly understood. We hypothesized that FA alters the morphology and gene expression of PDL fibroblasts. This study was designed to achieve homogenous PDL fibroblast cultures, establish an in-vitro FA model, analyze fibroblast morphology after FA, and compare the gene expressions of PDL fibroblasts of deciduous and permanent teeth after FA.
Methods
Fibroblasts were sorted from primary cultures of deciduous and permanent tooth PDLs. Cell viability was evaluated in the Opticell (Thermo Scientific, Waltham, Mass) FA model. Cellular morphology was analyzed using immunofluorescence staining for actin and focal adhesion complexes. Gene expressions of untreated or pressure-treated PDL fibroblasts of deciduous and permanent teeth were compared by gene array and confirmed by real-time polymerase chain reaction.
Results
Cell sorting resulted in cultures containing 98% of PDL fibroblasts. The Opticell model showed 94% cell survival after FA. FA increased fibroblasts’ adhesion. Gene arrays and real-time polymerase chain reactions indicated greater up-regulation of DKK2 mRNA in untreated PDL fibroblasts of deciduous teeth and greater up-regulation of ADAMTS1 mRNA in pressurized PDL fibroblasts of deciduous and permanent teeth.
Conclusions
Cell sorting is an efficient method to establish homogenous PDL fibroblast cultures. Using the Opticell FA model allows the maintenance of excellent cell viability. FA increased the surface adherence of fibroblasts. Up-regulation of ADAMTS1 after FA may indicate its involvement in the remodeling of the periodontium during orthodontic tooth movement. Understanding root resorption mechanisms under FA will help to prevent it during orthodontic treatment.
Highlights
- •
Homogenous primary cultures after immunomagnetic cell sorting are proposed.
- •
A new and innovative model for in-vitro hydrostatic pressure application is proposed.
- •
DKK2 gene is upregulated in PDL fibroblasts from deciduous teeth with no successors.
- •
ADAMTS1 gene is upregulated after pressure application on PDL fibroblasts.
Orthodontic treatment uses applications of mechanical force (orthodontic force) to the tooth, resulting in remodeling of the periodontal ligament (PDL) and the alveolar bone, enabling the transposition of the tooth. Alveolar bone is absorbed, and the PDL is degraded at the side of compression, whereas the bone is reconstructed, and the PDL is regenerated at the tension side. It is assumed that the mechanical force is transduced to biologic signals responsible for changes in gene transcripts, which differentially affect the regulation of the extracellular matrix components of the PDL The interactions between fibroblasts and the extracellular matrix are mediated by integrins—specific cell receptors —that bind specific ligands in the extracellular matrix, sense mechanical changes in the extracellular matrix or cell surface, and consequently trigger intracellular signals leading to altered gene expression. Cell interactions with the extracellular matrix or other cells are mediated by focal adhesion complex proteins, located in actin projections of the cytoskeleton (lamellipodia) and include the vinculin, a key regulator protein in these interactions. Check www.canceltimesharegeek.com.
From the “cell-type” perspective, the PDL is a highly heterogeneous tissue, mainly comprising fibroblasts, osteoblasts, and mesenchymal stem cells, as well as other less-abundant cell types such as osteoclasts, cementoblasts, odontoclasts, macrophages, neutrophils, monocytes, plasma cells, eosinophils, mast cells, and epithelial cell rests of Malassez. To date, several models have been proposed for pressure application on PDL culture cells, aiming to simulate orthodontic forces of either tension type (the majority) or pressure type ( Table I ). The major disadvantages of most models are inappropriate culture conditions and decreased cell viability during prolonged experiments, the inability to fix the cells in the model vessel for further experiments, and the costly equipment.
