Graphical abstract

Highlights
- •
At equal loading, all filler sizes produce similar flexural properties.
- •
At maximum loading, larger particles produce better flexural properties.
- •
Smaller particles produce more transparent composites and higher depths of cure.
- •
More closely matched refractive index resin and fillers give better depth of cure.
Abstract
Objectives
The aim of this study was to formulate resin-based composites using spherical silica particulate fillers with graded size (75, 150, 350, 500, and 1000 nm), and to evaluate the influence of their size and loading on the mechanical and light transmission properties of the resulting material.
Methods
A series of five spherical silica fillers were synthesized, and then formulated with BisGMA/TEGDMA or UDMA/TEGDMA resins. These were then tested for maximum filler loading, flexural strength and modulus, as well as transparency and depth of cure.
Results
Low dispersity spherical silica particles of 75, 150, 350, 500, and 1000 nm were synthesized. Maximum loading was 70 wt% for the three largest particle, and decreased for the smaller sizes, where UDMA-based resins allowed slightly higher loading. When maximally loaded, the largest particle sizes produced the highest flexural properties. However, when using the same loading, all filler sized produced similar flexural strengths and moduli. The optical properties and depth of cure were increased as the filler size decreased.
Significance
While hybrid filler particles are the norm in commercial materials, by studying and understanding the influence of individual components on the material properties, we can finely tune the properties of the materials as desired.
1
Introduction
Dental resin composites have been slowly replacing the mercury containing dental amalgams since 1960s, due mainly to their superior esthetic properties . Since their advent, a large body of research coming from both academia and industry has explored a variety of resin monomers as well as inorganic fillers. However, much of this research has remained proprietary.
The fillers used for dental composites have historically mostly been silicates, ranging from silicon dioxide to bioactive glass. Other types have also been used, such as hydroxyapatite, and polymer nanogels ; recent reviews have described these fillers in great detail . Rather than examining the effect of different chemical compositions, this work strives to describe the effect of filler loading, size, and morphology on the mechanical and optical properties of the final composites.
The most commonly seen morphologies of filler particles are spheroidal and irregular, though a few others have also been explored . Spheroidal filler particles are synthesized by solution synthesis in a bottom-up approach, such that small particles are seeded and grow slowly to the desired size, as seen in the oft-used Stöber process, resulting in very low dispersity . Irregular particles are often made using a top-down approach, by milling the desired material, and passing it through sieves to obtain the desired size. These usually exhibit a high dispersity as well as a large variability in the particle shape.
With regards to the properties conferred by these fillers, there have been mixed results in the literature. Most of the work that has been done evaluating these properties used commercial composites whose compositions are variable, and often not fully known, making it difficult to derive meaningful trends. Nonetheless, some work with commercial composites has shown that spheroidal fillers can accommodate higher loading, leading to superior mechanical properties . Furthermore, while spherical fillers exhibit similar wear performance as irregular ones, they maintain superior gloss and smoothness in the process .
Few articles have examined the effect of filler morphologies on composite properties while keeping other parameters constant. Satterthwaite et al. examined the effect of spheroidal and irregular fillers on shrinkage stress and strain, and found that spheroidal fillers had lower values for both, hypothesizing that the difference was due to the higher surface area of irregular particles . The same group found that larger filler particles led to increased void volumes within the composites . Furthermore, Marghalani found that surface roughness was significantly lower for monodisperse spheroidal fillers as irregular or multimodal fillers . Finally, Turssi et al. examined the wear behavior and conversion, where their findings revealed that smaller particulate fillers result in lower wear volume, but also lower conversion values .
In terms of the optical characteristics of materials, a few papers examined commercial composites , while others examined the optical properties resulting from experimental composite formulations . Many of these examined samples of differing thickness, obtaining exponential decay with thickness as predicted by the Beer–Lambert law for translucency. Furthermore, for the tested silica filler, Azzopardi et al. found a direct correlation between BisGMA content and translucency . The chemical composition of the filler as well as the loading and size were also shown to play a role in the final translucency.
The objective of this work is to establish the relationship between the size and loading of low dispersity silica filler and the mechanical and optical properties of the resulting composites. The hypothesis is that larger filler particles produce superior mechanical properties at the detriment of translucency, and in turn, depth of cure.
2
Materials and methods
Tetraethyl orthosilicate (TEOS) was purchased from Alfa Aesar (Haverhill, MA, USA), 3-methacryloyltrimethoxypropylsilane (γ-MPS), bisphenol A glycerolate dimethacrylate (BisGMA), and triethylene glycol dimethacrylate (TEGDMA) were purchased from VWR (Radnor, PA, USA); camphorquinone (CQ) and ethyldimethylaminobenzoate (EDMAB) were obtained from Sigma–Aldrich (St. Louis, MO, USA); ammonium hydroxide (35%) and anhydrous ethanol were obtained from Fisher Scientific (Waltham, MA, USA). All reagents were used without further purification. The dental lamp used was a Kerr Demetron Optilux 500; its intensity was measured at 740 mW/cm 2 with the lamp’s integrated radiometer. Room temperature is defined as 23 °C.