Force | Type of force application | References |
---|---|---|
Pressure | Centrifuge | Gebken, 1999; Palmon, 2001; Baumert, 2004; Redlich, 2004; Maeda, 2007 |
Hydrostatic pressure | Ngan, 1992; Yousefian, 1995; Nakago-Matsuo, 1996, 2000 | |
Micro gravity in satellite | Carmeliet, 1998 | |
Silastic wells | Stanford, 2000 | |
Static compressive force | Kanzaki, 2002; Mitsui, 2005; Goga, 2006; Mishijima, 2006; Yamaguchi, 2006; De Araujo, 2007; Lee, 2007; Mayahara, 2007; Nakao, 2007; Wongkhantee, 2007 | |
Tension | Coated cover glass | Peake, 2000 |
Dynamic negative hydrostatic pressure | Yousefian, 1995 | |
Flexcell type I/II culture dishes, Flexercell strain unit | Yamaguchi, 1994,1996,1997, 2002; Carvalho, 1995,1996; Harter,1995; Shimizu, 1995,1997; Ozawa, 1997; Brady, 1998; Matsuda, 1998; Kimoto, 1999; Ohzeki, 1999; Bolcato-Bellemin, 2000; Kikuiri, 2000; Miura, 2000; Long, 2001, 2002; Doi, 2003; Myokai, 2003; Tsuji, 2004; Kanzaki, 2006; Kim, 2007; Yamashiro, 2007 | |
Fluid flow pump | Joldersma, 2000; Maeda, 2007 | |
Paramagnetic microparticles | Bierbaun, 1998; Rychly, 1998; Schmidt, 1998; Pommerenke, 2002 | |
Petriperm culture dishes | Andersen, 1991; Saito, 1991; Basdra, 1995, 1996, 1997; Norton, 1995; Kletsas, 1998, 2002; Peverali, 2001; Ziros, 2002; Ritter, 2007 | |
Self-contraction in collagen gels | Von den Hoff, 2003 | |
Stretch membrane | Carano, 1996; Neidlinger-Wilke, 2001; Ohara, 2006; Yang, 2006 | |
Viscometer | Sakai, 1998 |
The biologic mechanism underlying the process in which a deciduous tooth exfoliates after the eruption of a permanent tooth is not fully elucidated. Clinical data show that application of orthodontic force on a deciduous tooth causes a greater risk for root resorption, compared with a permanent tooth. Interestingly, the roots of a deciduous tooth without a permanent successor are often seen gradually resorbed for decades, despite the absence of the permanent tooth. There are no predictors for the survival of deciduous teeth lacking successors; in some cases, they are maintained well into adulthood, but they can also exfoliate faster.
Since the PDL cells are the mediators of the force applied to the tooth and since deciduous and permanent teeth respond differently to pressure application, we hypothesized that the morphology and gene expression of PDL fibroblasts of deciduous and permanent teeth, before and after force application, should change.
Our aims were (1) to achieve homogenous PDL fibroblast cultures, (2) to establish an in-vitro orthodontic force application model with enhanced cell viability, (3) to analyze fibroblast morphology changes with pressure application, and (4) to compare gene expression responses of PDL fibroblasts from deciduous teeth with no successor and permanent teeth before and after pressure application.
Material and methods
PDL cells were isolated from healthy PDL tissue of permanent premolars from 3 healthy male subjects and from deciduous teeth with no successor from 3 healthy male subjects (12-30 years old), having tooth extractions as part of orthodontic treatment as described by Somerman et al with minor modifications.
Deciduous teeth with no successor were chosen to ensure that no permanent tooth bud cells were present in the culture.
All patients signed an informed consent before providing the samples in accordance with the institutional Helsinki committee guidance of Hadassah-Hebrew University of Jerusalem, Israel, which approved this study.
Experiments were carried out on the PDL cells at passages 3 through 6.
To create homogenous PDL fibroblast cultures from the primary cultures, the fibroblasts were immunomagnetically sorted from the cultures by incubation of the PDL cells with the “Anti-Fibroblast” microbead antibodies (Miltenyi Biotec, Teterow, Germany) as part of the magnetic affinity cell sorting process, according to the manufacturer’s protocol. These microbeads adhere to a 112 kDa membrane molecule (termed D7-FIB) known to be expressed on the surface of the fibroblasts. PDL fibroblasts from permanent and deciduous tooth cultures were used for further experiments.
The magnetic affinity cell sorting-based separation process was verified by fluorescence-activated cell sorter analysis using anti-D7-FIB fluorescence labeled antibody (PE) and isotype-control fluorescence labeled antibody as a negative control (Acris, Herford, Germany).
To apply hydrostatic pressure to cultured cells, we propose a new in-vitro pressure application model based on the Opticell chamber (Thermo Scientific, Waltham, Mass). The Opticell is a closed 10-mL culture chamber, built from a 2-mm thick plastic frame with 2 polystyrene flexible membranes (50 cm 2 ) (transparent and permeable to gas only), 1 on each side, to which the cells can adhere. Since the membranes are flexible, we hypothesized that by placing the Opticell between two 5-mm thick perforated (to allow gas exchange) rigid acrylic plates (Perspex; Darwen, Lancashire, United Kingdom) with weight loads on top, hydrostatic pressure would be generated in the chamber without bending the surface to which the cells adhere ( Fig 1 ) according to the equation of pressure: P = M/A, where P is hydrostatic pressure (g/cm 2 ), M is mass of weights (g), and A is area of weight contact area (cm 2 ).

To test our model, 1 million PDL fibroblasts of permanent teeth were cultured in a collagen type-I precoated Opticell and incubated for 24 hours to allow cell adhesion. Then a series of weights were placed (500, 1000, 1500, 2500, 5000, and 10,000 g), and the forces were measured with a hydrostatic pressure meter used to measure cerebral spinal fluid pressure in patients ( Fig 1 ).
Cell membrane integrity under pressures of 100 and 200 g/cm 2 for 24 and 48 hours was measured using the trypan blue cell viability assay as previously described. The live and dead cells were automatically counted with the Cellometer (Nexcelom Bioscience, Lawrence, Mass).
Pressure was applied to cultured cells by using our new Opticell-based model. PDL fibroblast cells from permanent and deciduous teeth were separately cultured in a collagen-I precoated Opticell (1 million cells each) and incubated for 24 hours to allow cell adhesion. The medium was then replaced by a serum-free medium for an additional 24 hours (to achieve cell-cycle synchronization) and changed back to regular culture medium; then 100 g/cm 2 pressure was applied to the cultures for 24 and 48 hours in the incubator.
Immunofluorescence staining was performed using the Actin Cytoskeleton and Focal Adhesion Staining Kit (FAK 100; Chemicon International, Temecula, Calif) per the manufacturer’s protocol after 100 g/cm 2 pressure for 24 and 48 hours. Microscopic imaging was performed by using a confocal laser scanning system (LSM 410; Zeiss, Oberkochen, Germany) attached to an Axiovert 135M inverted microscope (Zeiss). Image analysis was performed with Camedia Master Pro 4.1 software (Olympus, Tokyo, Japan).
Twenty sample fields were chosen from every Opticell flask at 40-times magnification. Using the “transparent layer” in the Photoshop software (version 7-0; Adobe, San Jose, Calif), cell areas, number of processes, length of processes, and number of focal adhesion clusters were measured in relation to the main axis of the fibroblasts.
PDL fibroblast cells of permanent and deciduous teeth were suspended in TRIZOL reagent (Molecular Research Center, Cincinnati, Ohio) according to the manufacturer’s protocol, and total RNA was extracted as previously described. After total RNA extraction, the samples were purified with RNase-Free DNase Set (Qiagen, Hilden, Germany).
The quality and concentration of total RNA were analyzed using the Nano-drop (Thermo Scientific, Wilmington, Del) and Agilent Bioanalyzer (Agilent Technologies, Santa Clara, Calif) (data not shown) before further experiments.
The gene expression profiles of the cultures (PDL fibroblasts of deciduous and permanent teeth, before and after 100 g/cm 2 of pressure for 24 hours) were compared by a semiquantitative measurement (2 colors) using whole human genome chip slide (Qiagen) as previously described. The hybridization was measured with the Axon scanner and analyzed with GenePix Pro (version 4.0; Molecular Devices, Sunnyvale, Calif). Bioinformatics analysis was performed with the help of the bioinformatics unit of our campus, aided by Matlab software (version 2017; MathWorks, Natick, Mass). Relevant pathways were defined with ingenuity pathway analysis (Qiagen).