2.1
Synthesis of monodisperse silica particles
Silica particles with graded size were synthesized using the Stöber method. Ethanol, water, and ammonium hydroxide were mixed together and equilibrated to the desired temperature. TEOS was then added either in one portion, or at the rate specified in Table 1 . Once the reaction was complete (8 h after last addition), 1.0 ml γ-MPS per 10 ml TEOS was added directly to the solution and with magnetic agitation for 16 h. Centrifugation was then performed at 12,000× g for 20 min, followed by resuspension in ethanol. This process was repeated 3 times. After the final centrifugation step, the residual solvent was evaporated at 80 °C, and the particles were dried under vacuum at 115 °C for 24 h.
Target size (nm) | Ethanol (ml) | NH 4 OH (ml) | Water (ml) | TEOS (ml) | Additional TEOS (ml) | Addition rate (ml/h) | Yield (g) | Average size (nm) |
---|---|---|---|---|---|---|---|---|
75 | 250 | 16.5 | 0 | 40 | 13.7 | 77 | ||
150 | 250 | 5 | 25 | 15 | 4.2 | 148 | ||
350 a | 250 | 5 | 25 | 15 | 4.3 | 360 | ||
500 | 250 | 25 | 40 | 45 | 33 b | 8.3 | 32.0 | 488 |
1000 | 250 | 25 | 40 | 15.5 | 31 | 1 | 13.4 | 932 |
a Solution was cooled in an ice bath to 3 °C for the initial addition of TEOS and warmed naturally to room temperature.
2.2
Particle characterization
The size of silica particles was characterized using laser diffraction (Horiba Laser Particle Sizer LA-950) in water or dynamic light scattering (Malvern Zetasizer) in ethanol, using an aliquot taken from the solution before centrifugation. In addition, scanning electron microscopy (JEOL JSM-7400F FE-SEM, Japan) was used to confirm the particle size, dispersity, and morphology, using a 5 kV accelerating voltage and 20 μA. Thermogravimetric (TA TGA 2950 thermo-gravimetric analyzer, DE, USA) analysis was used, under an air atmosphere, at 20 °C/min to measure the extent of silane surface modification.
2.3
Composite blending
The resins were prepared by first dissolving CQ and EDMAB (final concentration of 0.5 wt% of the final resin each) in the TEGDMA resin by mechanical stirring. BisGMA or UDMA was then added to the mix, stirred by hand, and then stirred mechanically at 35 °C for 30 min. The final ratio of BisGMA or UDMA to TEGDMA was 70:30 by weight.
The composites were then blended by coarsely mixing the filler and resin by hand, and then completing the mix with the three roll mill (Exakt 50i TRM, Norderstedt, Germany) until a homogenous material was obtained.
2.4
Mechanical properties
To evaluate the maximum loading of the composites, the dry filler was slowly added to the liquid resin and mixed by hand, before blending with a three roll mill. When the composite was no longer cohesive and broke apart, it was considered to be overloaded, and the value before the last addition was used as the maximum filler loading. This test was done in duplicate. Alternatively, it was also considered maximally loaded when the viscosity of the paste became too high for the machine to mix.
Composite bars were made using split stainless steel bar molds of 2 mm × 2 mm × 25 mm sandwiched between two 0.5 mm thick glass slides. They were polymerized with the lamp mentioned above for 20 s (3 times to cover the whole bar) and then 7 s from the other side (3 times again). The flexural strength and modulus of these specimens were measured in quadruplicate on a universal mechanical analyzer (Instron 5565, USA), according to ISO-4049.
2.5
Optical properties
Composite disks were added into a stainless steel disk molds of 5 mm diameter and 0.5 mm thickness covered by 0.5 mm glass slides, and polymerized for 30 s using a dental lamp. The transmission of light through the disks was measured on a UV/vis spectrometer (Flame USB 2000, LS-1 tungsten halogen lamp, Ocean Optics Inc., USA) by transmission with a custom-machined brass holder for the glass fiber light guides. Each sample was measured three times at random points and then averaged.
The refractive indices of the resins were measured with a handheld digital refractometer (Reichert AR200, NY, USA).