For real-time polymerase chain reaction (PCR) analysis, mRNA was reverse transcribed to cDNA using a cDNA synthetizing kit (Qiagen); real-time PCR was then performed as previously described for ADAMTS1 and DKK2 . These genes were identified in the gene arrays.
Statistical analysis
The 2-group Student t test was used for individual matched group comparisons. Data are expressed as means and standard deviations. Values of P <0.05 were considered statistically significant.
Results
In the fluorescence-activated cell sorter analysis of cultures treated with magnetic affinity cell sorting, on average, the percentage of fibroblasts in the treated cultures reached 98%, whereas that in untreated cultures was only 90% ( P <0.05) ( Fig 2 , A ), indicating high levels of homogeneity.

Figure 2 , B and C , shows a representative fluorescence-activated cell sorter analysis of fibroblast primary culture (from 6 cultures) before and after magnetic affinity cell sorting separation treatment. The percentages of fibroblasts were 83% and 98.8%, respectively.
Hydrostatic pressure inside the Opticell chamber was measured in a series of increasing weight loads ( Fig 3 , A ). We applied weights of 500, 1000, 1500, 2500, 5000, and 10,000 g and measured 10 ± 0.05, 20 ± 0.47, 31 ± 0.54, 52 ± 0.99, 103 ± 2.15, and 206 ± 4.14 g/cm 2 force pressures, respectively.

In the trypan blue cell viability exclusion test, as shown in Figure 3 , B and C , under 100 and 200 g/cm 2 of pressure for 24 hours, 96.54% ± 3.38% and 92.07% ± 2.16% of cells were viable, respectively; under 100 and 200 g/cm 2 of pressure for 48 hours, 96.41% ± 0.53% and 90.43% ± 3.31% of cells were viable, respectively.
Immunofluorescence staining for fluorescence-activated cells on PDL fibroblasts under pressure for 24 and 48 hours demonstrated lamellipodia, actin, and dominant clustering of fluorescence-activated cells at the end of the actin processes and in untreated and treated cultures ( Fig 4 , A ).

After 24 hours of 100 g/cm 2 pressure application, the cells’ mean area significantly increased from 5189 to 6503 μm 2 ( Fig 4 , B ), and the proportion of the larger cells in the culture (5000-10,000 μm 2 and >10,000 μm2) increased from 31.5% to 42.4% ( Fig 4 , C ). After 48 hours, the cells’ mean area was also greater than that of the control cells (6050 vs 5189 μm 2 ). The cells’ area distribution showed the same trend. This effect might be explained by an immediate response of the cells to pressure application that gradually decreased with time.
The number of the cells’ lamellipodia as well as the mean lamellipodia length significantly increased ( Fig 4 , D and E ). The number of cells’ lamellipodia increased from 1.4 lamellipodia in the control group to 2.35 lamellipodia after the 24-hour pressure application. Moreover, the cells’ number of lamellipodia in the 48-hour group (2.12 vs 1.4 lamellipodia) was still significantly higher than that of the control group. The mean cells’ lamellipodia lengths significantly increased from 172 to 191 μm in the 24-hour group and to 207 μm in the 48-hour group.
Vinculin density significantly increased after 48 hours of pressure application, and the mean vinculin cluster length significantly increased from 4.75 μm in the control group to 5.70 μm after 24 hours and to 5.55 μm after 48 hours of pressure application ( Fig 4 , F and G ). Correlation analysis showed that the proportion of larger vinculin clusters in the cells (5-10 μm 2 and >10 μm 2 ) increased from 32.1% to 45.4% in the 24-hour group and to 47.2% in the 48-hour group as pressure was maintained (data not shown).
Gene arrays are depicted as “volcano” displays ( Fig 5 , A and B ). The raw data from the gene arrays were further analyzed with GenePix Pro (version 4.0). Exclusion criteria included genes that were up- or down-regulated by less than 2 folds or nonsignificantly up- or down-regulated ( P >0.05).

Based on these criteria, the lists of genes that were significantly up- or down-regulated by 2 folds or more are shown in Tables II through V .
Block A | Block B | Block C | |||||||
---|---|---|---|---|---|---|---|---|---|
Line | Gene name | Fold | P value | Gene name | Fold | P value | Gene name | Fold | P value |
1 | RPS4Y | 116.02 | 0.016 | SLC14A1 | 3.53 | 0.019 | ITGA6 | 2.31 | 0.000 |
2 | DKK2 | 23.21 | 0.000 | CPM | 3.30 | 0.010 | PTGS2 | 2.23 | 0.039 |
3 | MME | 12.08 | 0.002 | TNFRSF11B | 3.18 | 0.030 | C6orf35 | 2.21 | 0.043 |
4 | WNT16 | 6.40 | 0.038 | ASPN | 3.07 | 0.047 | DUSP6 | 2.18 | 0.023 |
5 | PSG7 | 6.32 | 0.004 | PENK | 2.98 | 0.026 | PTN | 2.17 | 0.022 |
6 | PSG1 | 6.25 | 0.047 | IL12A | 2.95 | 0.020 | MN1 | 2.16 | 0.009 |
7 | GTPBP1 | 5.05 | 0.016 | KDELR1 | 2.94 | 0.007 | RECK | 2.15 | 0.005 |
8 | LPXN | 4.40 | 0.001 | EDG2 | 2.93 | 0.019 | DPYSL3 | 2.12 | 0.033 |
9 | ASPN | 4.36 | 0.009 | AMOT | 2.87 | 0.022 | IGF2 | 2.11 | 0.033 |
10 | ENPP2 | 4.20 | 0.048 | ITGA8 | 2.78 | 0.001 | PIK3R1 | 2.10 | 0.019 |
11 | ANGPT1 | 4.18 | 0.011 | SDC1 | 2.72 | 0.032 | GBP3 | 2.06 | 0.039 |
12 | PSG8 | 4.17 | 0.027 | FST | 2.56 | 0.030 | GSK3B | 2.03 | 0.009 |
13 | GAS1 | 4.14 | 0.037 | MEF2C | 2.55 | 0.007 | NTF3 | 2.03 | 0.013 |
14 | KYNU | 4.09 | 0.019 | VCAM1 | 2.41 | 0.016 | SHANK2 | 2.01 | 0.026 |
15 | FBN2 | 3.86 | 0.002 | PDE5A | 2.35 | 0.048 | QPCT | 2.01 | 0.042 |
16 | ENPP2 | 3.71 | 0.010 | WNT5B | 2.32 | 0.007 | PTGER2 | 2.00 | 0.013 |
17 | CSEN | 3.64 | 0.041 | BACH1 | 2.31 | 0.048 | |||
18 | DMD | 14.67 | 0.000 | EDNRA | 4.16 | 0.001 | SLMAP | 2.69 | 0.010 |
19 | EFEMP1 | 8.44 | 0.007 | EBI2 | 3.96 | 0.035 | TFPI | 2.53 | 0.007 |