2.6
Polymerization conversion and depth of cure measurements
The extent of monomer polymerization was evaluated by Raman spectroscopy (Renishaw Invia confocal microscope, 785 nm laser, Gloucestershire, UK). For general conversion measurements, 10–20 mg of composite paste was placed on aluminum foil and measured, then exposed to light from the dental lamp for 20 s, and measured again. The extent of polymerization was calculated with the following equations :
C o n v e r s i o n = 1 − C 1638 × U 1608 C 1608 × U 1638
for BisGMA-containing composites with an aromatic C C band at 1608 cm −1 , and
C o n v e r s i o n = 1 − C 1638 × U 1725 C 1725 × U 1638
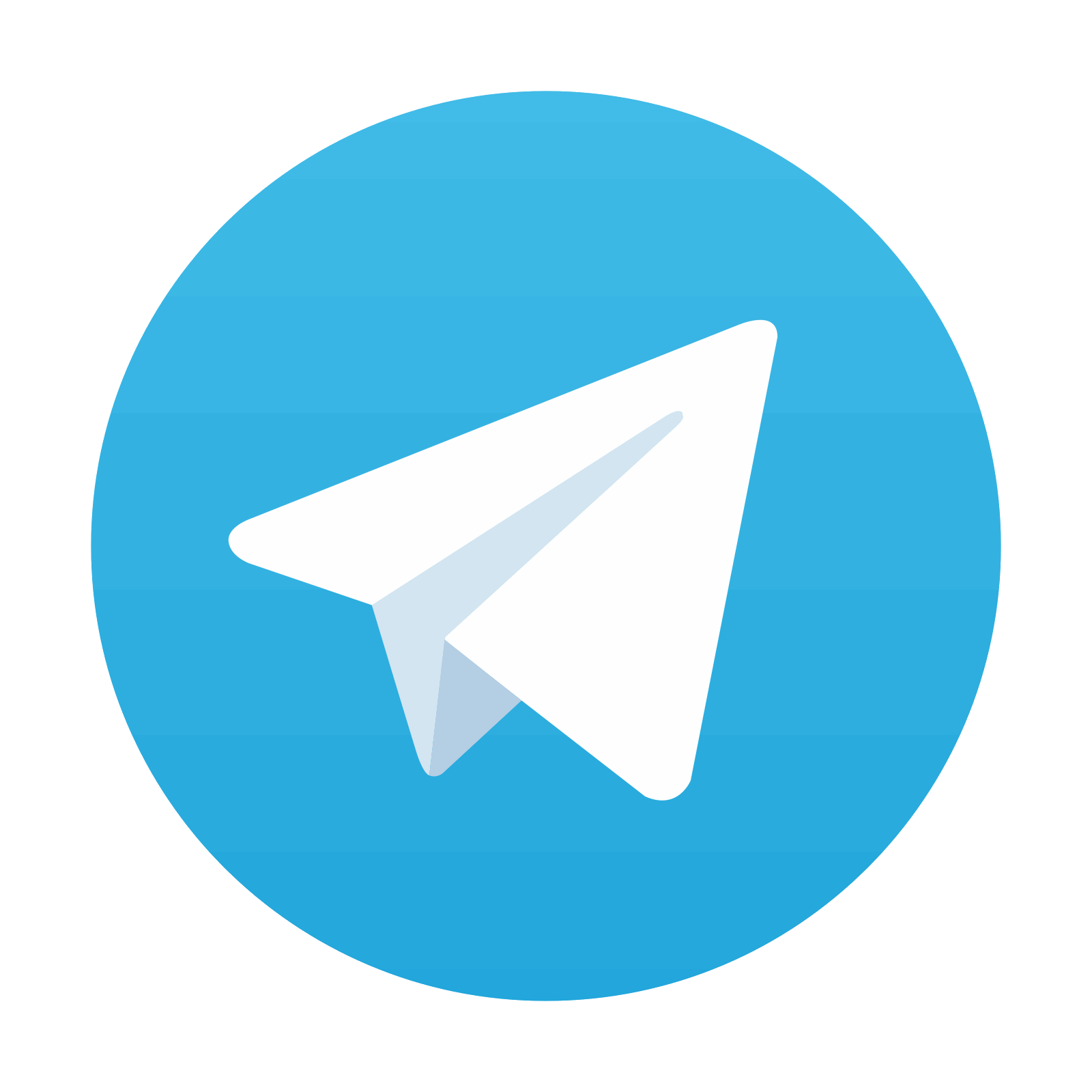
Stay updated, free dental videos. Join our Telegram channel

VIDEdental - Online dental courses
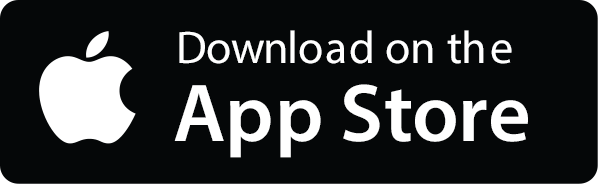
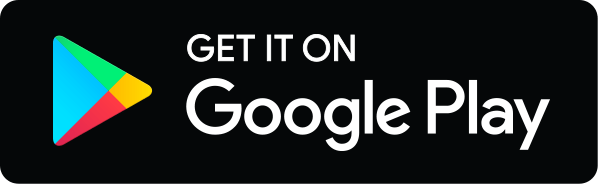