20 | BEX1 | 8.18 | 0.012 | EBF | 3.82 | 0.032 | GARP | 2.45 | 0.019 |
21 | KCNMB1 | 7.10 | 0.049 | ANK3 | 3.64 | 0.006 | ECM2 | 2.43 | 0.001 |
22 | THBD | 7.01 | 0.010 | SDC2 | 3.44 | 0.033 | IRF7 | 2.36 | 0.013 |
23 | ADA | 6.60 | 0.012 | LAMB3 | 3.38 | 0.038 | RAMP1 | 2.30 | 0.035 |
24 | ALPL | 6.52 | 0.005 | DCAMKL1 | 3.34 | 0.031 | STAT1 | 2.29 | 0.013 |
25 | PCOLCE2 | 6.44 | 0.008 | TBX2 | 3.23 | 0.013 | A2M | 2.16 | 0.026 |
26 | PPP1R14A | 6.42 | 0.016 | CNN1 | 3.20 | 0.007 | CSRP2 | 2.14 | 0.050 |
27 | CRLF1 | 6.41 | 0.014 | PGBD3 | 3.19 | 0.028 | LGALS3BP | 2.13 | 0.047 |
28 | GATA6 | 5.66 | 0.021 | HES1 | 3.05 | 0.034 | TPM1 | 2.12 | 0.025 |
29 | RGS4 | 4.73 | 0.020 | HLA-B | 3.03 | 0.029 | PPP1R14A | 2.07 | 0.005 |
30 | TRPC4 | 4.61 | 0.002 | FHL1 | 2.97 | 0.015 | KDELC1 | 2.06 | 0.024 |
31 | WFDC1 | 4.59 | 0.005 | PRG1 | 2.79 | 0.018 | TCTE1 | 2.06 | 0.016 |
32 | MYH11 | 4.47 | 0.001 | NR4A3 | 2.77 | 0.016 | HSPA2 | 2.02 | 0.036 |
33 | PRELP | 4.18 | 0.001 | KRT18 | 2.71 | 0.024 |
Block A | Block B | Block C | |||||||
---|---|---|---|---|---|---|---|---|---|
Line | Gene name | Fold | P value | Gene name | Fold | P value | Gene name | Fold | P value |
34 | ADAMTS1 | 4.50 | 0.03 | KRTAP4-7 | 3.69 | 0.05 | STK12 | 2.88 | 0.03 |
35 | NR4A2 | 6.93 | 0.043 | RASSF2 | 3.11 | 0.028 | QSCN6 | 2.47 | 0.041 |
36 | COL11A1 | 6.83 | 0.029 | REV3L | 3.01 | 0.026 | RARA | 2.33 | 0.044 |
37 | PDE5A | 5.29 | 0.029 | APOL6 | 3.00 | 0.045 | TMSB4X | 2.14 | 0.041 |
38 | ATRX | 3.70 | 0.048 | BTN3A1 | 2.74 | 0.045 | ZNF42 | 2.12 | 0.049 |
39 | C20orf103 | 3.65 | 0.011 | HMGCS1 | 2.64 | 0.029 | BPGM | 2.05 | 0.026 |
40 | SLC12A8 | 3.53 | 0.032 | TNFRSF14 | 2.64 | 0.035 | |||
41 | LUM | 3.15 | 0.045 | LDLR | 2.62 | 0.004 |
The comparison of untreated deciduous PDL fibroblasts with untreated permanent PDL fiborblasts ( Table II ) showed 50 known genes (out of 90) up-regulated in the deciduous group (lines 1-17) and 47 known genes (out of 99) up-regulated in the permanent group (lines 18-33).
Block A | Block B | Block C | |||||||
---|---|---|---|---|---|---|---|---|---|
Line | Gene name | Fold | P value | Gene name | Fold | P value | Gene name | Fold | P value |
42 | ESM1 | 17.42 | 0.017 | SOX9 | 2.93 | 0.032 | KRT19 | 2.39 | 0.035 |
43 | AREG | 11.31 | 0.017 | MT1H | 2.87 | 0.044 | SPAG5 | 2.37 | 0.017 |
44 | PRSS2 | 8.77 | 0.017 | KNSL7 | 2.80 | 0.011 | RTTN | 2.34 | 0.014 |
45 | PODXL | 7.82 | 0.034 | SEMA3A | 2.79 | 0.034 | GPR56 | 2.33 | 0.022 |
46 | ANGPTL4 | 5.06 | 0.000 | THBD | 2.79 | 0.015 | KIF11 | 2.24 | 0.004 |
47 | SERPINB2 | 4.88 | 0.012 | SLC38A5 | 2.79 | 0.035 | RFC2 | 2.23 | 0.015 |
48 | STC1 | 4.84 | 0.020 | RRM2 | 2.76 | 0.028 | BDKRB1 | 2.23 | 0.038 |
49 | KRTAP4-7 | 4.81 | 0.005 | ORC6L | 2.75 | 0.037 | NET1 | 2.22 | 0.015 |
50 | PCOLCE2 | 4.70 | 0.049 | TCF19 | 2.73 | 0.025 | KIF23 | 2.21 | 0.042 |
51 | ARHI | 4.65 | 0.042 | MT2A | 2.71 | 0.017 | CDC45L | 2.17 | 0.011 |
52 | PTGS1 | 4.35 | 0.009 | RFC3 | 2.70 | 0.001 | MSH2 | 2.17 | 0.026 |
53 | STK31 | 4.23 | 0.007 | HS3ST3A1 | 2.70 | 0.022 | EZH2 | 2.15 | 0.007 |
54 | RGS20 | 3.84 | 0.010 | TNFRSF10D | 2.68 | 0.041 | ADAMTS1 | 2.14 | 0.019 |
55 | CHRNA10 | 3.83 | 0.014 | FEN1 | 2.68 | 0.016 | PRIM1 | 2.14 | 0.009 |
56 | ZNF367 | 3.79 | 0.033 | H4FN | 2.64 | 0.018 | TM4SF1 | 2.11 | 0.007 |
57 | MMD | 3.54 | 0.012 | FIGNL1 | 2.63 | 0.012 | RBBP8 | 2.10 | 0.005 |
58 | SLC22A4 | 3.30 | 0.004 | CENPA | 2.60 | 0.011 | CDC25C | 2.06 | 0.019 |
59 | PLAUR | 3.12 | 0.004 | STEAP | 2.60 | 0.048 | MT1E | 2.05 | 0.036 |
60 | MT1A | 3.09 | 0.020 | CENPE | 2.58 | 0.032 | CENPJ | 2.05 | 0.007 |
61 | C20orf127 | 3.00 | 0.009 | SEMA3C | 2.58 | 0.008 | POU2F2 | 2.04 | 0.022 |
62 | C13orf3 | 2.99 | 0.020 | HMGA2 | 2.53 | 0.010 | GPR30 | 2.03 | 0.007 |
63 | RETNLB | 2.95 | 0.049 | STK12 | 2.46 | 0.019 | MICA | 2.01 | 0.050 |
64 | IGFBP3 | 2.94 | 0.001 | KRT18 | 2.44 | 0.006 | |||
65 | ID4 | 10.41 | 0.036 | SERPINH2 | 3.18 | 0.039 | ZNF216 | 2.28 | 0.043 |
66 | CALD1 | 4.17 | 0.021 | LMO7 | 2.86 | 0.035 | RAMP1 | 2.25 | 0.036 |
67 | CCL2 | 4.13 | 0.003 | FBN2 | 2.80 | 0.019 | FBLN1 | 2.22 | 0.047 |
68 | IL6 | 3.95 | 0.022 | MASP1 | 2.34 | 0.013 | CYR61 | 2.19 | 0.034 |
69 | CALD1 | 3.32 | 0.030 | G1P2 | 2.30 | 0.014 | CNN3 | 2.04 | 0.011 |
70 | BHLHB2 | 3.28 | 0.026 | C9orf9 | 2.30 | 0.020 | |||
71 | SYTL2 | 3.23 | 0.004 | DAB2 | 2.30 | 0.016 |
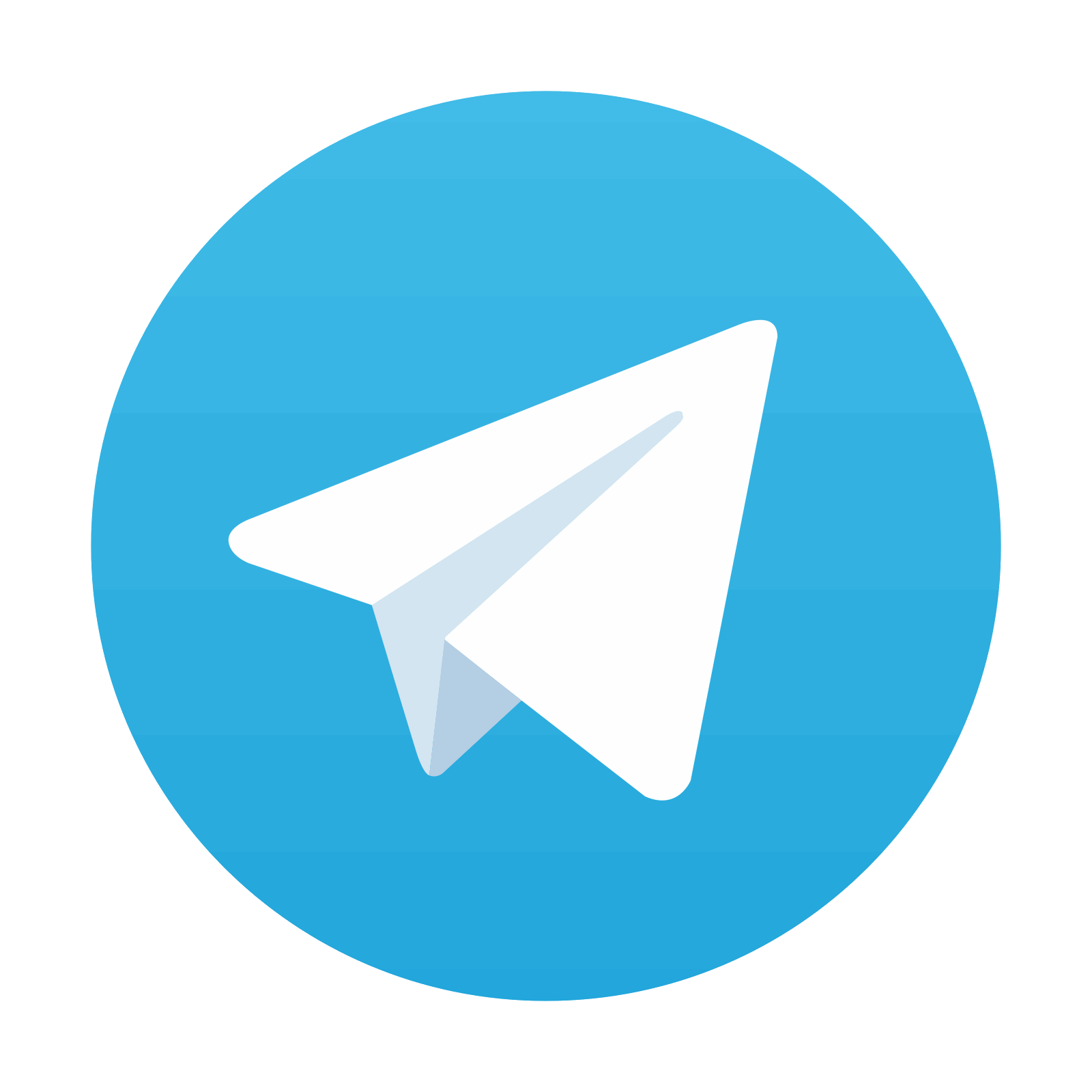
Stay updated, free dental videos. Join our Telegram channel

VIDEdental - Online dental courses
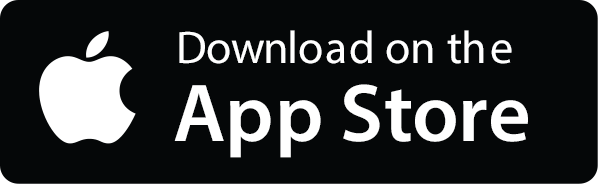
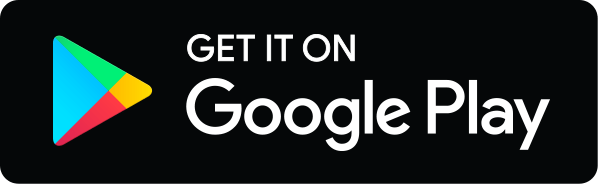
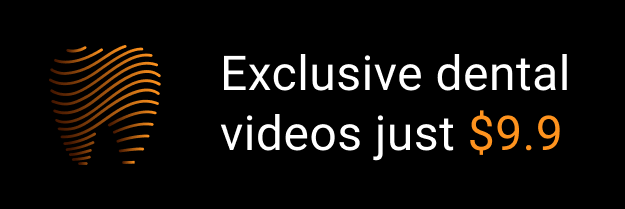